Common misconceptions and mistakes
- •
Hypoxemia is a common cause of dyspnea
- •
Hypoxemia is a common cause of exercise limitation in patients with chronic obstructive pulmonary disease (COPD)
- •
Believing that normal exercise is limited by the ability of an individual to blow off CO 2
- •
Believing that normal exercise is limited by O 2 desaturation and hypoxemia
- •
Believing that lung disease generates dyspnea solely by gas exchange/ventilation abnormalities (eg, ↑P co 2 , ↓pH, ↓P o 2 )
- •
Failing to treat exertional syncope as a medical emergency
Major mechanisms of dyspnea ( Fig. 5.1 )
- •
Dyspnea is the subjective perception of a discomfort associated with breathing
- •
Existing on a spectrum ranging from “needing to catch one’s breath” after maximal exercise, to shortness of breath, to “air hunger” (ie, a sense of suffocation accompanied by panic)
- •
- •
Dyspnea is generated (in isolation or in combination) by:
- •
Acidosis (eg, lactic acid, ketoacid, uncleared organic acids, elevated P co 2 )
- •
Respiratory system neuromechanical dissociation (especially when paired with a ventilatory stimulus)
- •
Increased cardiovascular pressure (eg, pulmonary vascular, intracardiac, aortic)
- •
Primary amygdala activation (eg, anxiety, depression, posttraumatic stress disorder [PTSD], panic disorder)
- •
- •
Respiratory system neuromechanical dissociation occurs when the brainstem respiratory center senses a mismatch between breathing effort and return on effort (ie, air movement)
- •
When efferent neurologic output regulating tidal volume (TV) and respiratory rate (RR) fails to achieve the anticipated mechanical result, as sensed by air flow and chest expansion, dyspnea ensues
- •
Extreme dyspnea occurs when neuromechanical dissociation is paired with a ventilatory stimulus (eg, hypercarbia, metabolic acidosis, hypoxemia)
- •
This is a common cause of ventilator dyssynchrony and patient discomfort in individuals with extremely abnormal pulmonary mechanics who require mechanical ventilation and permissive hypercarbia
- •
- •
- •
Dyspnea may also be generated by the cardiovascular system by three major mechanisms (angina, baroreceptor activation, and cardiogenic pulmonary edema)
- •
Angina may be perceived as dyspnea (without chest pressure or pain)
- •
Dyspnea as an anginal equivalent terminates exercise in an effort to decrease myocardial oxygen demand
- •
- •
Stretch or baroreceptor activation occurs when cardiac chambers (both right and left) and/or large vessels experience increased pressure (and thus stretch), as occurs during heart failure or increased afterload
- •
Baroreceptor activation generates a sense of dyspnea aimed at terminating exercise in an effort to lower cardiovascular pressure
- •
- •
Cardiogenic pulmonary edema may produce extreme dyspnea primarily by decreasing pulmonary compliance, leading to respiratory center neuromechanical dissociation (often paired with hypoxemia as a ventilatory stimulus)
- •
- •
Primary amygdala activation, as is seen with anxiety, depression, PTSD, and panic disorder may be perceived as dyspnea that occurs intermittently and is not associated with an exercise limitation
- •
Dyspnea generated by the cardiovascular system, the respiratory system, or acidosis is designed to terminate exercise (ie, dyspnea on exertion)
- •
Secondary activation of the amygdala occurs to varying degrees based on the magnitude of the acidosis, the neuromechanical dissociation, and/or the cardiovascular pressure increase
- •
- •
Chronic mild hypoxemia (PaO 2 55–60 mm Hg), most commonly seen with COPD, does not create significant dyspnea or limit exercise and is treated in an effort to extend life and prevent heart failure, not to reduce dyspnea or improve exercise ability (both of which are caused by impaired ventilation, not oxygenation)
- •
Chronic mild hypoxemia may be asymptomatic or associated with mild cognitive impairment/confusion
- •
- •
Acute mild hypoxemia may increase minute ventilation via the hypoxic hyperventilation reflex, causing tachypnea, but dyspnea is minimal if hypoxemia is not coupled with exercise
- •
Chronic moderate to severe hypoxemia, most commonly seen with pulmonary fibrosis, limits exercise and causes dyspnea by desaturation and hypoxemia

Physiology of exercise ( Table 5.1 )
- •
Normal exercise is limited by cardiac output and its ability to:
- •
Deliver oxygenated blood to exercising muscles
- •
Mobilize lactate, generated by skeletal muscle (anaerobic metabolism), to the liver
- •
- •
Ultimately exercise terminates with symptomatic lactic acidosis (ie, breathlessness, lightheadedness, leg heaviness, nausea)
- •
The experience of breathlessness comes from a low pH secondary to lactate (metabolic acidosis)
- •
P co 2 is compensatorily low (ie, pH 7. 32 /P co 2 32 mm Hg)
- •
- •
- •
Trained athletes owe their increased exercise capacity to:
- •
Trained skeletal muscle, which is more efficient at oxygen uptake
- •
The ability to dramatically increase their cardiac output (up to 40 L/min)
- •
The ability to tolerate a lower pH
- •
- •
Deconditioning describes the situation in which the normal limitation to exercise, namely cardiac output, oxygen delivery, and lactate mobilization, occur earlier during exercise than normal
- •
Atrophied, untrained skeletal muscle enters anaerobic metabolism more quickly
- •
Category of Exercise Limitation (and Associated Diseases) | Anticipated Derangements Cardiac Output/ Lactic Acid/pH/P co 2 | Cause of Exercise Limitation |
---|---|---|
Normal exercise limitation | ↑↑ Cardiac output, ↑↑ lactic acid, ↓ pH, ↓ P co 2 | Lactic acidosis |
Athlete exercise limitation | ↑↑↑↑ Cardiac output, ↑↑↑ lactic acid, ↓↓ pH, ↓↓ P co 2 | Lactic acidosis |
Deconditioned exercise limitation | ↑ Cardiac output, ↑ lactic acid, ↓ pH, ↓ P co 2 | Lactic acidosis |
Cardiac exercise limitation LHF (HFpEF/HFrEF/valve disease) Isolated RHF PAH | − Cardiac output, ↑↑ lactic acid, ↓ pH, ↓ P co 2 | Lactic acidosis |
Moderate ventilatory exercise limitation Obstructive disease Neuromuscular weakness | ↑ Cardiac output, ↑ lactic acid, ↓↓ pH, − P co 2 | Lactic acidosis |
Severe ventilatory exercise limitation Obstructive disease Neuromuscular weakness * | ↑ Cardiac output, − lactic acid, ↓ pH, ↑ P co 2 | Respiratory acidosis |
Exercise induced desaturation Moderate–severe diffuse parenchymal lung disease (and mimics) | ↑ Cardiac output, ↑ lactic acid, − pH, ↓↓ P co 2 | Hypoxemia |
Dyspnea—cardiovascular origin Hypertension and/or volume overload ↑ Afterload (hypertension) ↑ LVEDP (volume overload) ↑ Pulmonary vascular pressure | ↑ Cardiac output, ↑ lactic acid, ↓ pH, ↓ P co 2 | Dyspnea (baroreceptor mediated) |
Dyspnea—respiratory origin Mild obstructive disease Mild restrictive disease | ↑ Cardiac output, ↑ lactic acid, ↓ pH, ↓ P co 2 | Dyspnea (neuromechanical dissociation and ventilatory stimulus) |
Dyspnea/fatigue—metabolic acidosis | ↑ Cardiac output, ↑ lactic acid, ↓↓ pH, ↓ P co 2 | Dyspnea (metabolic acidosis) |
Dyspnea/fatigue—anemia | ↑ Cardiac output, ↑↑ lactic acid, ↓ pH, ↓ P co 2 | Dyspnea (lactic acidosis) |
* Although neuromuscular weakness causes restrictive physiology during pulmonary function testing (PFT), it also causes a ventilatory exercise limitation.
Pathophysiology of Cardiac Mediated Dyspnea on Exertion and Exercise Limitation (see Table 5.1 )
- •
Left-sided heart disease limits exercise primarily by inadequate left ventricular (LV) cardiac output secondary to either:
- •
Decreased LV systolic function (ie, cardiomyopathy)
- •
Aortic or mitral valve stenosis or regurgitation
- •
LV diastolic dysfunction
- •
Exercise-provoked diastolic dysfunction (from ischemia, hypertension, tachyarrhythmia, or hypoxemia) limits exercise, as impaired ventricular filling leads to an inadequate cardiac output (CO) relative to the needs of lactate mobilization and systemic oxygen delivery
- •
Exercise terminates early secondary to symptomatic lactic acidosis
- •
- •
- •
- •
Decreased LV cardiac output may lead to an increased LVEDP
- •
Increased left ventricular end-diastolic pressure (LVEDP) limits exercise by three major mechanisms:
- •
The biventricular heart failure reflex (triggered at an LVEDP > 15 mm Hg) further limits exercise via pulmonary hypertension and decreased right ventricular (RV) cardiac output
- •
Additionally, mild increases in LVEDP in the 15 mm Hg to 18 mm Hg range may activate cardiac baroreceptors and pulmonary capillary J receptors, producing dyspnea before pulmonary edema
- •
Higher LVEDP elevations (> 18 mm Hg) cause interstitial and alveolar edema
- •
This limits exercise by decreased compliance, increased airway resistance (submucosal edema), and by provoking respiratory system neuromechanical dissociation
- •
Pulmonary edema may also cause hypoxemia, further intensifying the sensation of dyspnea (by pairing neuromechanical dissociation with the increased ventilatory drive of hypoxemia)
- •
- •
- •
Pulmonary arterial hypertension (PAH) , as seen in chronic thromboembolic pulmonary hypertension (CTEPH) and idiopathic pulmonary arterial hypertension (IPAH), limits exercise (before right-sided heart failure) by loss of the pulmonary vascular bed cross-sectional area, which leads to increased pulmonary vascular resistance
- •
During extreme exercise, CO increases two- to fourfold
- •
The systemic circulation handles this increase in blood volume by vasodilatation of resistance arterioles
- •
The pulmonary circulation handles this increase in volume by passive distention
- •
Vascular obliteration in PAH decreases the capacity of the pulmonary vasculature to distend and accommodate the increased blood volume required by exercise
- •
Instead, as the RV attempts to increase CO to handle the increased venous return, pulmonary artery pressure rises, limiting RV CO and underfilling the LV
- •
This can lead to exertional syncope (and death), as the vasodilated systemic vasculature fails to receive enough blood return from the failing RV
- •
Exertional syncope is a “heralding event,” mandating immediate evaluation
- •
- •
- •
- •
- •
- •
Isolated right-sided heart failure (as occurs in decompensated PAH) limits exercise by inadequate RV CO, which leads to an underfilled LV, producing an inadequate CO relative to the needs of lactate mobilization and systemic oxygen delivery
- •
Leading to a symptomatic lactic acidosis with minimal exertion (New York Heart Association [NYHA] class III–IV)
- •
Pathophysiology of Pulmonary-Mediated Dyspnea on Exertion and Exercise Limitation (see Table 5.1 )
- •
Obstructive lung disease (eg, COPD/emphysema/asthma/bronchial hyperreactivity)
- •
Mild to moderate obstruction causes dyspnea and limits exercise by increasing the work of breathing to a degree that it prevents appropriate respiratory compensation
- •
Exercise may terminate prematurely (from a cardiac output standpoint) because of inappropriate respiratory compensation, where a relatively elevated P co 2 , promotes a symptomatic lactic acidosis (ie, pH 7.27/P co 2 37 mm Hg)
- •
Additionally, exercise may terminate prematurely secondary to dyspnea generated by respiratory system neuromechanical dissociation (as breathing effort increases with diminishing returns), related to increased airways resistance, dynamic hyperinflation, and intrinsic positive end expiratory pressure (PEEP)
- •
Made worse as it is paired with acidosis
- •
- •
- •
Severe obstruction may cause dyspnea and limit exercise by an inability to increase minute ventilation in response to relatively small amounts of exercise/aerobic metabolism
- •
Exercise terminates early (eg, at less than half a flight of stairs) secondary to acidosis as pH falls and P co 2 increases (producing a threatened or actual respiratory acidosis) before “anaerobic threshold” and significant lactate can be generated
- •
Dyspnea from neuromechanical dissociation related to airway resistance, dynamic hyperinflation, and intrinsic-PEEP may further impair exercise, especially when paired with acidosis
- •
- •
Very severe obstruction , when paired with an intact ventilatory drive (a.k.a. pink puffers) may produce constant dyspnea (even at rest) as the heterogeneously destroyed lung inefficiently scrubs CO 2
- •
This inefficient CO 2 removal necessitates an increased resting minute ventilation in an attempt to maintain a normal resting P co 2
- •
Ultimately leading to pulmonary cachexia because of the metabolic demands of persistently increased breathing
- •
- •
Individuals with a blunted respiratory drive (a.k.a. blue bloaters) experience less dyspnea, allowing their P co 2 to rise gradually over time (ultimately causing hypoxemia and heart failure)
- •
- •
- •
Restrictive lung disease (eg, pleural disease, neuromuscular weakness, or diffuse parenchymal lung disease [DPLD])
- •
Pleural disease alone (eg, pleural thickening, chronic pleural effusion) should not affect exercise or produce a significant restrictive defect
- •
Pleural disease with associated atelectasis (eg, pleural thickening with lung entrapment) is more likely to produce symptomatic restriction, impairing exercise by increasing the work of breathing and signaling dyspnea through neuromechanical dissociation
- •
Neuromuscular weakness causes dyspnea via neuromechanical dissociation and restrictive physiology, which limits ventilatory ability
- •
Dyspnea is worsened by supine positioning, which removes the mechanical advantage of gravity during diaphragmatic excursion
- •
Responds well to positive airway pressure (eg, bilevel positive airway pressure)
- •
- •
Progressive neuromuscular weakness ultimately leads to hypercarbic respiratory failure
- •
- •
Mild restriction from DPLD may produce dyspnea and limit exercise by neuromechanical dissociation
- •
Moderate to severe restriction from DPLD may limit exercise by causing profound O 2 desaturations (ie, PaO 2 < 50 mm Hg)
- •
These individuals experience dramatically improved exercise ability with oxygen supplementation
- •
- •
Pathophysiology of Acidosis and Anemia-Mediated Dyspnea on Exertion and Exercise Limitation (see Table 5.1 )
- •
Metabolic acidosis:
- •
Patients with an acute metabolic acidosis (eg, ketoacidosis) may present with a chief complaint of dyspnea and/or fatigue (at rest and/or with minimal exertion)
- •
Dyspnea is driven by the low pH itself
- •
- •
Patients with a chronic metabolic acidosis (eg, chronic kidney disease or end stage renal disease) may have a diminished exercise capacity, as exercise-induced lactic acid adds to the preexisting metabolic acidosis, which further lowers pH, creates dyspnea, and limits exercise by symptomatic acidosis
- •
Screen for with a serum HCO 3 and a venous blood gas (VBG) or arterial blood gas (ABG)
- •
Increasing the amount of buffer these patients receive either via oral supplementation or at dialysis can help
- •
- •
- •
Anemia :
- •
The exact mechanism by which anemia limits exercise is not agreed on, but decreased oxygen-carrying capacity and early lactate generation are probably central
- •
The exercise limitation may be described as fatigue more than dyspnea
- •
Screen for with a hemoglobin/hematocrit check
- •
Primary Activation of the Aygdala-Mediated Dyspnea (Dyspnea Without an Accompanying Exercise Limitation)
- •
Dyspnea without an exercise limitation is often caused by primary activation of the amygdala related to untreated or poorly treated depression, anxiety, PTSD, and/or panic disorder
- •
Dyspnea typically occurs intermittently, often at rest, and is worse during times of stress
- •
Individuals may complain of “forgetting to breathe” or dyspnea while speaking
- •
Similarly, inadequately treated anxiety and depression intensifies the experience of dyspnea for patients with chronic lung disease
- •
Often detectable in a panicked edge to typically routine complaints (eg, “I really felt like I was going to die after being out of breath in the shower”)
- •
- •
Dyspnea without an exercise limitation should be managed with:
- •
Reassurance:
- •
Providing reassurance may require demonstrating a normal chest x-ray, pulmonary function testing (PFT), and an echocardiogram in some cases (despite normal exercise ability)
- •
- •
Depression, anxiety, and PTSD screening
- •
Counseling
- •
Diagnostic/therapeutic selective serotonin reuptake inhibitor (SSRI) trial can provide dramatic relief/improvement
- •
- •
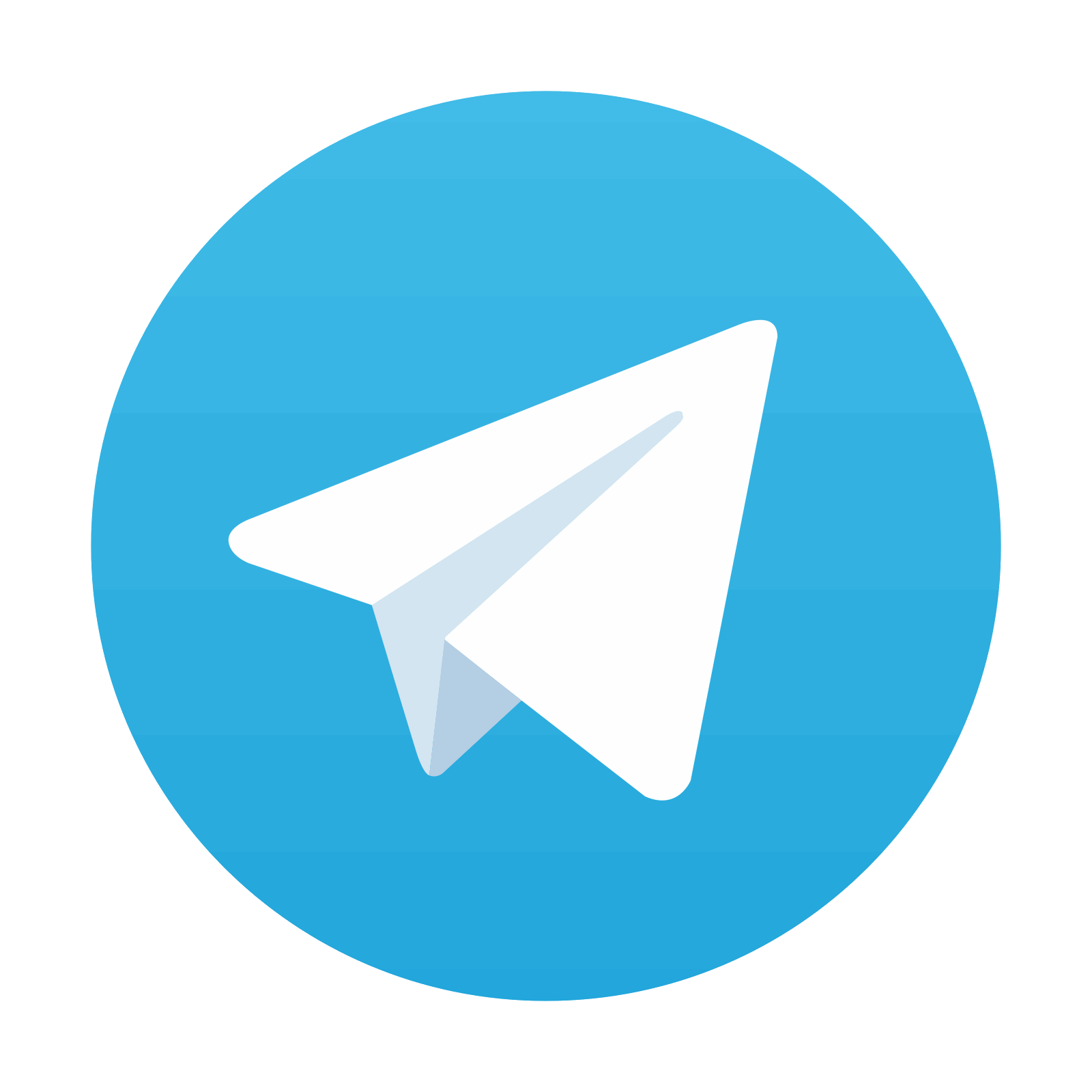
Stay updated, free articles. Join our Telegram channel

Full access? Get Clinical Tree
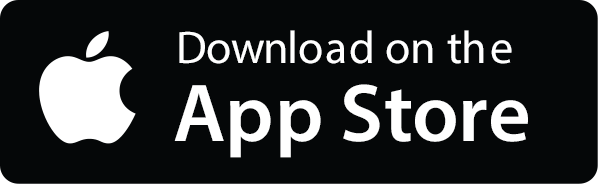
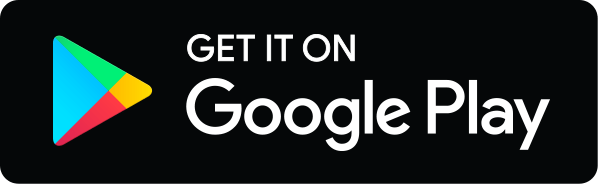