Background
Systemic hypertension and type 2 diabetes mellitus are associated with impaired left atrial (LA) function, but whether LA functional abnormalities also occur in patients with hypertension and diabetes who have normal LA sizes is unknown. The aim of this study was to explore LA strain using speckle-tracking echocardiography in patients with hypertension or diabetes and normal LA size.
Methods
LA strain was studied by speckle-tracking echocardiography in 155 patients with hypertension or diabetes with LA volume indexes < 28 mL/m 2 (83 with hypertension, 34 with diabetes, and 38 with both diabetes and hypertension) and 36 age-matched controls. The following indexes were measured: peak atrial longitudinal strain, time to peak atrial longitudinal strain, atrial longitudinal strain during early diastole and late diastole, and peak LA strain rate during ventricular systole, early diastole, and late diastole.
Results
Peak atrial longitudinal strain was lower in patients with hypertension (29.0 ± 6.5%) and those with diabetes (24.7 ± 6.4%) than in controls (39.6 ± 7.8%) and further reduced in patients with diabetes and hypertension (18.3 ± 5.0%) ( P < .0001). Similar results were found for atrial longitudinal strain during early diastole, atrial longitudinal strain during late diastole, and peak LA strain rate during ventricular systole and early diastole ( P < .0001 for all). An inverse trend was found for time to peak atrial longitudinal strain, whereas no differences in peak LA strain rate during late diastole were observed. Two-way analysis of variance showed no interactions between hypertension and diabetes. In multivariate analyses, hypertension and diabetes were both independently associated with decreases in all LA strain and strain rate indexes, with the exception of peak LA strain rate during late diastole.
Conclusions
LA deformation mechanics are impaired in patients with hypertension or diabetes with normal LA size. The coexistence of both conditions further impairs LA performance in an additive fashion. Speckle-tracking echocardiography may be considered a promising tool for the early detection of LA strain abnormalities in these patients.
Diabetes mellitus and arterial hypertension are both associated with morphologic and functional left atrial (LA) abnormalities. An increase in LA size in patients with diabetes or hypertension is a common finding in clinical practice, and the mechanisms underlying this enlargement have been extensively analyzed. An impact of both conditions on LA function has been shown as well, using methods based on two-dimensional echocardiographic measurements of LA volumes, standard Doppler imaging of transmitral and pulmonary vein flow, and Doppler tissue imaging of LA myocardial wall motion. However, these approaches are limited by a number of shortcomings, including the need for geometric assumptions, suboptimal reproducibility, and technical limitations common to all Doppler-based techniques. Moreover, most of these studies included patients regardless of LA size. This raises the question of whether LA dysfunction in patients with hypertension and diabetes may be detected in the absence of LA enlargement. This question may be of clinical interest, because LA size is often used as a surrogate marker of LA function in clinical practice.
Speckle-tracking echocardiography (STE) allows direct and angle-independent analysis of myocardial deformation, thus providing sensitive and reproducible indexes of myocardial fiber dysfunction that overcome most of the limitations of Doppler-derived strain measures. The feasibility and reproducibility of STE for the study of LA mechanics have been recently validated. Abnormalities in LA strain have been shown by STE in some pathophysiologic conditions, including dilated cardiomyopathy, left ventricular (LV) hypertrophy, paroxysmal atrial fibrillation, hypertrophic cardiomyopathy, congenital heart diseases, and prolonged strenuous exercise. However, no data exist regarding patients with diabetes or hypertension. The assessment of LA strain dynamics by STE in these patients may be of particular interest in those with no evidence of LA enlargement, because it may provide additional information for the early detection of LA abnormalities.
The aim of this study was to explore LA function using STE in patients with hypertension, diabetes, or both, and with echocardiographic evidence of normal LA size.
Methods
Study Population
Asymptomatic patients affected by arterial hypertension and/or type 2 diabetes mellitus, consecutively enrolled in our laboratory of echocardiography between June 2009 and September 2010, were considered for this study. For inclusion in the study population, patients needed to have echocardiographic evidence of normal LA size, defined as LA volume indexed to body surface area < 28 mL/m 2 , and no symptoms of suspected cardiac origin. According to current European Society of Hypertension and European Society of Cardiology guidelines, hypertension was defined as systolic blood pressure ≥ 140 mm Hg and/or diastolic blood pressure ≥ 90 mm Hg on three or more occasions or as antihypertensive treatment in the presence of a documented history of hypertension. Diabetes mellitus was diagnosed in accordance with the World Health Organization criteria as fasting glucose ≥ 126 mg/dL or the use of hypoglycemic medications in subjects with documented histories of diabetes. Exclusion criteria were overt coronary artery disease (defined by at least one of the following: history of effort angina, acute coronary syndromes, or revascularization procedures; evidence of positive exercise stress test results; and segmental wall abnormalities at echocardiography); history of atrial fibrillation, atrial flutter, or other major arrhythmias; mitral regurgitation of higher degree than trivial; aortic regurgitation; any degree of valve stenosis; previous valve surgery; hypertrophic cardiomyopathy; left bundle branch block; pacemaker implantation; heart transplantation; malignant hypertension; uncontrolled diabetes, defined as glycosylated hemoglobin ≥ 7.0% ; severe renal failure, defined as estimated creatinine clearance < 30 mL/min; history of chronic obstructive pulmonary disease, abnormal thyroid function, or other significant systemic chronic disease; inadequate acoustic windows; and refusal to participate in the study. A total of 164 patients met the selection criteria during the period of enrollment. Within the study population, 87 subjects had hypertension but not diabetes, 37 had diabetes but normal blood pressure, and 40 had diabetes and hypertension. In the hypertension group, grade I, II, and III hypertension was present in 57, 26, and four patients, respectively, and 47 subjects (54%) had evidence of LV hypertrophy, as identified using sex-specific cutoff values recommended by the American Society of Echocardiography’s guidelines for chamber quantification. Among patients with diabetes and hypertension, grades I, II, and III hypertension was present in 23, 12, and five patients, respectively, and 27 subjects (68%) had evidence of LV hypertrophy.
The control group was selected among a total of 76 healthy individuals with no histories of any cardiovascular or systemic disease and with normal findings on clinical examination, electrocardiography, and echocardiography who visited our laboratory during the period of enrollment. Matching for age was performed using a 1:4 scheme and to within ±5 years. The procedure was performed by obtaining randomly arranged subsets of four noncontrol subjects, calculating mean age in each subset, and then matching one control subject to this age by considering the age criterion. Thus, 41 age-matched healthy subjects entered the control group. Main reasons for evaluation for these subjects were preoperative assessment of risk in subjects undergoing minor noncardiovascular surgery ( n = 22), atypical chest discomfort with negative maximal exercise stress test results ( n = 8), preparticipation sports examination for noncompetitive physical activities ( n = 7), and palpitations with no significant abnormalities on Holter electrocardiographic recording ( n = 4). All subjects provided informed consent for participation in the study, and all procedures were conducted in accordance with the Declaration of Helsinki.
Standard Echocardiography
Studies were performed using a high-quality echocardiograph (Vivid 7; GE Medical Systems, Milwaukee, WI) equipped with a 1.5-MHz and 3.6-MHz transducer. Standard LV measurements were measured from the long-axis parasternal view in accordance with current American Society of Echocardiography recommendations. LV and LA volumes were calculated from apical four-chamber and two-chamber views using the biplane modified Simpson’s rule. Maximal, precontraction, and minimal LA volumes were measured just before mitral valve opening, at the beginning of the P wave, and at mitral valve closure, respectively. LV inflow was assessed using pulsed Doppler by placing the sample volume at the level of leaflet tips. LV long-axis function was explored using pulsed tissue Doppler from the apical four-chamber view, by placing the sample volume at the junction of the LV wall with the septal and lateral mitral annulus. Peak systolic (s′), early diastolic (e′), and late diastolic (a′) annular velocities were obtained by averaging values recorded at the septal and lateral positions. The E/e′ ratio was used as an index of LV filling pressures.
Standard Assessment of LA Function
The following indexes of LA function were calculated. Total LA stroke volume (LASV) was obtained as the difference between maximal and minimal LA volumes. Passive LASV was calculated as the difference between maximal and precontraction LA volumes, whereas active LASV was calculated as the difference between precontraction and minimal LA volumes. Total and passive emptying fractions were calculated as the ratio of total LASV and passive LASV to maximal LA volume × 100, respectively. Active emptying fraction was calculated as the ratio of active LASV to precontraction LA volume × 100. LA expansion index was obtained as the ratio of total LASV to minimum LA volume × 100.
STE
Speckle-tracking analyses were performed using commercially available software (EchoPAC; GE Medical Systems). Apical four-chamber and two-chamber images were recorded using conventional two-dimensional grayscale imaging, during breath hold with a stable electrocardiographic recording. Care was taken to optimize visualization of the LA cavity and to maximize LA area in apical views, avoiding foreshortening of the left atrium. The frame rate was set in all patients between 60 and 80 frames/sec. Offline analyses were performed as previously described. Briefly, the LA endocardial border was manually traced at end-systole using a point-and-click approach in both apical views. An epicardial surface tracing was automatically generated by the software, delineating a region of interest including six segments. The region of interest was manually adjusted to encompass the thickness of the LA myocardium, and an automated segmental tracking quality analysis was obtained. Further manual adjustment of the region of interest was performed in the case of segments with inadequate tracking. Cine loop preview was also used to check that the inner border of the region of interest followed the LA endocardial border throughout the cardiac cycle. Segments in which adequate tracking quality could not be obtained despite manual adjustment were excluded from the analysis, and patients in whom no adequate tracking quality was obtained in more than three segments were excluded from the study. Longitudinal strain and strain rate curves were then generated for each atrial segment. Setting zero strain at LV end-diastole, the LA strain pattern is characterized by a predominant positive wave that peaks at the end of ventricular systole, followed by two distinct descending phases in early diastole and late diastole. The LA strain rate pattern is characterized by a positive wave occurring during ventricular systole and two negative waves during early diastole and late diastole. The systolic component of LA strain and strain rate mostly reflects LA reservoir function, whereas the early diastolic and late diastolic components mostly reflect LA conduit function and LA contractile function, respectively. The following indexes were measured from average LA strain curves ( Figure 1 , top ): (1) peak atrial longitudinal strain (PALS); (2) time to PALS, measured from the onset of the QRS complex to the positive peak of strain curve and expressed as a percentage of the RR interval duration; (3) atrial longitudinal strain during late diastole (ALS late ), defined as strain at the onset of the P wave; and (4) atrial longitudinal strain during early diastole (ALS early ), defined as the difference between PALS and ALS late . The following indexes were measured from LA strain rate curves ( Figure 1 , bottom ): (1) PALS rate during ventricular systole (ALSR syst ), (2) PALS rate during early diastole (ALSR early ), and (3) peak atrial longitudinal strain rate during late diastole (ALSR late ). All indexes were obtained by averaging values obtained in the apical four-chamber and two-chamber views.

Data Collection and Reproducibility
LA strain analyses were performed offline by two experienced and independent investigators (M.C. and M.L.C.) who were blinded to clinical and other echocardiographic characteristics of patients. All indexes were obtained by averaging measurements obtained in three consecutive cardiac cycles. Adequate reproducibility and feasibility of speckle tracking for the assessment of LA longitudinal strain in our laboratory have been recently reported, with variability coefficients <6% for all measurements in both interobserver and intraobserver analyses. For the purpose of this study, we also assessed variability in LA volumes in a randomly selected subset of 30 patients. Interobserver and intraobserver variability coefficients were as follows: maximal LA volume, 5.8% and 4.2%; precontraction LA volume, 6.3% and 5.0%; and minimal LA volume, 6.1% and 4.9%.
Statistical Analysis
All data are expressed as mean ± SD. Between-group comparisons of continuous variables were performed using analysis of variance (ANOVA), followed by the Scheffé post hoc pairwise comparison test. Categorical variables were compared using χ 2 tests or Fisher’s exact tests as appropriate. General linear modeling analysis, considering hypertension and diabetes as main factors within a 2 × 2 factorial design, was used to explore the interaction effect between the two conditions on LA strain and strain rate indexes. Univariate correlations were determined using Pearson’s coefficient for continuous variables and the point-biserial coefficient for dichotomous variables. Multiple regression was performed to explore the associations of hypertension and diabetes with indexes of LA function after adjustment to confounders. A first multivariate model was built by adjusting for age, gender, and body size. A second multivariate model was built by adjusting to heart rate, systolic blood pressure, ejection fraction, indexed LV mass, relative wall thickness, s′, e′, and E/e′ ratio. For negative LA strain rate variables (i.e., ALSR early and ALSR late ), the absolute value (i.e., the positive value obtained after ignoring the negative sign) was considered in correlation and regression analyses and in the figures. The significance level was set at .05. All statistical tests were two-tailed. SPSS Windows release 12.0.0 (SPSS, Inc., Chicago, IL) was used to perform the analyses. The age-matching procedure was performed using SAS version 9.1 (SAS Institute Inc., Cary, NC).
The sample size for this study was calculated by hypothesizing a common standard deviation within a group of 7% for PALS, on the basis of previously published data. Because the study was planned to enroll patients consecutively, to take into account the expected differences in the prevalence of hypertension and diabetes, we assumed an expected 2:1 ratio between the size of the group with isolated hypertension and that of the other study groups. Under these assumptions, a total sample of 140 subjects (i.e., 28 controls, 56 patients with hypertension, 28 patients with diabetes, and 28 patients with diabetes and hypertension) would have achieved 80% power to detect a “standard deviation among the means” of 2% (i.e., a standard deviation among four hypothesized means, one for each group, under the alternative hypothesis) in a one-way ANOVA design at a .05 significance level. This corresponded to an effect size (Cohen’s f ) of 2/7 = 0.3. Power calculations were performed using Power Analysis and Sample Size 2008 version 08.0.6 (NCSS, Kaysville, UT).
Results
General Characteristics
In the overall study population, 14 subjects (nine patients and five controls) were excluded during offline strain analysis because of the impossibility of obtaining adequate tracking quality in more than three LA segments. Among a total of 2,292 segments analyzed in the remaining 155 patients (patients with hypertension but not diabetes, n = 83; patients with diabetes and normal blood pressure, n = 34; patients with diabetes and hypertension, n = 38) and 36 controls, adequate tracking was possible in 2,180 segments (95.1%). The average postprocessing time was 2.2 ± 0.9 min. Main clinical and echocardiographic characteristics of the study groups are shown in Tables 1 and 2 . Expected between-group differences were found in body size, blood pressure, LV mass and geometry, LV longitudinal systolic function, and diastolic function. There were no differences in LA volumes and standard indexes of LA function.
Variable | Controls | HTN group | DM group | HTN and DM group | P |
---|---|---|---|---|---|
Age (y) | 62.3 ± 12.7 | 65.6 ± 11.0 | 64.0 ± 12.3 | 64.7 ± 11.5 | .64 |
Women | 19 (52.8%) | 40 (48.2%) | 14 (41.2%) | 16 (42.1%) | .72 |
Body surface area (m 2 ) | 1.7 ± 0.2 | 1.9 ± 0.3 ∗ | 1.9 ± 0.3 ∗ | 1.9 ± 0.2 ∗ | .017 |
Body mass index (kg/m 2 ) | 23.8 ± 2.4 | 28.3 ± 5.3 ∗ | 27.4 ± 4.2 ∗ | 28.7 ± 4.4 ∗ | <.0001 |
SBP (mm Hg) | 127.3 ± 11.4 | 143.3 ± 14.5 ∗ | 128.0 ± 12.1 | 145.5 ± 13.3 ∗ | <.0001 |
DBP (mm Hg) | 79.3 ± 6.5 | 88.0 ± 7.5 ∗ | 80.3 ± 7.9 | 89.2 ± 6.2 ∗ | <.0001 |
Heart rate (beats/min) | 75.6 ± 13.7 | 74.1 ± 13.0 | 73.3 ± 10.9 | 75.4 ± 13.4 | .85 |
Cigarette smoking | 5 (13.9%) | 15 (18.1%) | 6 (17.6%) | 7 (18.4%) | .95 |
Hypercholesterolemia | — | 22 (26.5%) | 10 (29.4%) | 17 (44.7%) | .13 |
Hypertriglyceridemia | — | 13 (14.5%) | 8 (23.5%) | 13 (34.2%) | .071 |
Medications | |||||
ACE inhibitors | — | 37 (44.0%) | — | 21 (55.3%) | .37 |
Angiotensin II receptor blockers | — | 19 (23.9%) | — | 9 (26.5%) | .89 |
β-blockers | — | 11 (13.0%) | — | 5 (13.2%) | .78 |
Calcium antagonists | — | 16 (19.0%) | — | 10 (24.3%) | .52 |
Statins | — | 19 (22.9%) | 10 (29.4%) | 15 (39.5%) | .17 |
Oral antidiabetic medications | — | — | 30 (88.2%) | 33 (86.8%) | .86 |
Insulin | — | — | 8 (23.5%) | 9 (21.9%) | .79 |
Variable | Controls | HTN group | DM group | HTN and DM group | P |
---|---|---|---|---|---|
LVEDV (mL) | 83.3 ± 19.0 | 94.3 ± 34.9 | 92.0 ± 40.8 | 93.3 ± 29.0 | .42 |
Indexed LVEDV (mL/m 2 ) | 46.6 ± 11.4 | 51.8 ± 19.6 | 51.8 ± 16.1 | 49.5 ± 13.9 | .51 |
LVESV (mL) | 36.8 ± 15.0 | 40.2 ± 17.9 | 40.0 ± 19.8 | 40.4 ± 14.5 | .80 |
Indexed LVESV (mL/m 2 ) | 20.5 ± 7.9 | 22.2 ± 10.8 | 22.9 ± 9.9 | 21.4 ± 7.2 | .75 |
LV mass (g/m) | 139.3 ± 42.0 | 204.9 ± 52.0 ∗ | 181.1 ± 38.2 ∗ | 232.4 ± 66.2 ∗ ‡ | <.0001 |
Indexed LV mass (g/m 2 ) | 82.4 ± 28.7 | 109.4 ± 27.9 ∗ | 100.1 ± 22.6 ∗ | 124.2 ± 34.4 ∗ ‡ | <.0001 |
Relative wall thickness | 0.40 ± 0.07 | 0.48 ± 0.08 ∗ | 0.43 ± 0.07 † | 0.51 ± 0.11 ∗ | <.0001 |
LV ejection fraction (%) | 59.1 ± 7.3 | 57.5 ± 5.7 | 58.0 ± 5.8 | 56.9 ± 5.9 | .47 |
Mitral E/A ratio | 1.20 ± 0.42 | 0.78 ± 0.31 ∗ | 0.88 ± 0.26 ∗ | 0.80 ± 0.34 ∗ | <.0001 |
Sm (cm/sec) | 9.3 ± 2.3 | 8.2 ± 2.0 ∗ | 8.0 ± 2.4 ∗ | 8.1 ± 2.3 ∗ | .036 |
Em (cm/sec) | 12.6 ± 4.9 | 9.3 ± 3.1 ∗ | 9.6 ± 3.0 ∗ | 8.7 ± 2.3 ∗ ‡ | <.0001 |
Am (cm/sec) | 11.2 ± 3.6 | 12.7 ± 3.2 ∗ | 12.2 ± 3.2 ∗ | 12.6 ± 3.9 | .21 |
E/Em ratio | 6.9 ± 4.3 | 7.2 ± 3.5 | 7.6 ± 2.5 | 8.1 ± 2.5 | .46 |
LA volumes | |||||
Maximal LAVi (mL/m 2 ) | 21.9 ± 4.0 | 22.0 ± 4.3 | 22.1 ± 4.3 | 22.9 ± 4.0 | .58 |
Precontraction LAVi (mL/m 2 ) | 13.2 ± 4.2 | 14.0 ± 4.5 | 14.5 ± 3.7 | 14.5 ± 3.3 | .55 |
Minimal LAVi (mL/m 2 ) | 8.5 ± 3.3 | 8.7 ± 3.0 | 9.2 ± 2.7 | 8.7 ± 3.0 | .75 |
LA function | |||||
Total LASV (mL) | 24.9 ± 4.2 | 25.2 ± 8.5 | 23.9 ± 8.4 | 27.2 ± 6.0 | .28 |
Passive LASV (mL) | 15.9 ± 4.2 | 15.3 ± 7.9 | 14.4 ± 6.4 | 16.3 ± 4.8 | .62 |
Active LASV (mL) | 9.1 ± 4.0 | 10.0 ± 6.5 | 9.6 ± 4.4 | 11.0 ± 5.2 | .50 |
Total LAEF (%) | 62.3 ± 9.6 | 60.6 ± 10.0 | 58.2 ± 9.5 | 62.6 ± 10.0 | .22 |
Passive LAEF (%) | 40.3 ± 11.0 | 36.7 ± 14.9 | 34.6 ± 11.4 | 37.2 ± 9.1 | .30 |
Active LAEF (%) | 37.0 ± 11.3 | 35.6 ± 16.7 | 35.8 ± 11.2 | 39.6 ± 16.0 | .57 |
LA expansion index (%) | 183.5 ± 76.7 | 169.5 ± 65.7 | 153.6 ± 68.1 | 194.0 ± 111.9 | .15 |
† P < .05 versus HTN group and HTN and DM group.
LA Strain and Strain Rate
LA Strain
The comparison of LA strain indexes among the study groups is shown in Figure 2 . PALS was lower in patients with hypertension (29.0 ± 6.5%) and patients with diabetes (24.7 ± 6.4%) than in controls (39.6 ± 7.8%) and was further depressed in patients with diabetes and hypertension (18.3 ± 5.0%) (ANOVA P < .0001). Similar results were observed for ALS early (controls, 19.1 ± 7.3%; patients with hypertension, 12.5 ± 6.1%; patients with diabetes, 8.8% ± 6.0%; patients with diabetes and hypertension, 5.2 ± 3.8%; P < .0001) and ALS late (controls, 20.4 ± 5.0%; patients with hypertension, 16.6 ± 3.3%; patients with diabetes, 15.9% ± 3.5%; patients with diabetes and hypertension, 13.1 ± 3.1%; P < .0001). An inverse trend was found for time to PALS, expressed as a percentage of RR interval duration (controls, 44.5 ± 5.8%; patients with hypertension, 49.3 ± 9.1%; patients with diabetes, 51.2 ± 7.9%; patients with diabetes and hypertension, 53.7 ± 9.8%; P < .0001). General linear modeling analysis revealed no significant interactions between diabetes and hypertension. Figure 3 illustrates LA strain patterns in four example patients taken from different study groups. Segmental values of PALS, calculated by averaging values measured in each LA wall, are shown in Table 3 . Between-group differences were found in all LA walls, whereas no differences across LA walls were observed within each group. Two-way ANOVA, performed by considering study group and LA wall as main factors, confirmed a significant impact of group ( P < .0001), with no effect of LA wall ( P = .35) and no significant interaction ( P = .46).


LA wall | Controls | HTN group | DM group | HTN and DM group | P |
---|---|---|---|---|---|
Septal | 37.2 ± 7.5 | 27.9 ± 6.5 | 23.1 ± 5.8 | 18.5 ± 6.8 | <.0001 |
Lateral | 41.0 ± 8.7 | 29.4 ± 7.0 | 25.2 ± 7.2 | 19.4 ± 7.5 | <.0001 |
Inferior | 42.3 ± 8.9 | 29.8 ± 7.4 | 26.9 ± 6.9 | 19.1 ± 6.8 | <.0001 |
Anterior | 38.0 ± 6.3 | 28.7 ± 6.1 | 23.5 ± 6.4 | 19.0 ± 6.7 | <.0001 |
P | .24 | .30 | .53 | .79 |
LA Strain Rate
The comparison of LA strain rate indexes among the study groups is shown in Figure 4 . ALSR syst was reduced in patients with hypertension (1.0 ± 0.4 sec −1 ) and patients with diabetes (1.0 ± 0.4 sec −1 ) compared with the controls (1.3 ± 0.5 sec −1 ) and was further depressed in patients with diabetes and hypertension (0.6 ± 0.3 sec −1 ) (ANOVA P < .0001). Similar results were observed for ALSR early (controls, −1.8 ± 0.7 sec −1 ; patients with hypertension, −1.3 ± 0.4 sec −1 ; patients with diabetes, −1.3 ± 0.3 sec −1 ; patients with diabetes and hypertension, −0.9 ± 0.4 sec −1 ; P < .0001). No differences in ALSR late were observed (controls, −2.2 ± 0.6 sec −1 ; patients with hypertension, −1.9 ± 0.4 sec −1 ; patients with diabetes, −2.0 ± 0.4 sec −1 ; patients with diabetes and hypertension, −1.9 ± 0.6 sec −1 ; P = .62). Again, general linear modeling analysis revealed no significant interaction terms between diabetes and hypertension.
Regression Analysis
Univariate correlations of LA strain and strain rate indexes in the overall study population are listed in Tables 4 and 5 . Hypertension and diabetes showed negative associations with PALS, ALS early , ALS late , ALSR syst , and ALSR early and a positive association with time to PALS. No association with ALSR late was found. Most LA strain and strain rate indexes also showed significant relationships with body mass index, LV mass and geometry, and indexes of LV systolic and diastolic function.
Variable | PALS | TPLS | ALS early | ALS late | ||||
---|---|---|---|---|---|---|---|---|
R | P | R | P | R | P | R | P | |
Age | −0.17 | .019 | −0.03 | .72 | −0.22 | .0048 | −0.03 | .70 |
Male gender | −0.03 | .72 | 0.12 | .11 | 0.01 | .85 | 0.09 | .26 |
Body mass index | −0.28 | <.0001 | 0.12 | .13 | −0.15 | .040 | −0.33 | <.0001 |
Diabetes mellitus | −0.56 | <.0001 | 0.25 | <.0001 | −0.50 | <.0001 | −0.37 | <.0001 |
Hypertension | −0.35 | <.0001 | 0.15 | .044 | −0.26 | <.0001 | −0.31 | <.0001 |
Heart rate | −0.01 | .86 | −0.14 | .07 | 0.01 | .91 | −0.04 | .56 |
SBP | −0.10 | .18 | −0.08 | .34 | −0.06 | .44 | −0.08 | .35 |
DBP | −0.03 | .77 | 0.04 | .64 | 0.05 | .48 | −0.11 | .14 |
Indexed LVEDV | 0.08 | .33 | −0.18 | .033 | 0.10 | .22 | 0.01 | .96 |
Indexed LVESV | 0.02 | . 78 | −0.14 | .062 | 0.06 | .52 | −0.04 | .61 |
Indexed LV mass | −0.34 | <.0001 | −0.06 | .45 | −0.23 | .0022 | −0.34 | <.0001 |
Relative wall thickness | −0.31 | <.0001 | 0.23 | .0015 | −0.30 | <.0001 | −0.16 | .032 |
LV ejection fraction | 0.18 | .011 | −0.08 | .33 | 0.13 | .063 | 0.18 | .023 |
E/A ratio | 0.20 | .0067 | −0.25 | .0007 | 0.17 | .022 | 0.13 | .069 |
Sm | 0.27 | <.0001 | 0.03 | .70 | 0.23 | .0014 | 0.16 | .027 |
Em | 0.44 | <.0001 | −0.19 | .011 | 0.51 | <.0001 | 0.17 | .018 |
Am | −0.02 | .78 | 0.12 | .11 | −0.11 | .13 | 0.10 | .18 |
E/Em ratio | −0.37 | <.0001 | 0.10 | .18 | −0.45 | <.0001 | −0.14 | .065 |
Maximal LAVi | −0.13 | .08 | 0.09 | .25 | −0.09 | .21 | −0.06 | .41 |
Precontraction LAVi | −0.18 | .014 | 0.05 | .51 | −0.17 | .018 | −0.15 | .056 |
Minimal LAVi | −0.08 | .29 | 0.05 | .50 | −0.04 | .58 | −0.02 | .83 |
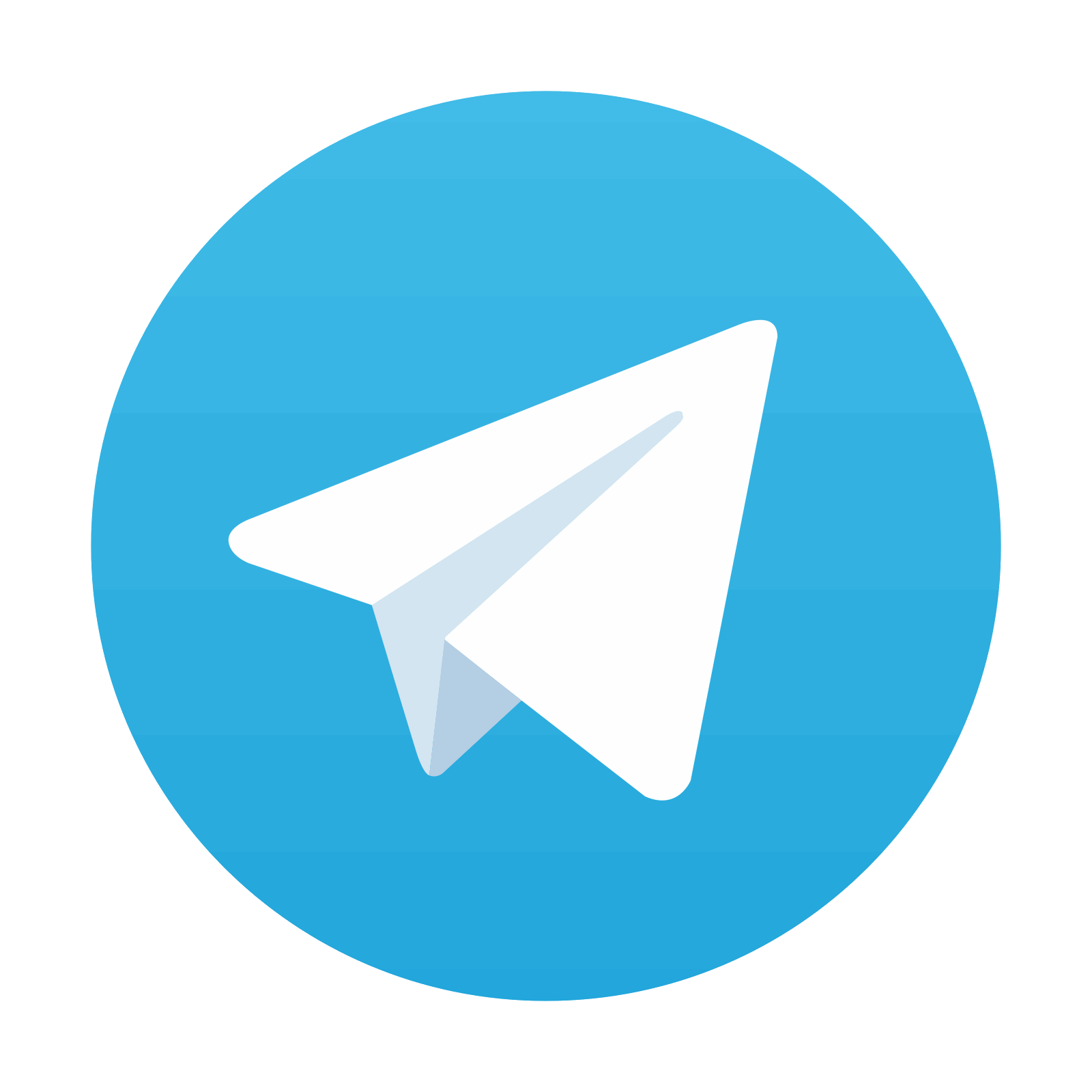
Stay updated, free articles. Join our Telegram channel

Full access? Get Clinical Tree
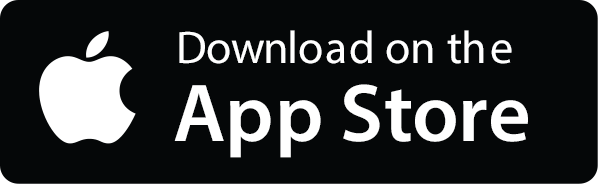
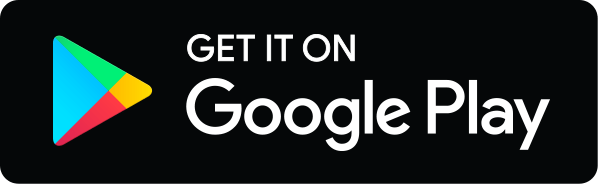
