The introduction of devices for transcatheter aortic valve implantation, mitral repair, and closure of prosthetic paravalvular leaks has led to a greatly expanded armamentarium of catheter-based approaches to patients with regurgitant as well as stenotic valvular disease. Echocardiography plays an essential role in identifying patients suitable for these interventions and in providing intra-procedural monitoring. Moreover, echocardiography is the primary modality for post-procedure follow-up. The echocardiographic assessment of patients undergoing trans-catheter interventions places demands on echocardiographers that differ from those of the routine evaluation of patients with native or prosthetic valvular disease. Consequently, the European Association of Echocardiography in partnership with the American Society of Echocardiography has developed the recommendations for the use of echocardiography in new transcatheter interventions for valvular heart disease. It is intended that this document will serve as a reference for echocardiographers participating in any or all stages of new transcatheter treatments for patients with valvular heart disease.
Attention ASE Members:
ASE has gone green! Visit www.aseuniversity.org to earn free continuing medical education credit through an online activity related to this article. Certificates are available for immediate access upon successful completion of the activity. Nonmembers will need to join the ASE to access this great member benefit!
Introduction
Until recently, transcatheter therapy for valvular heart disease was limited to balloon valvuloplasty. However, the introduction of devices for transcatheter aortic valve implantation (TAVI), mitral repair, and closure of prosthetic paravalvular leaks has led to a greatly expanded armamentarium of catheter-based approaches to patients with regurgitant as well as stenotic valvular disease.
Echocardiography plays an essential role in identifying patients suitable for these interventions and in providing intra-procedural monitoring. Moreover, echocardiography is the primary modality for post-procedure follow-up. The echocardiographic assessment of patients undergoing transcatheter interventions places demands on echocardiographers that differ from those of the routine evaluation of patients with native or prosthetic valvular disease. Consequently, anticipating growing use of transcatheter valve therapies and, along with it, an expanding need for informed echocardiographic evaluation, the European Association of Echocardiography in partnership with the American Society of Echocardiography has developed these recommendations. It is intended that this document will complement the earlier ASE guideline for Echocardiography-guided interventions and will serve as a reference for echocardiographers participating in any or all stages of new transcatheter treatments for patients with valvular heart disease.
Introduction
Until recently, transcatheter therapy for valvular heart disease was limited to balloon valvuloplasty. However, the introduction of devices for transcatheter aortic valve implantation (TAVI), mitral repair, and closure of prosthetic paravalvular leaks has led to a greatly expanded armamentarium of catheter-based approaches to patients with regurgitant as well as stenotic valvular disease.
Echocardiography plays an essential role in identifying patients suitable for these interventions and in providing intra-procedural monitoring. Moreover, echocardiography is the primary modality for post-procedure follow-up. The echocardiographic assessment of patients undergoing transcatheter interventions places demands on echocardiographers that differ from those of the routine evaluation of patients with native or prosthetic valvular disease. Consequently, anticipating growing use of transcatheter valve therapies and, along with it, an expanding need for informed echocardiographic evaluation, the European Association of Echocardiography in partnership with the American Society of Echocardiography has developed these recommendations. It is intended that this document will complement the earlier ASE guideline for Echocardiography-guided interventions and will serve as a reference for echocardiographers participating in any or all stages of new transcatheter treatments for patients with valvular heart disease.
Transcatheter Aortic Valve Implantation
TAVI is a new technique with the potential for transforming the treatment of patients with aortic stenosis (AS). The technology is currently being evaluated in patients with severe symptomatic AS who are at high risk for conventional open heart surgery or considered inoperable. In the future, however, there may be expanded indications for TAVI. At this stage of development, TAVI remains a challenging technology that requires a multidisciplinary team approach involving interventional cardiologists, surgeons, anaesthesiologists, and imaging specialists. Imaging indeed plays a central role in successfully implementing TAVI as it is needed at each step of the procedure including patient selection, choice of procedural access, prosthetic choice and sizing, procedural guidance, and detection of early and late complications.
Introduction
In April 2002, Cribier et al. reported the first successful implantation of a bovine pericardial bioprosthesis mounted within a stainless steel balloon-expandable stent in a patient with severe AS who presented in cardiogenic shock. After this first-in-man implantation, the procedure was attempted on a compassionate basis in several other patients with an equine pericardial modification of the original valve design. Valve placement was initially done via an antegrade transseptal approach. This was a challenging procedure, owing to the need for transseptal puncture, the tortuous navigation of the valve assembly across the mitral and aortic valves, and the guide wire interaction with the mitral valve apparatus, which often caused severe mitral regurgitation (MR). These limitations prompted technical improvements in the size and steer-ability of the delivery system which allowed for the development of the more practical retrograde transfemoral approach. Additional changes in the structure of the valve (processed bovine pericardium and extended skirt height) resulted in the Edwards SAPIEN ™ valve. For patients with poor peripheral vascular access, a transapical approach was subsequently developed. The SAPIEN ™ valve received European approval (CE Mark) for both transfemoral and transapical approaches in 2007.
In 2005, Grube et al. first reported the use of a different type of percutaneous valve system designed for the aortic position, the CoreValve ™ system. This received CE mark in 2007. The CoreValve ™ valve is self-expandable and offers the advantage of being self-centring and partially repositionable.
Expansion and refinement of transcatheter approaches for aortic valve implantation is an area of active research and development with a variety of devices in the pipeline, but only the SAPIEN ™ and CoreValve ™ valves have been approved. Both have been reported to have excellent flow characteristics with core-lab-adjudicated mean aortic valve area (AVA) and mean gradient at 1 year of 1.5 cm 2 and 11 mmHg, respectively, for the SAPIEN ™ valve, and site-reported mean gradients of 8 mmHg at 1 year for the CoreValve ™ .
Current Status of Edwards SAPIEN ™ and CoreValve ™ Systems in Europe and North America
European approval of both the Edwards SAPIEN ™ and CoreValve ™ valves was granted in 2007, in the absence of a randomized trial and depending on data from a series of relatively small studies and registry reports. A newer generation modification of the Edwards valve, the Edwards SAPIEN ™ XT, received CE mark in 2010. Both the SAPIEN ™ and CoreValve ™ valves are available in Canada for compassionate use for the treatment of patients with severe AS who are considered inoperable or at very high surgical risk. Although neither of these valves has been approved for commercial or compassionate use in the USA, the Edwards SAPIEN ™ valve was approved for use as an investigational device in a pivotal trial (PARTNER US; Placement of AoRTic traNscatheterER valves) and results were recently published. A US randomized multicentre trial evaluating the CoreValve ™ valve is underway, and a US randomized multicentre trial evaluating the SAPIEN ™ XT valve has been approved.
Transcatheter Aortic Valve Prostheses
Echocardiographers need to be familiar with the design of the two available prostheses, the Edwards SAPIEN ™ valve and the Medtronic CoreValve ™ valve. Each valve has specific characteristics and different aortic anatomic requirements. Thus, a precise echocardiographic evaluation is essential for appropriate patient selection.
‘The Edwards SAPIEN ™ valve’ is a balloon-expandable valve based on Cribier’s original design. The current-generation valve is composed of a cylindrical stainless steel balloon-expandable stent into which three symmetric leaflets made of bovine pericardium are mounted ( Figure 1 A). The stent also has a polyethylene terephthalate fabric skirt that decreases paravalvular leaks. The valve is available in two sizes, oversized in relation to the aortic annulus to reduce the degree of paravalvular regurgitation (PVR); a 23 mm prosthesis for transverse aortic annular diameters of 18–21 mm (measured at the level of aortic cusp insertion) and a 26 mm prosthesis for aortic annular diameters of 22–25 mm. The valve may be deployed via a transfemoral or transapical route. Because of the large valve size, sheath size is a significant factor with respect to procedural complications.
A newer generation valve, the Edwards SAPIEN ™ XT as well as NovaFlex ™ transfemoral and Ascendra ™ transapical delivery systems, has recently received CE mark in Europe. The delivery system has a smaller calibre (18 F) and the valve stent is thinner and comprised of a cobalt-chromium frame ( Figure 1 B), providing improved radial strength and enhanced circularity.
Transfemoral ‘retrograde’ delivery technique
Transfemoral placement is undertaken using an introducer sheath with an internal calibre of 22 or 24 F depending on the valve size. After femoral artery vascular access is achieved, a balloon aortic valvuloplasty is performed during rapid right ventricular pacing. Subsequently, the stented valve, crimped onto the delivery balloon, is advanced under fluoroscopic guidance, using a manually deflectable-guiding catheter that facilitates atraumatic navigation of the valve around the aortic arch and centring the guide wire through the native valve commissures. The valve is then positioned in a subcoronary position using fluoroscopic and/or transoesophageal echocardiography (TEE) guidance. Once the proper position has been achieved, the valve is deployed under rapid right ventricular pacing.
Transapical delivery technique
This more invasive approach requires an anterolateral mini-thoracotomy, ideally performed in a hybrid operative suite. Prior to the creation of a sterile field, the location of the apex is identified by palpation and confirmed by transthoracic echocardiography (TTE). Subsequently, the pericardium is opened near the left ventricular (LV) apex, a sheath is inserted directly into the LV cavity, and a guide wire is used to cross the aortic valve under fluoroscopic and TEE guidance. Aortic balloon valvuloplasty is then performed during rapid pacing after which the 26 F sheath is inserted permitting deployment of the prosthetic valve.
Procedural success and early clinical outcomes
Recent preliminary data reported from the SAPIEN ™ Aortic Bioprosthesis European Outcome SOURCE Registry, a clinical post-commercialization ‘real-world’ registry of patients undergoing TAVI with the Edwards SAPIEN ™ valve, included 1038 consecutive patients (575 apical and 463 transfemoral) from 32 sites. Overall short-term procedural success was 93.8%. The incidence of valve embolization and coronary obstruction was 0.6 and 0.3%, respectively. Thirty-day mortality was 6.3% in transfemoral patients and 10.3% in transapical patients. Illustrating the steep learning curve with the procedure, Webb et al., reporting a single institution’s experience of 113 patients noted that mortality fell from 12.3% in the initial half to 3.6% in the second half of the experience. In the report of 1-year results for Cohort B of the PARTNER trial (inoperable patients randomized to either TAVI or medical therapy including valvuloplasty), 1-year survival was 50.7% in the TAVI arm vs. 30.7% in the medical arm. This is the only randomized trial to date comparing TAVI with surgery or medical therapy. The results of Cohort A [699 high-risk surgical patients, Society of Thoracic Surgeons (STS) score ≥10 or a predicted operative mortality ≥15%, randomized to either surgery or transfemoral/transapical valve implantation, depending on vascular access] were recently presented, showing non-inferiority with regard to mortality at 1 year. In both PARTNER and the 1-year SOURCE reports, vascular complications at the time of intervention were associated with reduced survival.
‘The CoreValve ™ ReValving system prosthesis consists of porcine pericardial tissue sewn to form a trileaflet valve mounted within an asymmetrical self-expanding nitinol frame ( Figure 2 ). Once deployed, the point of coaptation of the leaflets is supra-annular. The current-generation nitinol frame is >50 mm in length and is hourglass-shaped. The lower portion of the frame affixes the valve to the LV outflow tract (LVOT) and has the greatest radial strength, but care must be taken not to impinge on the anterior mitral leaflet. The mid-portion of the prosthesis has a constrained waist that must be deployed at the level of the sinuses of Valsalva and the coronary ostia, so as not to jeopardize coronary flow. It has a high radial force to firmly anchor the prosthesis and prevent migration or paravalvular leakage. Finally, the upper section (outflow) has the lowest radial force and is designed to fix and stabilize the prosthesis in the ascending aorta.
The prosthetic size is determined by the external diameter of the ventricular end; the 26 and 29 mm prostheses have mid-portion diameters of 22 and 24 mm, aortic end-diameters of 40 and 43 mm, and prosthetic lengths of 55 and 53 mm, respectively. The 26 mm prosthesis is designed for patients with aortic annular diameters of 20–23 mm, whereas the 29 mm prosthesis is suitable for patients with 24–27 mm aortic annuli. However, the design of this prosthesis, with a broader upper segment to secure it to the ascending aortic wall, mandates that the height and width of the aortic sinuses and the ascending aortic diameter be carefully measured. In the presence of ascending aortic diameters >45 mm and/or aortic annular diameters, <20 or >27 mm, this device should not be implanted. The delivery system of the CoreValve ™ has evolved from an initial 25 F to the current 18 F device, which allows completely percutaneous arterial access and the possibility of avoiding general anaesthesia.
CoreValve ™ delivery technique
The CoreValve ™ is designed for retrograde delivery through arterial access, although there are case reports of deployment using a transapical route. Vascular access can be obtained with or without standard surgical cut down of the common iliac, common femoral, or subclavian arteries. The procedure can be performed under general anaesthesia or with local anaesthesia in combination with mild systemic sedation/analgesia. After femoral artery access has been secured, a balloon aortic valvuloplasty of the calcified stenotic aortic valve is performed during rapid right ventricular pacing. After this valvular dilation, the prosthesis is deployed and implanted retrogradely over a stiff guide wire. Post-dilation of the CoreValve ™ prosthesis can be performed at the discretion of the operator depending on the perceived proper placement of the device angiographically and the degree of aortic regurgitation.
Procedural success and early clinical outcomes
Recently, Piazza et al. reported procedural success and outcomes at 30 days in 636 patients with symptomatic AS, who underwent implantation with the third-generation CoreValve ™ during the first year of the multicentre expanded CoreValve ™ evaluation registry. Procedural success was achieved in 97.2% patients. Procedural death occurred in 1.5% of the patients. The combined incidence of procedural death, myocardial infarction, and stroke was 2.5%. At 30 days, all-cause mortality was 8%, one half of these deaths being judged to be procedure-related. Permanent pacemaker implantation was needed in 9.3% of the patients. TTE performed prior to discharge demonstrated a significant reduction in mean transaortic pressure gradients (from 49 ± 14 to 3 ± 2 mmHg).
Patient Selection for Transcatheter Aortic Valve Implantation
Appropriate screening and patient selection, based on clinical criteria and careful analysis of cardiovascular anatomy, is crucial for the success of TAVI. Selection of candidates is complex and involves a multidisciplinary team evaluation and the use of multiple imaging modalities in order to fully delineate the anatomy of the aortic valve, aorta, and peripheral vasculature. Although not the focus or scope of these recommendations, the clinical criteria for patient selection are briefly described below.
Clinical criteria
The consensus statement on TAVI from 2008 recommends the use of this procedure in high-risk patients or those with contraindications for surgery. Risk evaluation is usually performed using the Logistic European System for Cardiac Operative Risk Evaluation (EuroSCORE) and/or the STS Predicted Risk of Mortality Score. High surgical risk is defined by a logistic EuroSCORE of ≥15–20% or an STS mortality risk score of ≥10%. However, these scores have clear limitations and their predictive capacity may be reduced in high-risk patients who represent a small proportion of the population from which the scores were constructed. Moreover, the suitability of these scores for assessing risk during TAVI has been questioned since co-morbidities that are less significant for TAVI considerably increase the risk of surgical aortic valve replacement (AVR), especially in elderly patients.
Patient characteristics that might favour TAVI over AVR include prior cardiac surgery with grafts and/or adhesions, previous chest radiation therapy, porcelain aorta, liver cirrhosis, pulmonary hypertension, right ventricular failure, or marked patient frailty. Nevertheless, TAVI is not recommended for patients whose life expectancy is less than 1 year or who cannot expect significant improvement in quality of life. In clinically suitable patients for TAVI, the evaluation of the size, tortuosity, and calcification of peripheral arteries by angiography, multislice computed tomography (MSCT), or magnetic resonance imaging assists in choosing between transfemoral and transapical approaches.
Echocardiographic evaluation
Echocardiography is critical in the assessment of candidates for TAVI, providing both anatomic and haemodynamic information.
Transthoracic echo
Transthoracic echo plays a key role in establishing the presence of severe AS with Doppler assessment of peak and mean transaortic gradients as well as AVA calculation by the continuity equation. According to the current guidelines, severe AS is defined by an AVA of <1cm 2 (<0.6 cm 2 /m 2 ) or a mean aortic valve gradient of >40 mmHg. However, the requirements for SAPIEN ™ implantation as defined in the PARTNER trial are a valve area of <0.8 cm 2 , a peak transvalvular velocity of ≥4 m/s and/or a mean gradient of ≥40 mmHg, targeting patients with particularly severe (critical) stenosis.
Although a full discussion of the pitfalls in diagnosing severe AS is beyond the scope of this document, two groups where the diagnosis of severe AS may be challenging should be noted. Patients may present with low gradients, despite valve areas within the severe range in the presence of severe LV systolic dysfunction. This may pose the dilemma of distinguishing between true severe AS and pseudo-severe AS in which reduced LV systolic function contributes to the reduction in calculated valve area. Dobutamine stress echocardiography has been shown to distinguish between the two and provide useful information concerning contractile reserve. Additionally, attention has recently been focused on patients with low gradients and normal LV ejection fraction but low flow AS for whom calculation of projected valve area under normal flow states may be useful. Cardiac catheterization is no longer recommended for determining the severity of AS, except in exceptional cases with conflicting data on echocardiography.
Once the diagnosis of severe valvular AS is clear, echocardiography must determine whether the patient’s anatomy is suitable for TAVI. Using TTE, assessing the annular dimension and detailed anatomic characteristics of the aortic valve, including the number, mobility, and thickness of cusps, as well as the extent and distribution of calcification should be described. Currently, bicuspid aortic valve is an exclusion criterion for TAVI because an elliptical valvular orifice may predispose to an increased risk of incomplete and incorrect deployment of the aortic prosthesis. Moreover, the risk of aortic complications, such as spontaneous aortic dissection, may be increased, due to abnormal arterial wall structure. That said, cases of successful TAVI in bicuspid AS have been reported.
Accurate sizing is critical to TAVI procedural success. Annular dimension is a key measurement as this determines eligibility for TAVI and guides the selection of valve type and size. Prior sections have described criteria for selecting valve size based on aortic annular, sinus of Valsalva, and ascending aortic dimensions.
Undersizing the prosthesis can result in device migration or significant paravalvular aortic regurgitation. Moreover, even if severe procedural complications do not occur, prosthesis mismatch may result. Oversizing predisposes to complications related to vascular access or to difficulties when crossing the native aortic valve with the delivery system. There is also the risk of under-expansion with consequent redundancy of leaflet tissue, creating folds that will generate regions of compressive and tensile stress that may cause central aortic regurgitation or reduction in valve durability.
Annular diameter is typically measured in systole, in a parasternal long-axis view, zoomed on the LVOT. The measurement is taken at the point of insertion of the aortic valve cusps, from tissue–blood interface to blood–tissue interface—trailing edge to leading edge ( Figure 3 A), regardless of the degree of calcification of the aortic cusps. When transthoracic two-dimensional (2D) echocardiographic measurements of the annulus are uncertain, particularly if measurements are near critical cut-offs for valve selection or if calcification extends from the aortic valve onto either the anterior mitral leaflet or the septum, TEE ± 3D evaluation may be necessary ( Figure 3 B). The resolution of 3D TTE is currently inadequate for assistance in annular measurements in most subjects.
LV and right ventricular dimensions and function, aortic regurgitation, and the structure and function of the other valves should be evaluated. The presence of haemodynamically significant LVOT obstruction due to basal septal hypertrophy represents a contraindication as septal hypertrophy is a potential cause of prosthesis displacement during or after implantation. These patients are potential candidates for myomectomy. The presence of an LV thrombus must be excluded, as it represents a contraindication to the procedure. The presence of a patch in the LV as well as significant pericardial calcification is a contraindication for TAVI using the transapical approach.
Transoesophageal echo
TEE is recommended prior to TAVI if there are any concerns about the assessment of the aortic root anatomy, aortic annular size, or number of cusps. Since patients with symptomatic AS tolerate hypotension poorly, sedation should be performed carefully with an emphasis on effective topical anaesthesia.
The aortic root is a direct continuation of the LVOT and extends from the basal attachment of the aortic valvular cusps to the level of the sinotubular junction. The diameter of the root varies considerably along its length, but it is the annular diameter at the level of the basal attachment of the aortic valve cusps, measured in systole, that dictates the size of the prosthesis, irrespective of the type of the valve inserted ( Figure 3 B).
TEE aortic annular measurements correlate well with TTE, although the latter underestimates TEE-measured aortic annular size with a mean difference of 1.36 mm (95% confidence interval, 1.75–4.48 mm). There is concern that the assumption of annular circularity made by 2D echo may result in erroneous annular measurements in patients whose annuli are more oval-shaped. However, a strategy based on 2D TEE measurements has been shown to provide good clinical results when compared with MSCT.
Currently, there is no consensus regarding the gold standard imaging technique for annular sizing, although, from a practical perspective, TTE performs this task adequately in most patients.
Transoesophageal echocardiography protocol
The pre-procedure TEE evaluation may be performed as part of screening or as the initial step of intra-procedural monitoring.
Using the long-axis view (usually around 110–130°), the LVOT and upper septum should be assessed since the presence of a subaortic septal bulge may create an obstacle to proper seating of the aortic prosthesis.
Using short-axis views, the opening of the aortic valve should be classified as central or eccentric and the severity, location, and symmetry of aortic valve calcification accurately described. During TAVI, the prosthesis anchors according to the resistance of the subleaflet tissue. During implantation, the native cusps are crushed against the aortic wall and the differences in the tension–force across the valve may cause asymmetric deployment of the prosthesis and contribute to the risk of compression of the coronary arteries during TAVI.
In order to minimize the risk of coronary occlusion, it is essential to know the distance from the aortic annulus to the ostia of the coronary arteries and to compare this with the length of the cusps measured in a long-axis view. Although the cusps are typically shorter than the annular-ostial distances, patients in whom the cusp length exceeds the annular-ostial distances are at risk of ostial coronary occlusion when the valve is deployed and the native cusps crushed to the side. Although the determination of the right coronary annular-ostial distance should be possible with 2D TEE ( Figure 4 ), measurement of the left coronary annular-ostial distance requires 3D TEE (see below) or MSCT.
It is also important to assess the characteristics of the ascending aorta, the aortic arch, and the descending thoracic aorta since the presence of aortic arch atheromas may increase the risk of peri-procedural embolization and therefore favour a transapical approach.
Peri-procedural echocardiography during transcatheter aortic valve implantation
Two-dimensional echocardiography
Although TTE clearly plays an important role in patient selection for TAVI, its role during the actual procedure is limited. In patients undergoing TAVI via a transapical approach, TTE can be helpful in locating and marking the position of the LV apex in order to guide the thoracotomy. However, there are a number of points to remember when doing this: (i) it is important to use two orthogonal TTE apical views; (ii) the apex should be located with the surgeon and echocardiographer on the same side of the patient so that both can agree on the optimum intercostal space; and (iii) once the skin is marked with the optimal position, it is essential that the patient and/or the skin not be moved. Such movement may occur as surgical drapes are being applied and may change the position of the skin mark relative to the ribs.
The use of peri-procedural TEE is variable. The technique can aid balloon positioning during valvuloplasty, detect post-valvuloplasty aortic regurgitation, aid prosthesis positioning during implantation, confirm prosthesis function immediately post-implantation, and rapidly detect complications. However, the use of peri-procedural TEE usually requires general anaesthesia and the probe may also partially obstruct the optimal fluoroscopic view. Therefore, some operators feel that these disadvantages outweigh the many advantages of peri-procedural TEE. However, it should be noted that the transapical approach will always require general anaesthesia anyway and some centres have reported transfemoral implantation with TEE guidance using only moderate sedation. Moreover, to avoid obstructing the fluoroscopic view, the TEE probe may be retracted during the actual valve implantation and be rapidly repositioned following deployment.
Transnasal TEE is a relatively new technique that can be used to monitor TAVI. Although its image quality is not quite as good as conventional TEE and transnasal TEE does not currently have 3D capability, this approach could be considered in patients where general anaesthesia is not deemed appropriate. Some sites have also adapted intracardiac echo (ICE) for TAVI, although ICE poses additional challenges in securing adequate windows.
As described more fully in a subsequent section, 3D TEE conveys certain advantages over 2D TEE during TAVI. For example, the 3D depth perspective makes it easier to visualize the position of the prosthesis on the balloon relative to the native valve annulus and surrounding structures. It also facilitates appreciation of the guide wire path through the LV and around the mitral valve subvalvular apparatus.
Both transapical and transfemoral TAVI procedures commence with balloon valvuloplasty. This is designed to split the valve commissures and make subsequent valve implantation easier. TEE can be used to guide positioning of the balloon relative to the aortic valve and is especially useful when the valve is not very calcified and, consequently, difficult to image on fluoroscopy. It may also help in the final decision-making concerning the appropriate valve size, because a valve with bulky calcification and small sinuses may require a smaller prosthesis than the annular dimension alone would suggest.
Although balloon inflation is normally performed during rapid right ventricular pacing to reduce cardiac output, the balloon may still migrate during inflation, particularly in patients with extensive subaortic septal hypertrophy or a small sinotubular junction. Loss of right ventricular capture and premature restoration of the native rhythm may also result in balloon migration. TEE may be used to confirm a stable position during inflation and to monitor the behaviour of the calcified aortic cusps during inflation as they are pushed back into the sinuses and towards the coronary ostia ( Figure 5 A).
During deployment of the prosthesis, TEE is very helpful in confirming the correct position of the valve and is usually used in conjunction with fluoroscopy for this purpose. In patients with limited native valve calcification or for valve-in-valve procedures where TAVI is used in the setting of another bioprosthesis, TEE may be the main technique used for guidance.
The optimal position for the Edwards SAPIEN ™ valve is with the ventricular side of the prosthesis positioned 2–4 mm below the annulus in the LVOT. Examples of 2D TEE imaging during prosthesis positioning and deployment are shown in Figure 5 B and C. Since the CoreValve ™ has a different structure, the ventricular edge of the prosthesis should be placed 5–10 mm below the aortic valve annular plane. A normally positioned CoreValve ™ is shown in Figure 6 .
Immediately following deployment, TEE is used to confirm satisfactory positioning and function of the prosthesis ( Figure 7 A and B). This requires a combination of 2D imaging and Doppler evaluation with 3D also used if available. When the prosthesis is positioned too low, it may impinge on the mitral valve apparatus ( Figure 8 ) or it may be difficult to stabilize in patients with marked subaortic septal hypertrophy. The native valve cusps may also fold over the top of the prosthesis and impede its function. If the prosthesis is implanted too high, it may migrate up the aorta, obstruct the coronary ostia, or be associated with significant PVR.
It is important to confirm that all the prosthetic cusps are moving well, that the valve stent has assumed a circular configuration (using 2D or 3D views), and that there is no significant valvular or PVR. Some regurgitation through the prosthesis will be common, whereas the delivery apparatus and/or guide wire remain across the valve and may persist, to a lesser degree, after their removal as it may take a few minutes post-implant for the leaflets to completely recover from being crimped for deployment. Until this occurs, the cusps may not coapt completely and mild valvular regurgitation may be transiently observed. Transgastric TEE views with continuous-wave, pulsed-wave, and colour Doppler should be used to confirm satisfactory prosthetic functioning before the probe is finally removed. This window is essential to ensure that all regurgitant jets are detected ( Figure 9 ).
PVR, not infrequently with multiple jets, is common following TAVI, though trace to mild and with a benign stable course in the majority of patients. On the other hand, severe aortic regurgitation may occur as a consequence of incomplete expansion or incorrect positioning of the device, restricted cusp motion, or inappropriate prosthetic size. An undersized prosthesis is expected to be associated with paravalvular aortic regurgitation. In contrast, an oversized prosthesis may result in suboptimal stent expansion, impaired cusp mobility, and central aortic regurgitation. Moreover, in the presence of severe asymmetric calcification of the native aortic valve, deficient (asymmetric) accommodation of the stent may occur, causing PVR of varying severity. The approach to assessing post-TAVI aortic regurgitation is discussed in detail in a later section. However, in the context of the immediate post-implantation assessment, conventional criteria including using colour jet dimensions, vena contracta, pressure half-time, and quantitative Doppler may all be helpful. Three-dimensional TEE is an additional tool to evaluate the early function of the bioprosthesis and define the severity and precise location of paravalvular and/or central regurgitation. Additionally, the patient’s haemodynamic status and aortography may all help identify the patient with excessive regurgitation.
In the case of moderate paravalvular aortic regurgitation, supplementary balloon dilation can be performed. However, the risk of aortic rupture, cusp trauma, and over dilatation of the stent, all of which might worsen central aortic insufficiency, must be considered. Aortic regurgitation has also been reported as a consequence of residual native aortic valve leaflet tissue prolapsing into the prosthesis, interfering with cusp motion and coaptation. This may result from deficient containment of residual native aortic tissue by the prosthesis and/or positioning the valve too low.
The extreme consequence of prosthesis mismatch (or failed pacing capture) is prosthetic embolism. If the embolization occurs towards the aorta, it might be resolved through successful transcatheter repositioning, but if it happens towards the LV, surgical removal is usually the only option.
During the procedure, the echocardiographer may be alerted to acute, severe hypotension. Possible explanations identifiable by TEE are cardiac tamponade secondary to wire perforation of the left or right ventricle, LV dysfunction, or severe aortic regurgitation. Left ventricular dysfunction with acute wall motion abnormalities may be secondary to ostial occlusion by fragment embolization or by an obstructive portion of the valve frame, sealing cuff, or native cusp. Although this complication may be fatal, successful management of ostial occlusions with percutaneous angioplasty or bypass surgery has been reported.
Another possible complication of TAVI is sudden worsening of MR. This may occur due to right ventricular pacing (LV asynchrony) or as a consequence of prosthetic misplacement with pressure exerted on the anterior mitral leaflet from the ventricular edge of the prosthesis ( Figures 10 and 11 ) or by direct damage or distortion of the subvalvular apparatus. The latter is more common with the antegrade apical approach, as the catheter might trap the subvalvular apparatus when passing through the LV towards the outflow tract. This may cause temporary or, in the case of chordal or leaflet rupture, permanent distortion and severe MR. Careful echocardiographic monitoring of the mitral valve during and after implantation can help avoid this complication.
Rarely, (frequency 0–4%), a tear or rupture of the aortic root may be observed during the procedure after balloon valvuloplasty or prosthesis deployment, especially in the presence of extensive annular calcification or prosthesis oversizing. Inspection of the ascending aorta and aortic arch may also detect aortic cusp fragment embolization or atheroembolism. These complications, along with thrombo-embolism from catheters, air embolism, prolonged hypotension, or arch vessel dissection, may cause stroke which occurs with rates ranging from 0 to 10%.
Most of the peri-procedural complications just described may arise with either the SAPIEN ™ valve or CoreValve ™ ( Table 1 ). However, because the CoreValve ™ extends into the LV with close proximity of the skirt of the valve to the membranous septum where the atrioventricular (AV) node is located, conduction abnormalities are more common with the CoreValve ™ than with the SAPIEN ™ valve. Optimal deployment of the valve can decrease the risk of this complication. Additionally, the CoreValve ™ can be repositioned during deployment and its format and larger length make stable positioning more independent of valvular calcification than the SAPIEN ™ valve.
Aortic prosthesis misplacement |
Embolization towards the aorta or left ventricle |
Deployed valve is positioned too high (towards the aorta) or too low (towards the mitral valve apparatus) |
Aortic regurgitation |
Central |
Paravalvular |
Mitral regurgitation |
Aortic prosthesis impinges on the anterior mitral leaflet |
Left ventricle asynchrony caused by right ventricular pacing |
Damage or distortion of the subvalvular mitral apparatus by delivery system |
New left ventricular wall motion abnormalities |
Acute coronary ostial occlusion |
Cardiac tamponade |
Perforation of the left or right ventricle |
Dissection or rupture of the aortic root |
Three-dimensional echocardiography
A complete understanding of the 3D anatomy of the aortic and mitral valves by interventionalists and imagers has become the foundation for accurate placement of new transcatheter devices. Although 3D TTE imaging is undergoing dramatic improvements and the development of real-time 3D colour Doppler imaging will simplify quantification of valvular regurgitation, the current TTE technology plays a limited role in TAVI. Therefore, this section will focus on the utility of 3D TEE in TAVI.
Although 3D TEE may be helpful in distinguishing between tricuspid and bicuspid valves, this is rarely an indication for 3D TEE. However, defining the aortic valve annulus is a particularly important aspect of pre-implantation TEE and an area where 3D can be extremely helpful. Piazza et al. have described the AV complex as being composed of four rings: the virtual annulus, the anatomic annulus, the sinotubular junction, and a crown-like ring from the cusps. The anatomic annulus is located where the muscular arterial aortic root joins the myocardium of the septum anteriorly and the fibrous tissue of the mitral valve posteriorly. Two-thirds of the ring abuts the septum and one-third of the ring the anterior mitral valve ( Figure 12 ). What we measure as the AV annulus is the virtual ring which is also the hinge point of the AV cusps. Because the AV typically has three equal cusps, bisecting the aortic annulus to measure the maximum diameter will typically result in an image where the immobile, calcified right coronary cusp is anterior and the commissure between the left and non-coronary cusps is posterior. As shown in Figure 13 , the orientation of the typical 2D parasternal long-axis view that displays the commissure between right and non-coronary cusps (red arrow) does not show the maximum diameter of the annulus (blue arrow). Three-dimensional TEE can be very useful in accurately sizing the annulus because aligning the short-axis view of the AV to present the true annulus allows the assessment of its circularity and the measurement of the maximum diameters ( Figure 14 ).
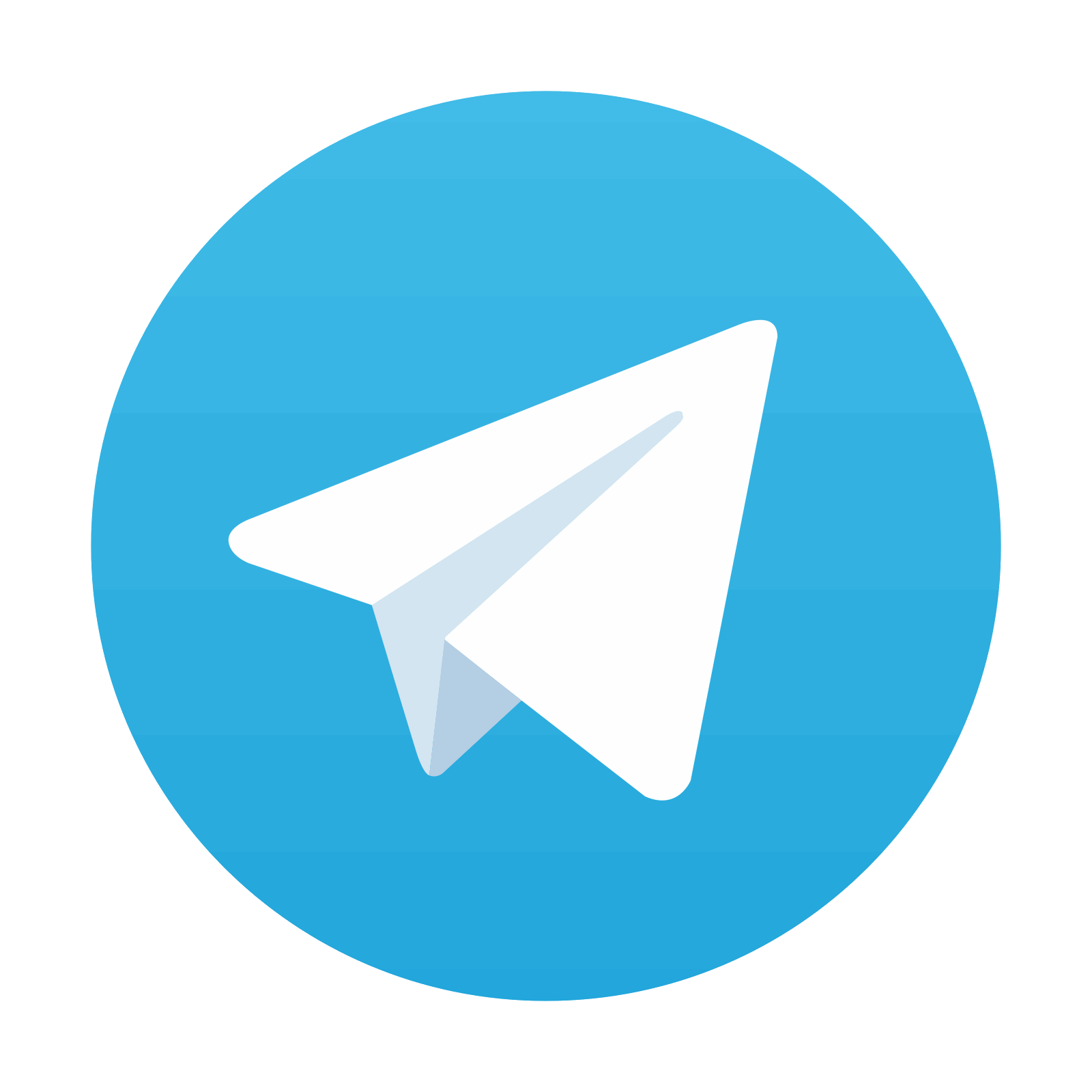
Stay updated, free articles. Join our Telegram channel

Full access? Get Clinical Tree
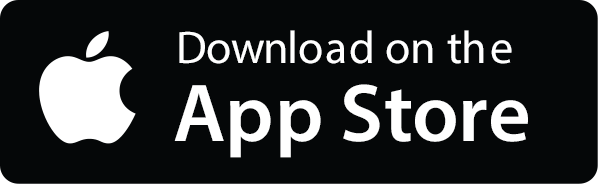
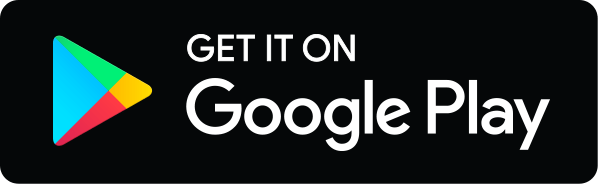