Background
The chronic consumption of androgenic anabolic steroids (AAS) has shown to cause subclinical impairment of myocardial function. Pulsed-wave Doppler tissue imaging (PWDTI) detects early regional alterations of ventricular function, whereas integrated backscatter cyclic variations (IBScv) are tightly related to the contractile efficiency of the left ventricular wall. The aim of this study was to identify the effects of chronic AAS misuse on myocardial function using both PWDTI and IBScv.
Methods
Twenty-eight male bodybuilders (11 AAS users, 17 AAS nonusers) and 20 healthy sedentary subjects (controls), matched according to age, were studied. To assess left ventricular function, each subject underwent standard Doppler echocardiography, PWDTI, and IBScv analyses.
Results
Left ventricular mass was significantly higher in AAS users than in AAS nonusers and controls. Global systolic function (assessed by determining the ejection fraction) was similar in all subjects, but isovolumetric relaxation time was significantly higher in AAS users than in controls. On PWDTI analysis, AAS users showed regional systolic and diastolic dysfunction (evaluated by measuring s′, e′, and a′) not detectable in the other two groups. IBScv identified regional systolic impairment only in AAS users at the level of the left ventricular inferior wall.
Conclusions
The present study confirms that in AAS users, PWDTI and IBScv are effective and reliable noninvasive diagnostic tools for detecting early abnormalities of the systolic and diastolic longitudinal myocardial function, probably related to an increase in myocardial collagen content, interpretable as a repair process against the direct cellular injury produced by AAS.
Androgenic anabolic steroids (AAS) are a major group of doping drugs used by bodybuilders to increase lean body mass and muscle strength. Long-term use of AAS has been associated with functional and toxic multiple-organ alterations, such as cardiovascular diseases, including an increase in left ventricular (LV) mass, hypertension, arrhythmia, myocardial infarction, cerebral stroke, and sudden death. The link between intensive training and AAS use in causing heart hypertrophy is well known. However, the effects of chronic consumption of AAS on cardiovascular structures and function are still a matter of debate. Biopsy data have shown that in athletes under the pharmacologic effects of AAS, a focal increase in myocardial collagen content might occur as a repair mechanism against myocardial damage.
Pulsed-wave Doppler tissue imaging (PWDTI) and strain rate imaging have proven to be valid diagnostic tools for detecting early LV dysfunction in several diseases, such as systemic amyloidosis, myotonic dystrophy, and hypertensive hypertrophy. These techniques have also been used to identify early regional myocardial dysfunction in bodybuilders chronically exposed to AAS. However, they both fail to provide data on the structure of the myocardial wall, such as the localization, distribution, and percentage of connective tissue. Conversely, integrated backscatter cyclic variations (IBScv), which measure the total ultrasonic energy backscattered by the myocardium, offer information on the composition of the examined tissue in addition to its contractility. Indeed, the amount of collagen influences myocardial reflectivity and thus alters the intensity of the backscattered signal.
The aim of our study was to investigate systolic and diastolic cardiac function in both AAS users and drug-free bodybuilders in comparison with healthy sedentary controls by means of an approach combining PWDTI and IBScv.
Methods
Study Population
Power Athletes and Control Group
We selected a population of 28 competitive bodybuilders, including 11 actively taking AAS for ≥2 years (AAS users) and 17 who had never used AAS (AAS nonusers), and 20 age-matched healthy sedentary controls, all men. All subjects gave written informed consent, and the study was approved by the appropriate institutional ethics review committee. Exclusion criteria were coronary artery disease, valvular and congenital heart disease, congestive heart failure, diabetes mellitus, sinus tachycardia, smoking habit, and psychiatric, respiratory, or metabolic disorders.
Training Protocols
All participants had trained intensively for ≥10 h/wk for >5 years. AAS users and nonusers had started bodybuilding at approximately the same age (19.44 ± 2.91 vs 21.47 ± 3.24 years, respectively, P = NS) and completed the same anaerobic isometric static exercises (4.81 ± 1.72 vs 4.5 ± 2.06 h/wk, P = NS), even though the self-reported strength was significantly greater among AAS users (best single repetition for bench press, 144.5 ± 19.49 vs 111.37 ± 21.2 kg, P < .001; Table 1 ).
Variable | AAS users (n = 11) | AAS nonusers (n = 17) |
---|---|---|
Sessions per week | 3.45 ± 0.82 | 3.85 ± 0.89 |
Aerobic exercise (h/wk) | 1.54 ± 0.82 | 3.06 ± 3.10 |
Anaerobic exercise (h/wk) | 4.81 ± 1.72 | 4.50 ± 2.06 |
Years | 8.90 ± 1.80 | 8.06 ± 2.76 |
Starting age | 19.44 ± 2.91 | 21.47 ± 3.24 |
Maximal weight (kg) ∗ | 144.50 ± 19.49 | 111.37 ± 21.20 |
AAS Abuse
An anonymous, self-administered questionnaire was used to investigate each athlete’s clinical (diseases and medication) and drug intake history (type and timing of steroid use and other performance-enhancing drugs). Additionally, urine testing was performed by high-performance liquid chromatography coupled to mass spectrometry to confirm or exclude any recent consumption of anabolic steroids. Each AAS user admitted the current use of multiple AAS administered by intramuscular injection and/or orally. The mean duration of AAS use was 5.73 ± 3 years (range, 3-20 years). The mean weekly dosage of AAS was 1395.5 ± 754 mg.
Sedentary Healthy Controls
We also studied 20 age-matched sedentary controls without detectable cardiovascular risk factors. None of the controls had structural or functional cardiovascular abnormalities or had received any drugs. We ensured comparability of the groups (athletes and controls) by using frequency matching. Baseline characteristics such as family history of atherosclerotic disease, eating habits, and work activities were examined, and for these parameters, no significant differences were observed between the groups of subjects.
Physical Examination and Laboratory Tests
All subjects were examined on an empty stomach. Height, weight, body mass index, body surface area (BSA), body fat mass, heart rate, and blood pressure were measured. Venous blood samples were drawn from each subject, always in the afternoon between 1 and 2 pm , to evaluate serum hormone levels (testosterone, luteinizing hormone, follicle-stimulating hormone, insulin, T3, and T4), hematology (hematocrit, hemoglobin), and blood lipids (total cholesterol, high-density lipoprotein).
Echocardiographic Procedures
Standard Doppler echocardiography, PWDTI, and IBScv were performed using the Sonos 7500 system (Philips Medical Systems, Eindhoven, The Netherlands). The multifrequency phased-array transducer (1.75-3.5 MHz) was used for Doppler echocardiography and Doppler tissue imaging. The Acoustic Densitometry System (Hewlett-Packard, Palo Alto, CA) integrated with the ultrasound system was used to study IBScv. All measurements were analyzed by two experienced readers, using the average of 3 to 5 cardiac cycles.
Standard Doppler Echocardiography
All subjects were at rest and in the left decubitus position. Complete M-mode, two-dimensional Doppler echocardiographic (spectral and color) studies were performed in all subjects to assess cardiac chamber and valve morphology and function, according to the joint recommendations developed by the American Society of Echocardiography and the European Society of Echocardiography. The following parameters were measured: LV end-diastolic and end-systolic diameters, interventricular septal thickness, LV posterior wall thickness, relative diastolic wall thickness, LV mass (calculated according to American Society of Echocardiography criteria and normalized for both BSA and height 2.7 ), LV end-diastolic volume, and LV ejection fraction, calculated from the LV volumes measured using the biplane Simpson method. Pulsed Doppler LV inflow recordings were obtained in the apical 4-chamber view, with a 1-mm to 3-mm sample volume placed between the mitral leaflet tips during diastole. E and A peak velocities, the E/A ratio, and isovolumetric relaxation time (IVRT) (ie, the interval between the end of the systolic output flow and the onset of the transmitral E wave, derived by placing the cursor of continuous-wave Doppler in the LV outflow tract to simultaneously display the end of aortic ejection and the onset of mitral inflow) were measured.
PWDTI
PWDTI relies on the Doppler principle to assess ventricular wall motion velocity. Doppler tissue imaging recordings were performed in 4-chamber, 3-chamber, and 2-chamber views, with a 5-mm sample volume placed in the center of each myocardial segment of interest. PWDTI is characterized by a myocardial systolic wave (S), an early diastolic wave (E), and a late diastolic wave (A). We measured the peak systolic myocardial wall velocity (s′), the early and late diastolic myocardial velocities (e′ and a′), the E/e′ ratio, the e′/a′ ratio, and the IVRT in 12 segments including the basal and mid segments of the lateral wall and posterior septum (4-chamber view), the basal and mid segments of the inferolateral wall and anterior septum (3-chamber view), and the basal and mid segments of the anterior and inferior walls (2-chamber view). The average measurements, based on 3 individual evaluations, were obtained during the end-expiratory phase.
IBScv
Two-dimensional conventional and densitometric images were acquired using the Acoustic Densitometry System, which enables the real-time acquisition of myocardial integrated backscatter. These data were displayed using a logarithmic scale in decibels and with a dynamic range of 44 dB. Acoustic densitometric image loops were acquired in digital format. The IBScv data were analyzed offline in all myocardial segments properly visualized in the long-axis and short-axis views of the left ventricle. The myocardial segments examined in these views were almost perpendicular to the ultrasonic beam, thus minimizing the problems associated with the angle of orientation of the myocardial fibers and the incidence of the ultrasonic beam. Within each segment, an elliptic region of interest was placed within the myocardial thickness of the LV wall, taking care to exclude the endocardial and epicardial specular reflections. Specific software provided a mean value of the integrated backscatter on a logarithmic scale, enabling the calculation of the IBScv between the end of diastole and systole.
Statistical Analysis
Baseline characteristics, blood test results, and echocardiographic data are all expressed as mean ± SD. Analysis of variance with Bonferroni’s post hoc test for multiple comparisons and the t test for unpaired data were used to estimate differences between groups. Proportions were compared using the χ 2 test or Fisher’s exact test. Relationships between IBScv, peak systolic myocardial wall velocity (s′), and age, myocardial mass, LV end-diastolic volume, and AAS use were evaluated using the simple linear correlation coefficient r (determination coefficient r 2 ). Multivariate linear regression analysis with a forward selection procedure was used to identify the parameters independently associated with regional systolic and diastolic function impairment on PWDTI and IBScv. A P value < .05 was considered significant for statistical purposes. SPSS (SPSS, Inc, Chicago, IL) was used for calculations.
Results
Clinical Characteristics of the Study Population
Anthropometric characteristics and blood test results are shown in Table 2 . No differences between groups emerged in height, blood pressure, or heart rate. AAS users weighed more and had higher body mass indexes and BSAs compared with AAS nonusers and sedentary controls. Observing the systolic and diastolic pressure values, it appeared that some bodybuilders (both AAS users and AAS nonusers) may have been affected by hypertension. However, our data include only blood pressure values measured in a single visit, thus not allowing a diagnosis of hypertension. As for blood tests, high-density lipoprotein cholesterol was significantly lower in AAS users than in nonusers and controls. The other parameters did not differ significantly among the 3 groups.
Variable | AAS users (n = 11) | AAS nonusers (n = 17) | Sedentary men (n = 20) |
---|---|---|---|
Age (y) | 31.27 ± 4.40 | 29.75 ± 2.95 | 28.30 ± 3.26 |
Height (cm) | 179.81 ± 7.19 | 179.50 ± 6.92 | 176.60 ± 5.73 |
Weight (kg) ∗†‡ | 94.90 ± 11.07 | 82.31 ± 10.33 | 72.90 ± 6.19 |
Body mass index (kg/m 2 ) ∗†‡ | 29.27 ± 2.24 | 25.47 ± 2.74 | 23.46 ± 1.33 |
BSA (m 2 ) ∗†‡ | 2.14 ± 0.15 | 2.01 ± 0.14 | 1.89 ± 0.09 |
Heart rate | 70.72 ± 8.25 | 62.05 ± 9.05 | 69.00 ± 12.49 |
Systolic blood pressure (mm Hg) | 134.50 ± 15.72 | 129.33 ± 15.79 | 122.22 ± 7.94 |
Diastolic blood pressure (mm Hg) | 84.54 ± 9.34 | 84.33 ± 10.15 | 82.50 ± 6.34 |
Hematocrit | 0.45 ± 0.04 | 0.45 ± 0.14 | 0.45 ± 0.02 |
Hemoglobin (g/dL) | 15.12 ± 1.42 | 15.20 ± 1.48 | 15.58 ± 1.04 |
Total cholesterol (mmol/L) | 4.31 ± 0.90 | 4.66 ± 1.16 | 4.73 ± 0.73 |
High-density lipoprotein (mmol/L) ∗† | 0.74 ± 0.47 | 1.45 ± 0.30 | 1.47 ± 0.20 |
∗ AAS users versus AAS nonusers, P < .05
† AAS users versus sedentary men, P < .05
Standard Doppler Echocardiographic Data
Standard echocardiographic and color Doppler results are listed in Table 3 . LV end-diastolic volume, interventricular septal thickness, LV posterior wall thickness, LV mass (normalized to BSA and height 2.7 ) and relative diastolic wall thickness were significantly greater in AAS users than in nonusers and sedentary controls ( P < .05).
Variable | AAS users (n = 11) | AAS nonusers (n = 17) | Sedentary men (n = 20) |
---|---|---|---|
LV end-diastolic diameter (mm) | 51.00 ± 5.30 | 50.00 ± 4.90 | 48.60 ± 2.22 |
LV end-diastolic volume (mm) ∗† | 71.09 ± 12.20 | 61.06 ± 6.31 | 59.10 ± 5.87 |
LV end-systolic volume (mm) | 56.63 ± 14.90 | 56.71 ± 13.20 | 48.22 ± 10.69 |
Interventricular septal thickness (mm) ∗† | 12.27 ± 0.90 | 10.00 ± 1.17 | 9.00 ± 0.81 |
LV posterior wall thickness (mm) ∗† | 11.45 ± 1.30 | 9.70 ± 1.00 | 9.00 ± 0.81 |
LV mass/BSA (g/m 2 ) ∗† | 96.72 ± 24.96 | 88.15 ± 26.48 | 60.30 ± 13.78 |
LV mass/height 2.7 (g/m 2.7 ) ∗† | 42.29 ± 11.87 | 27.71 ± 7.06 | 24.46 ± 5.77 |
Relative diastolic wall thickness ∗† | 0.47 ± 0.07 | 0.40 ± 0.04 | 0.37 ± 0.03 |
Ejection fraction (%) | 63.45 ± 4.56 | 61.12 ± 4.35 | 62.30 ± 4.80 |
Peak E velocity (cm/s) | 76.08 ± 11.92 | 83.00 ± 13.47 | 85.74 ± 12.88 |
Peak A velocity (cm/s) | 50.84 ± 10.72 | 56.63 ± 8.75 | 53.22 ± 11.16 |
Peak E/A ratio | 1.57 ± 0.52 | 1.48 ± 0.22 | 1.66 ± 0.39 |
Isovolumetric relaxation time (ms) † | 77.67 ± 16.57 | 71.50 ± 11.21 | 60.78 ± 11.60 |
∗ AAS users versus AAS nonusers, P < .05
The prevalence of cut LV hypertrophy was 1 in 11 for AAS users, 0 in 17 for AAS nonusers, and 0 in 20 for controls. The normality of both the E/A and E/e′ ratios suggested a physiologic nature of LV hypertrophy. Moreover, concentric remodeling was found in 8 of 11 AAS users (72%) and in 4 of 17 AAS nonusers (24%); none of the control group exhibited concentric geometry (relative wall thickness > 0.42).
PWDTI Data
PWDTI findings are shown in Table 4 . Peak s′ was significantly lower in AAS users than in sedentary controls in the basal segment of the lateral wall as well as in the basal and mid segments of the anterior wall. Impaired peak s′ in ≥1 segment of the left ventricle was more frequently seen among AAS users (7 of 11 [73%]) than in either AAS nonusers (3 of 17 [18%], P < .05) or sedentary controls (2 of 20 [10%], P < .05). The regional systolic function impairment assessed by PWDTI was correlated with LV mass indexed to BSA ( r = 0.51, P < .001), LV end-diastolic volume ( r = 0.34, P < .05), and AAS use ( r = 0.52, P < .001). Multivariate regression analysis ( Table 5 ) identified AAS use as an independent factor correlated with regional systolic function (odds ratio, 10.6; 95% confidence interval, 2.04-55.5; P = .005). Peak e′ was significantly lower in AAS users than in nonusers at the level of both the basal and mid segments of the inferior wall. E/e′ ratios were significantly higher in AAS users than in nonusers and controls at the level of the basal inferior wall and mid posterior septum. The e′/a′ ratios were significantly lower in AAS users than in nonusers at the mid segment of the lateral wall ( Figure 1 A and B). Inverse e′/a′ ratios (impaired diastolic function) were detected in 6 of 11 AAS users, in only 2 of 17 nonusers ( P < .05), and in none of the sedentary controls ( P < .05). IVRTs were significantly longer in AAS users than in nonusers or sedentary controls, measured at the level of both the mid segment of the lateral wall and the basal segment of the anterior septum. Regional diastolic function impairment was correlated with age ( r = 0.47, P = .003), BSA ( r = 0.40, P = .003), LV mass ( r = .45, P < .005), and AAS use ( r = .52, P < .001). Multivariate linear regression analysis showed that AAS use was an independent predictor of regional diastolic dysfunction (odds ratio, 30; 95% confidence interval, 2.9-306; P = .005).
Variable | AAS users (n = 11) | AAS nonusers (n = 17) | Sedentary men (n = 20) |
---|---|---|---|
Basal lateral wall (4-chamber view) | |||
Peak s′ (cm/s) † | 8.49 ± 2.57 | 9.81 ± 2.38 | 11.18 ± 1.93 |
Peak e′ (cm/s) | 13.57 ± 3.19 | 15.99 ± 3.10 | 15.31 ± 1.85 |
E/e′ | 5.77 ± 1.31 | 5.31 ± 0.67 | 5.64 ± 0.93 |
e′/a′ | 1.99 ± 0.78 | 2.66 ± 0.78 | 2.58 ± 0.96 |
Mid lateral wall (4-chamber view) | |||
Peak s′ (cm/s) | 6.69 ± 2.69 | 7.90 ± 2.73 | 10.38 ± 3.24 |
Peak e′ (cm/s) † | 11.65 ± 2.99 | 11.86 ± 1.41 | 12.34 ± 1.71 |
E/e′ | 6.61 ± 1.26 | 7.11 ± 1.34 | 7.28 ± 1.38 |
e′/a′ ∗‡ | 1.88 ± 0.66 | 2.52 ± 0.59 | 1.98 ± 0.34 |
Mid posterior septum (4-chamber view) | |||
Peak s′ (cm/s) | 6.32 ± 1.10 | 6.58 ± 0.78 | 6.05 ± 1.09 |
Peak e′ (cm/s) | 10.41 ± 2.65 | 9.78 ± 2.13 | 10.37 ± 1.60 |
E/e′ ∗ | 7.61 ± 2.04 | 5.74 ± 0.92 | 6.23 ± 1.91 |
e′/a′ †‡ | 1.69 ± 0.59 | 1.57 ± 0.29 | 1.91 ± 0.49 |
Mid inferolateral wall (3-chamber view) | |||
Peak s′ (cm/s) † | 6.22 ± 2.01 | 8.12 ± 2.81 | 9.33 ± 2.88 |
Peak e′ (cm/s) ∗ | 10.42 ± 2.25 | 13.01 ± 2.65 | 12.29 ± 2.16 |
E/e′ | 7.57 ± 1.33 | 6.56 ± 1.19 | 7.10 ± 1.05 |
e′/a′ | 1.57 ± 0.60 | 2.31 ± 0.88 | 2.10 ± 0.57 |
Basal anterior wall (2-chamber view) | |||
Peak s′ (cm/s) † | 7.05 ± 1.98 | 8.71 ± 2.76 | 10.33 ± 2.57 |
Peak e′ (cm/s) | 10.93 ± 2.74 | 12.26 ± 2.58 | 11.90 ± 4.00 |
E/e′ †‡ | 6.74 ± 1.58 | 6.88 ± 1.59 | 8.38 ± 1.58 |
e′/a′ | 1.77 ± 0.71 | 68.23 ± 14.40 | 1.73 ± 0.45 |
Mid anterior wall (2-chamber view) | |||
Peak s′ (cm/s) † | 5.59 ± 1.29 | 6.53 ± 2.09 | 7.80 ± 1.62 |
Peak e′ (cm/s) | 8.69 ± 2.45 | 9.14 ± 2.34 | 9.41 ± 0.69 |
E/e′ | 9.17 ± 2.48 | 9.42 ± 2.64 | 8.87 ± 1.64 |
e′/a′ | 1.55 ± 0.44 | 1.87 ± 0.46 | 1.94 ± 0.59 |
Basal inferior wall (2-chamber view) | |||
Peak s′ (cm/s) | 8.73 ± 1.28 | 9.59 ± 1.20 | 9.42 ± 1.66 |
Peak e′ (cm/s) ∗ | 11.90 ± 2.87 | 15.50 ± 3.52 | 14.97 ± 2.68 |
E/e′ ∗† | 7.60 ± 2.26 | 5.74 ± 1.50 | 5.96 ± 1.01 |
e′/a′ † | 1.52 ± 0.52 | 2.11 ± 0.67 | 2,35 ± 0.97 |
Mid inferior wall (2-chamber view) | |||
Peak s′ (cm/s) | 6.79 ± 1.19 | 7.37 ± 1.05 | 7.03 ± 1.31 |
Peak e′ (cm/s) ∗ | 9.22 ± 1.99 | 11.66 ± 2.92 | 10.54 ± 1.61 |
E/e′ | 8.61 ± 1.65 | 7.50 ± 1.75 | 8.01 ± 1.31 |
e′/a′ | 1.41 ± 0.61 | 1.88 ± 0.59 | 1.82 ± 0.51 |
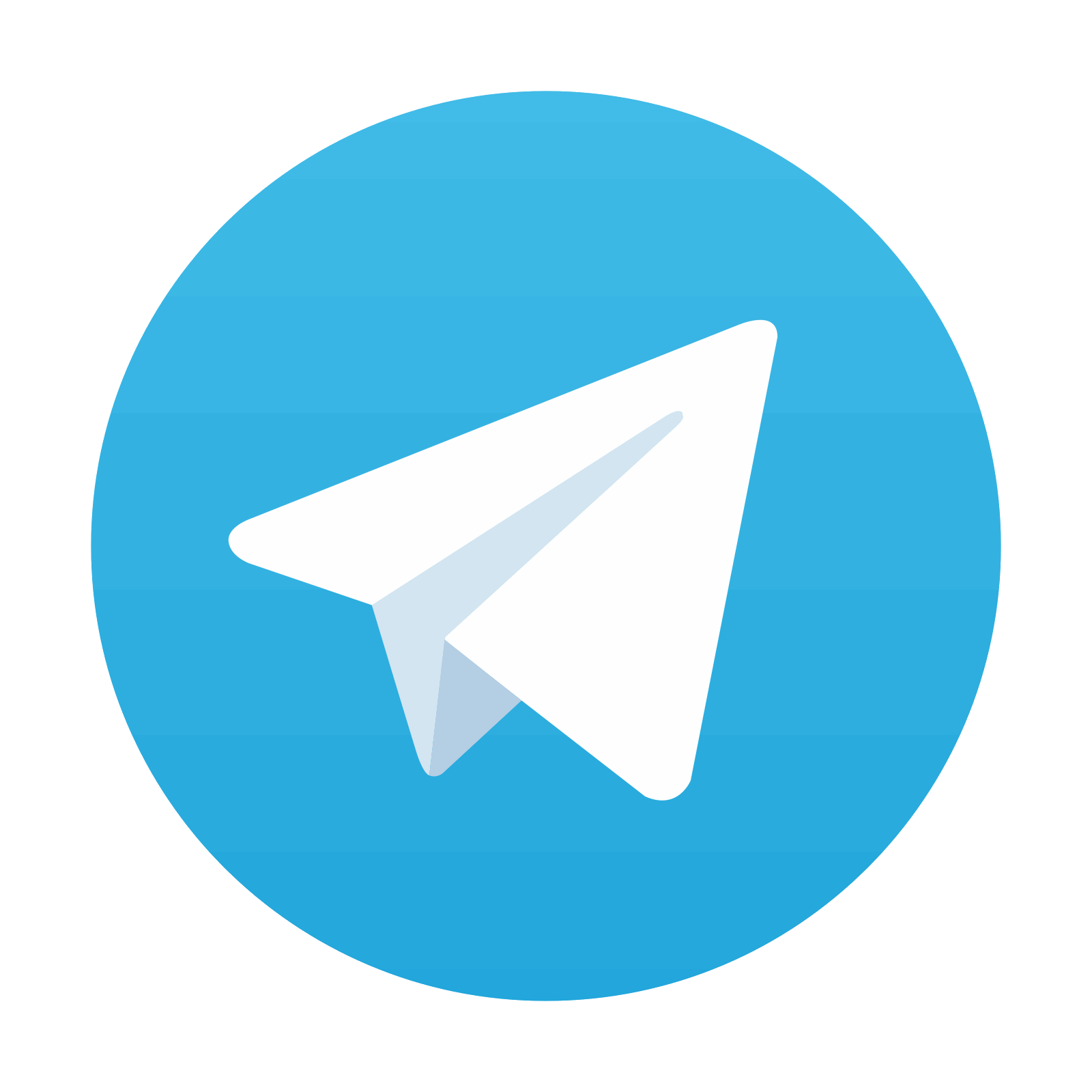
Stay updated, free articles. Join our Telegram channel

Full access? Get Clinical Tree
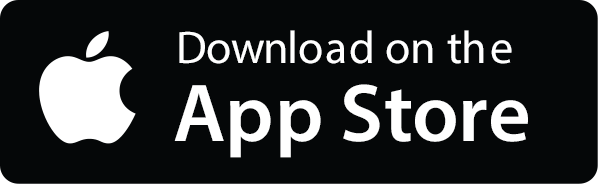
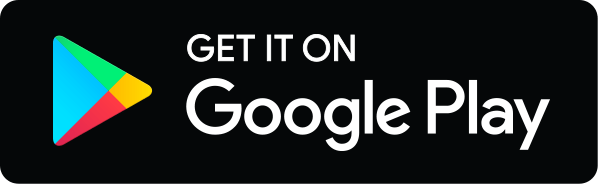
