Background
Hypertrophic cardiomyopathy (HCM) is the most common form of inherited cardiomyopathy. Echocardiography is the mainstay of screening and disease surveillance, and genetic testing has identified a carrier population without hypertrophy. The aim of this study was to investigate whether changes in left ventricular (LV) function are detectable before the advent of hypertrophy.
Methods
Fourteen children with genotype-positive, phenotype-negative HCM were identified (12 male; median age, 9.14 years; range, 1.91–15.9 years; median weight, 34.6 kg; range, 15–92.1 kg) and compared with age-matched and sex-matched healthy controls. All children underwent full echocardiographic studies using an extensive functional protocol, including two-dimensional dimensions, Doppler tissue imaging, and two-dimensional speckle-tracking echocardiography.
Results
There were no differences in LV wall thickness, chamber dimensions, length, and shortening fraction between the groups. Doppler tissue imaging in children with HCM demonstrated mildly reduced septal velocities, notably A′ (5.9 cm/sec [range, 4–8.9 cm/sec] vs 6.7 cm/sec [range, 5.2–9.5 cm/sec]; P = .009). Circumferential and longitudinal strain was similar between groups. Mean apical circumferential deformation was increased in the HCM group (−24.6 ± 3.8% vs −22.2 ± 2.5%, P = .04). There were significant increases in basal and apical rotation and LV twist in children with HCM, most marked at the apex (11.7 ± 4.4° vs 5.3 ± 2.5°, P = .0001). On receiver operating characteristic curve analysis, apical rotation > 7° conferred 83% sensitivity and 82% specificity for predicting HCM (area under the curve, 0.919; P = .0001).
Conclusions
Increased LV rotation and twist are present in children with genotype-positive, phenotype-negative HCM. Apical rotation on speckle-tracking echocardiography provides good sensitivity and specificity for the prediction of gene-positive HCM and may be a clinically useful early marker of HCM before the onset of hypertrophy.
Hypertrophic cardiomyopathy (HCM) is a common inherited genetic cardiovascular disease. The definition of the characteristic phenotype is one of left ventricular (LV) hypertrophy unexplained by either abnormal loading conditions or systemic disease. Typically the diagnosis has been made after the demonstration of LV hypertrophy > 15 mm in adults or >2 standard deviations above the mean for children on two-dimensional (2D) echocardiography in either symptomatic patients or as part of screening echocardiography. The prevalence is estimated to be 0.2%, equating to 1 in 500 in the general population, with an equal global distribution. Inheritance is in an autosomal-dominant pattern with variable penetrance and expression, with typical phenotypic features usually presenting in the second to third decade of life. However, patients with HCM demonstrate a wide spectrum of disease, with the onset of clinical features possible from early childhood. The presentation and clinical course vary widely from an asymptomatic benign finding to sudden cardiac death as the initial event. The estimated incidence of sudden cardiac death due to HCM in childhood is 1% to 2%.
The advent of commercially available genetic testing has led to the identification of genotype-positive, phenotype-negative (G+P−) population. Recent guidelines have advocated the use of genetic testing in index cases and in first-degree relatives of index cases with identified mutations. At this time, it is possible to identify a pathogenic mutation in 60% to 70% of index cases with positive family histories. Mutations have been identified in genes encoding for sarcomere proteins and sarcomere-associated proteins, with a single mutation capable of producing HCM, but when multiple mutations occur, potentially a more severe phenotype is seen.
Although the availability and indications for genetic testing are increasing in the HCM population, echocardiography remains the mainstay of diagnosis and disease surveillance. Given the potential for early presentation and potential clinical implications, the early identification of at-risk patients is important. Previous studies have used Doppler tissue imaging to try to identify G+P− patients. We sought to determine if it was possible to identify G+P− patients using 2D speckle-tracking echocardiography (STE). STE is a relatively new, angle-independent technique using speckles in grayscale B-mode images to track myocardial deformation, validated against sonomicrometry and magnetic resonance imaging.
Methods
Study Population
A total of 14 first-degree relatives of patients with HCM with known genetic mutations were identified from our outpatient population. The inclusion criterion was a known genetic mutation with no evidence of LV hypertrophy on 2D echocardiographic imaging. This was defined as LV septal and LV posterior wall thickness Z scores < 2.0. The study subjects were matched 1:2 with normal control patients (healthy volunteers) from our normal control database. Control patients were scanned according to the same functional protocol. The study was approved by our institutional research ethics board.
Echocardiography
Echocardiographic studies were performed using either the Vivid 7 and E9 ( n = 12; GE Healthcare, Milwaukee, WI) or the iE33 ( n = 2; Philips Medical Systems, Andover, MA) ultrasound system. All studies were performed at rest in the left lateral position. A standardized clinical functional assessment was undertaken with 2D, M-mode, spectral Doppler, and tissue Doppler images obtained. Particular attention was paid to ensuring optimized short-axis 2D imaging at the level of the mitral valve (base), midventricle, and apex and an apical four-chamber view for strain analysis, in keeping with manufacturers’ recommendations regarding frame rate. A single observer (J.F.) analyzed the echocardiographic data offline. Chamber dimensions and septal and posterior wall thickness were measured from M-mode short-axis acquisitions, and LV volumes and ejection fraction were calculated using the modified Simpson’s method in accordance with standard protocols. Spectral Doppler was used to quantify transmitral inflow with peak E and A waves and aortic outflow peak velocity and duration. These values were used to calculate the E/A ratio and myocardial performance index. Pulsed-wave Doppler tissue imaging acquired from an apical four-chamber view was measured for E′, A′, and S′ velocities just below the level of the mitral valve for both the ventricular septum and the LV lateral wall. Postprocessing was performed using dedicated software (EchoPAC version 6.0.1; GE Vingmed Ultrasound AS, Horten, Norway).
Terminology and Strain Analysis
Only images acquired on the Vivid 7 or E9 ultrasound system were used for subsequent strain analysis. All strain analysis was undertaken using a manufacturer-specific postprocessing system (EchoPAC). Two-dimensional STE was performed for both circumferential and longitudinal deformation. Strain analysis was performed at three levels for circumferential strain (basal, midventricular, and apical) and from an apical four-chamber view for longitudinal strain. The endocardium was manually traced at end-systole, with the range of interest adjusted to incorporate the entire myocardium. Once visual inspection of tracking was accepted, a six-segment model in keeping with standard nomenclature at each level was calculated. Peak strain was defined as the maximal deformation of a segment in systole and is represented as a percentage of the original size. Values for peak strain as indicated by the software were accepted only if adequate tracking was obtained as judged by visual inspection. Global or mean strain is the average of the segments at each level and was calculated only if adequate tracking was obtained in a minimum of four segments. Rotation is the clockwise or counterclockwise movement of the heart along the long axis of the left ventricle as seen in the short axis when viewed from the apex. Peak rotation was calculated at both the base and the apex and measured in degrees. LV twist was defined as the peak net difference in rotation at a simultaneous time point between the base and apex of the left ventricle and measured in degrees. Examples of circumferential strain curves and rotational profiles are depicted in Figure 1 .

Statistical Analysis
Data are expressed as mean ± SD, ranges, and percentages as appropriate. Standard 2D echocardiographic parameters, tissue Doppler, and speckle-tracking echocardiographic values were compared using unpaired t tests, with P values < .05 accepted as indicating statistical significance. Receiver operating characteristic (ROC) curves were plotted to determine optimal cutoff values for apical rotation to predict genotype-positive individuals. Interobserver variability was calculated for 10 randomly selected patients across both the G+P− and control groups using Bland-Altman statistics for all strain and rotational parameters. Two observers blinded to the others’ results performed strain measurements, and each observer selected a single best cardiac cycle for analysis from a designated three-beat loop. Variability measures are reported as mean ± SD, and the coefficient of variability was calculated as the standard deviation of the difference of the samples divided by the mean of the paired samples. Because of the small patient groups, further analysis was undertaken to include the absolute difference, expressed as a percentage of the mean of repeated measurements. Intraclass correlation coefficients were also calculated (proportion of variability in the observations that is due to differences between each pair of observations) to validate the reproducibility and reliability of measures. All statistical analyses were performed using GraphPad InStat version 3.01 (GraphPad Software, San Diego, CA).
Results
Clinical Characteristics and 2D Imaging
Fourteen patients were identified from 9 families with four different sarcomeric mutations: MYBPC3 ( n = 6), MYH7 ( n = 5), MYHC ( n = 2), and TPM1 ( n = 1). The 14 patients with HCM were control matched for age, sex, and weight with 28 healthy volunteers taken from our database. Patient characteristics and 2D echocardiographic measurements are detailed in Table 1 . The study groups were well matched for age and weight. In the study group, 12 patients were male, and in the control group 23 of the selected patients were male. No significant differences were found between the groups for baseline 2D LV cavity dimensions and wall thickness. Both septal and posterior wall Z scores all fell within 2 standard deviations of normal (septum, −0.69 to 1.79; posterior wall, −1.74 to 0.69), with hypertrophy excluded in all patients. Both groups had normal LV systolic function, with ejection fractions calculated using the modified Simpson’s equation falling within the normal range.
Variable | Patients with HCM ( n = 14) | Controls ( n = 28) | P |
---|---|---|---|
Age (y) | 9.8 ± 4.5 | 9.8 ± 4.4 | .98 |
Weight (kg) | 40.3 ± 21.6 | 35.5 ± 17.6 | .45 |
Height (cm) | 140.6 ± 27.5 | 137.6 ± 25.9 | .72 |
BSA (m 2 ) | 1.23 ± 0.47 | 1.16 ± 0.4 | .59 |
Septum (mm) | 7.1 ± 1.4 | 6.7 ± 1.6 | .49 |
Septum Z score | 0.8 ± 0.7 | 0.5 ± 1.1 | .40 |
LV posterior wall (mm) | 6.2 ± 1.2 | 5.5 ± 1.2 | .13 |
LV posterior wall Z score | −0.04 ± 0.8 | −0.50 ± 1.0 | .14 |
LVEDD (mm) | 42.6 ± 6.3 | 41.8 ± 5.7 | .70 |
LVEDD Z score | 0.3 ± 1.3 | 0.7 ± 1.5 | .46 |
LV length (mm) | 67.4 ± 12.6 | 66.1 ± 11.3 | .74 |
LV mass indexed to BSA (g/m 2 ) | 68.4 ± 10.9 | 64.3 ± 11.5 | .27 |
Ejection fraction (%) | 64.5 ± 5.9 | 64.2 ± 6.4 | .86 |
Tissue Doppler
Pulsed-wave tissue Doppler analysis was performed in all study patients and in 27 of the control subjects. Results are shown in Table 2 , E′, A′, and S′ were measured in both the basal lateral wall and the septum. All indices in the lateral wall showed no significant differences between the groups. In the basal septum, A′ velocity was significantly lower in the patient group compared with the normal control group. The ratios of transmitral E wave velocity to septal and lateral E′ velocities were not different between both groups.
Variable | HCM ( n = 14) | Control ( n = 28) | P |
---|---|---|---|
Lateral | |||
E′ (cm/sec) | 18.8 ± 2.5 | 19.5 ± 3.5 | .51 |
A′ (cm/sec) | 7.80 ± 2.1 | 7.10 ± 1.5 | .95 |
S′ (cm/sec) | 11.2 ± 2.3 | 11.0 ± 2.7 | .88 |
E/E′ ratio | 5.30 ± 1.3 | 5.70 ± 1.4 | .43 |
Septal | |||
E′ (cm/sec) | 14.1 ± 2.1 | 15.5 ± 2.6 | .09 |
A′ (cm/sec) | 5.80 ± 1.1 | 6.80 ± 1.0 | .01 |
S′ (cm/sec) | 8.20 ± 1.2 | 8.90 ± 1.2 | .07 |
E/E′ ratio | 7.00 ± 1.6 | 7.30 ± 1.6 | .60 |
Circumferential and Longitudinal Strain
Strain analysis was performed in 12 study patients who had had their initial echocardiographic assessments performed using a GE ultrasound system, because of the need for vendor-specific raw data for postprocessing on the EchoPAC system. Frame rates were optimized for speckle-tracking strain analysis, with medians of 75 frames/sec (range, 35–133 frames/sec) for short-axis imaging and 64 frames/sec (range, 33–129 frames/sec) for apical images. For the 12 patients in whom strain measurements were attempted, analysis was technically possible, with good tracking at the majority of segments at the level of base, with the lowest success rate in the posterior segment, for which only 75% (nine of 12) success was achieved. This pattern was reproduced at the midventricular segments, again with posterior segment tracking lower in both the study and control groups (67% and 71%, respectively). Only true apical cuts below the level of the papillary muscles were accepted for analysis; this was more frequently attained in the study population compared with the control group, with nine control subjects excluded from apical segmental analysis. Tracking was attained in the majority of segments, with the posterior and lateral segments having lower success rates (75% in the study group vs 68% in the control group).
Table 3 illustrates the mean and segmental peak systolic strain values for circumferential and longitudinal strain measurements. No significant differences were found in mean circumferential basal and midventricular strain values ( Figure 2 ). In the G+P− group, a significant increase in peak systolic circumferential strain was found at the apical level (−24.6 ± 3.8% vs −22.2 ± 2.5, P = .04), although given the relatively small study population, this should be interpreted with caution. For the majority of the segmental analyses, there were few significant differences between the groups. In the circumferential strain analysis, certain segments did demonstrate a statistical difference, including reduced values for G+P− patients at the basal lateral position (−18.4 ± 2.7% vs −22.7 ± 4.7%, P = .008) and the mid lateral segment (−16.7 ± 3.9% vs −20.4 ± 4.7%, P = .04). Interestingly, there were increases in strain values in G+P− patients at the mid anterior septum (−28.9 ± 5.4% vs −23.3 ± 5.0%, P = .003), apical anterior septum (−26.9 ± 5.6% vs −21.5 ± 4.6%, P = .007), and apical septum (−29.4 ± 5.8% vs −23.2 ± 5.4%, P = .005). For the different longitudinal strain measurements, no significant segmental differences were found.
Variable | Patients with HCM | Controls | P |
---|---|---|---|
Circumferential strain, basal level (%) | |||
Anterior septal | −26.5 ± 5.7 | −23.7 ± 5.5 | .15 |
Anterior | −21.1 ± 3.1 | −18.8 ± 5.7 | .21 |
Lateral | −18.4 ± 2.7 | −22.7 ± 4.7 | .008 |
Posterior | −19.3 ± 3.4 | −21.8 ± 6.4 | .28 |
Inferior | −20.7 ± 3.8 | −22.6 ± 4.4 | .24 |
Septal | −25.3 ± 5.0 | −25.2 ± 4.8 | .95 |
Mean | −22.2 ± 2.7 | −22.7 ± 2.8 | .62 |
Circumferential strain, midventricular level (%) | |||
Anterior septal | −28.9 ± 5.4 | −23.3 ± 5.0 | .003 |
Anterior | −23.8 ± 6.4 | −21.0 ± 4.4 | .12 |
Lateral | −16.7 ± 3.9 | −20.4 ± 4.7 | .04 |
Posterior | −17.1 ± 4.1 | −19.6 ± 19.8 | .26 |
Inferior | −21.2 ± 4.7 | −21.0 ± 5.2 | .94 |
Septal | −27.9 ± 5.2 | −25.7 ± 5.7 | .28 |
Mean | −23.0 ± 3.2 | −22.2 ± 3.3 | .47 |
Circumferential strain, apical level (%) | |||
Anterior septal | −26.9 ± 5.6 | −21.5 ± 4.6 | .007 |
Anterior | −24.3 ± 6.1 | −23.6 ± 4.2 | .73 |
Lateral | −21.5 ± 6.7 | −20.9 ± 4.8 | .83 |
Posterior | −21.1 ± 5.1 | −20.7 ± 5.8 | .88 |
Inferior | −24.3 ± 4.1 | −21.9 ± 2.4 | .07 |
Septal | −29.4 ± 5.8 | −23.2 ± 5.4 | .005 |
Mean | −24.6 ± 3.8 | −22.4 ± 2.5 | .04 |
Longitudinal strain, apical four-chamber (%) | |||
Basal septal | −19.7 ± 2.4 | −19.6 ± 2.3 | .83 |
Mid septal | −20.6 ± 2.4 | −21.9 ± 2.4 | .12 |
Apical septal | −22.6 ± 3.5 | −23.2 ± 5.7 | .71 |
Apical lateral | −21.2 ± 4.1 | −20.9 ± 5.0 | .89 |
Mid lateral | −21.6 ± 4.3 | −20.2 ± 4.7 | .38 |
Basal lateral | −22.2 ± 3.5 | −22.3 ± 5.9 | .95 |
Mean | −21.3 ± 2.2 | −21.4 ± 2.7 | .95 |
Rotation | |||
Basal rotation (°) | −8.7 ± .3.7 | −5.8 ± 1.9 | .0015 |
Basal rotation rate (°/sec) | −90.9 ± 29 | −102 ± 29.6 | .27 |
Apical (°) | 11.7 ± 4.3 | 5.3 ± 1.9 | <.0001 |
Apical rotation rate (°/sec) | 108 ± 31.9 | 98.5 ± 23.4 | .39 |
LV twist (°) | 13.9 ± 4.7 | 8.8 ± 2.5 | .0008 |
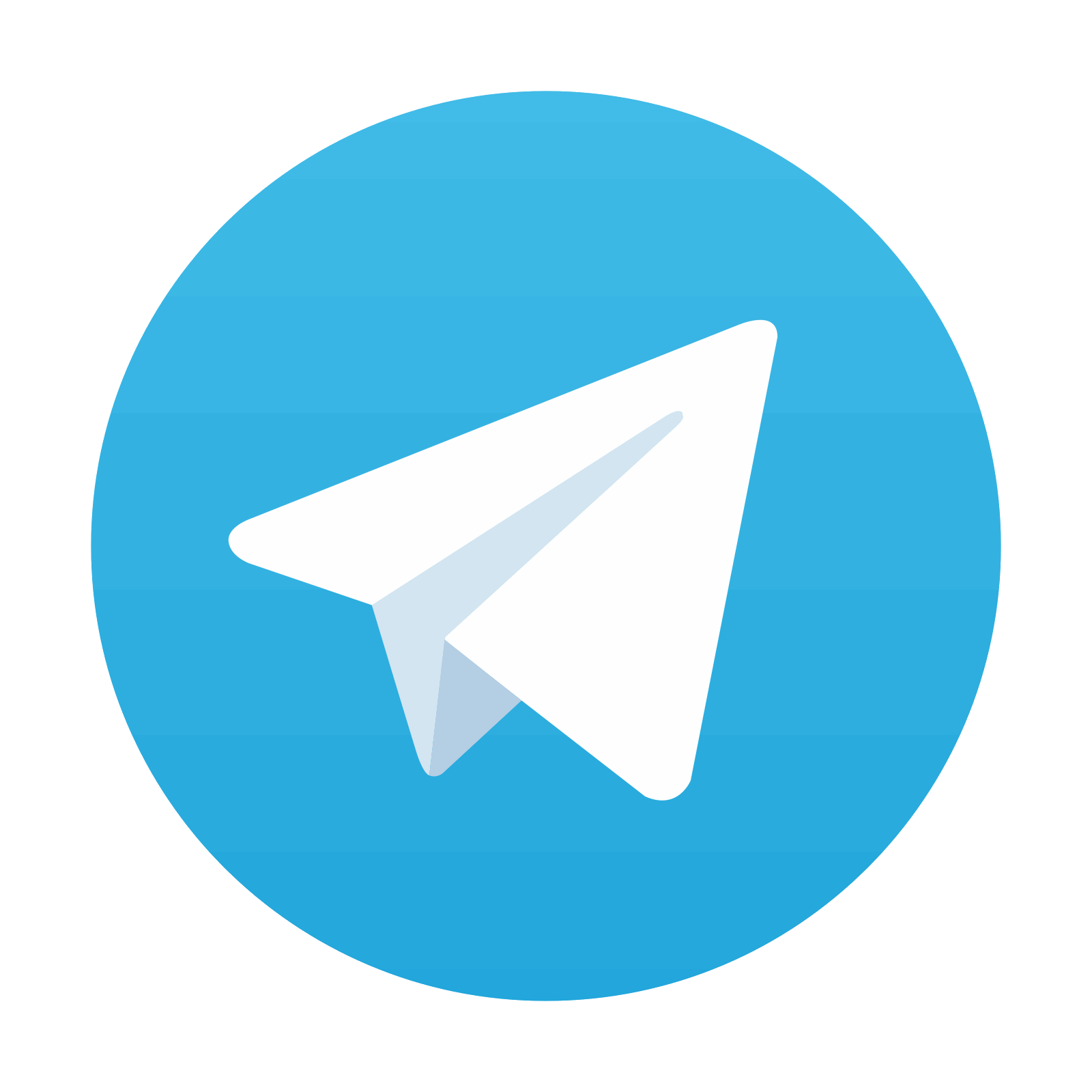
Stay updated, free articles. Join our Telegram channel

Full access? Get Clinical Tree
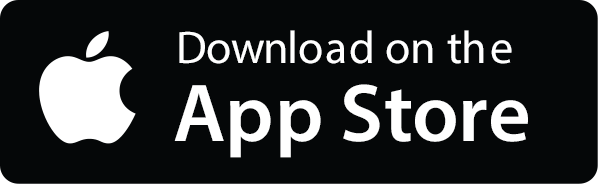
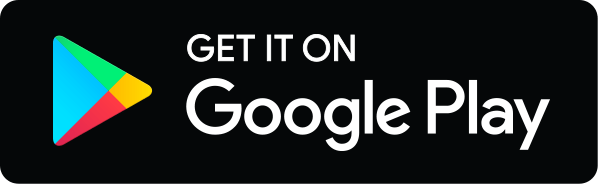
