Atherosclerotic cardiovascular (CV) disease is a major cause of morbidity and mortality and is responsible for more than 50% of all the deaths in the United States and other Western countries. The best and longest known risk factor for coronary artery disease (CAD) is hypercholesterolemia. Other types of dyslipidemia, obesity, smoking, hypertension, and diabetes mellitus are among the known CV risk factors. Although most of the clinical burden of CV disease occurs in adulthood, these risk factors can develop during childhood and adolescence. In fact, there is now clear evidence that atherosclerosis begins during childhood. To reduce CV death, we need to turn our attention to preventing and correcting these risk factors before adulthood when atherosclerotic processes may have progressed too far to be reversible by therapy.
Unfortunately, the importance of preventing heart disease in the pediatric population is not well perceived by pediatricians and pediatric cardiologists. When pediatricians and pediatric cardiologists are asked about heart disease in children, they think about congenital heart defects (CHD). Although CHD is associated with the highest mortality rate of any congenital defects, only 0.4% of deaths from CV diseases are caused by CHDs in the United States. The great majority of CV death is from CAD (54%), stroke (18%), congestive heart failure (6%), and hypertension (5%) (Lauer et al, 2006) . Therefore, preventive cardiology is and should be in the pediatric domain. The primary purpose of this chapter is to raise physicians’ attention to the emerging importance of practicing medicine to prevent future CV disease (and type II diabetes) during childhood.
The strongest CV risk factors include a high concentration of low-density lipoprotein (LDL) cholesterol and triglycerides, a low concentration of high-density lipoprotein (HDL), elevated blood pressure, diabetes mellitus, cigarette smoking, and obesity. The prevalence of obesity has increased rapidly in recent decades, which is now known to be an independent risk factor for CV disease and diabetes. Obesity is often associated with other CV risk factors such as atherogenic dyslipidemia. Therefore, early detection and treatment of dyslipidemias and obesity during childhood and adolescence may help reduce CV mortality and morbidity in adulthood.
This chapter discusses the following topics in the order listed below. Hypertension, another CV risk factor, is discussed in a separate chapter ( Chapter 28 ).
- 1.
Evidence of childhood onset of atherosclerotic heart disease
- 2.
Identification of standard CV risk factors and the diagnosis of metabolic syndrome in children
- 3.
In-depth discussion of dyslipidemia in children, including lipid screening and diagnosis and treatment of dyslipidemia. Dyslipidemia includes hypercholesterolemia, hypertriglyceridemia, and low HDL cholesterol.
- 4.
Diagnosis and principles of management of childhood obesity
- 5.
Strategies for smoke cessation
- 6.
The summary table of the American Heart Association (AHA) Guidelines on practice of pediatric preventive cardiology
Childhood Onset of Coronary Artery Disease
Atherosclerotic lesions start to develop in early childhood and progress to irreversible lesions in adolescence and adulthood. The strongest evidence of childhood onset of CAD comes from the Bogalusa Heart Study (Berenson et al, 1998) and the Pathological Determinants of Atherosclerosis in Youth (PDAY) Research Group ( Strong et al, 1995 ; McGill et al, 2002 ). Autopsy studies of the aorta and coronary arteries in the youth after unexpected deaths in the Bogalusa Heart Study and in the study by the PDAY Research Group have found that atherosclerosis originates in childhood, with a rapid increase in the prevalence of coronary pathology during adolescence and young adulthood.
These studies have found the following:
- 1.
Fatty streak, the earliest lesion of the atherosclerosis, occurred by 5 to 8 years of age, and fibrous plaque, the advanced lesion, appeared in the coronary arteries in subjects in their late teens.
- 2.
Fibrous plaque was found in more than 30% of 16- to 20-year-old patients, and the prevalence of the lesion reached nearly 70% by age 26 to 39 years.
- 3.
The extent of pathological changes in the aorta and coronary arteries increased with age and so did the number of known CV risk factors that the individual had at the time of death.
Therefore, one strategy of reducing CAD in adults is to prevent or correct CV risk factors in children and adolescents.
Cardiovascular Risk Factors and Metabolic Syndrome
Box 33-1 lists major risk factors for CV disease, according to the Third Report of the National Cholesterol Education Program (NCEP) ( Circulation, 2002).
Family history of premature coronary heart disease, cerebrovascular or occlusive peripheral vascular disease (with onset before age 55 years for men and 65 years for women in parents or grandparents)
Cigarette smoking
Hypercholesterolemia
Hypertension (blood pressure >140/90 mm Hg or on antihypertensive medication)
Low levels of high-density lipoprotein (<40 mg/100 mL)
Diabetes mellitus (as a coronary heart disease risk equivalent)
Cardiovascular risk factors include a positive family history of coronary heart disease, smoking, high levels of cholesterol, hypertension, being overweight, smoking, and diabetic or prediabetic states. These risk factors are all associated with an increased prevalence and extent of atherosclerosis. Obtaining a history of these CV risk factors should be a routine process in the practice of medicine.
A family history of premature CAD in first-degree relatives (parents and siblings) has been found to be the single best predictor of CV risk for adults. For children, however, family history includes the first- and second-degree relatives (including parents, siblings, grandparents, or blood-related aunts and uncles) who have or had CAD before age 55 years for boys and before age 60 years for girls. The reason that the second-degree relatives are included in family history for children is because some children’s parents are too young to have developed clinical CAD when their children are examined.
The relationship between obesity and CAD has been a subject of some dispute for many years. However, more recent studies have shown that obesity is a risk factor for CAD independently of the standard risk factors, probably through the emerging risk factors. The emerging risk factors, which are commonly found in obese persons, include atherogenic dyslipidemia (also known as the “ lipid triad” consisting of raised level of triglycerides; small, dense LDL particles; and low levels of HDL-C), insulin resistance (hyperinsulinemia), a proinflammatory state (elevation of serum high-sensitivity C-reactive protein [CRP]), and a prothrombotic state (increased amount of plasminogen activator inhibitor-1 [PAI-1]).
The cluster of the above risk factors occurring in one person is known as “the metabolic syndrome.” In the metabolic syndrome, LCL-C levels may not be elevated, but apoprotein B (apo B) and small, dense LDL particles are elevated; the smallest particles in the LDL fraction are known to have the greatest atherogenicity (see a later section for further discussion on small, dense LDL particles). This syndrome occurs more commonly in individuals with abdominal (visceral) obesity. The exact mechanism for the role of visceral adiposity is not completely understood, but it appears to be closely related to insulin resistance. It has been assumed that obese adipose tissue releases an excess of fatty acids and cytokines that induce insulin resistance. With increasing adiposity, the lipid triad becomes more pronounced. Hispanics and South Asians seem to be particularly susceptible to the syndrome. Black men have a lower frequency of the syndrome than do white men, likely because of a lower prevalence of atherogenic dyslipidemia.
Clinically identifiable components of the metabolic syndrome for adults are listed in Box 33-2 . The presence of at least three of the risk factors is required to make the diagnosis of the metabolic syndrome in adults. Evidence has supported that waist circumference (WC) (reflecting visceral adiposity) is a better predictor of CV disease than body mass index (BMI). Although LCL-C may not be elevated, small, dense LDL particles present in this syndrome are highly atherogenic. Other components of metabolic syndrome, such as proinflammatory and prothrombotic states, are not routinely measured in clinical practice. CRP of 3 mg/L or above may be significant in adults.
Adults † | Children and Adolescents ‡ | |
---|---|---|
Obesity (WC) |
| WC ≥90th percentile or adult cut-off point |
Triglycerides | ≥150 mg/dL | ≥150 mg/dL |
HDL cholesterol |
| ≤40 mg/dL |
Hypertension | ≥130/85 mm Hg | Systolic ≥130 mm Hg/diastolic ≥85 mm Hg |
Elevated fasting glucose | ≥100 mg/dL | ≥100 mg/dL |
The presence of at least three of these abnormalities constitutes metabolic syndrome. |
† The Third Report of the National Cholesterol Education Program (NCEP) Expert Panel on Detection, Education, and Treatment of High Blood Cholesterol in Adults (Adult Treatment Panel III) final report. Circulation 106:3143-3421, 2002.
‡ Zimmet P, Alberti G, Kaufman F, et al: International Diabetes Federation Task Force on Epidemiology and Prevention of Diabetes. The metabolic syndrome in children and adolescents. Lancet 369(9579):2059-2061, 2007.
A direct association between obesity and insulin resistance also exists in children. Several definitions for use in the clinical setting for diagnosis of the metabolic syndrome in children exist. One in particular, Cook and coworkers’ (Cook et al, 2003) definition, includes triglycerides of 110 mg/dL or above and fasting glucose of 110 mg/dL or above. On the other hand, the International Diabetes Federation (2007) has proposed different cutoff points for these two, 150 mg/dL or above for triglycerides and 100 mg/dL or above for fasting glucose. The triglyceride level of 150 mg/dL is more appropriate according to more recent NHANES (National Health and Nutrition Examination Survey) data (collected between 1988 and 2002); the 90th percentile of triglyceride is much higher than 110 mg/dL (Jolliffe, 2006) . Note that the new values for triglycerides and fasting glucose proposed by International Diabetes Federation are the same as in adults (see Box 33-2 ). As in adults, the presence of at least three of the risk factors is required to make the diagnosis of the metabolic syndrome in children. It is important to note that LDL-cholesterol (LDL-C) levels may not be elevated, but this is because the LDL is mostly made up by small, dense LDL particles, which are much more atherogenic than large LDL particles. The prevalence of the metabolic syndrome in adolescents has been reported to be about 4%, but it increases to 30% to 50% in overweight adolescents ( Singh, 2006 ).
As in adults, WC is preferable to BMI for children as well, which represents abdominal (visceral or central) obesity. Ethnicity- and gender-specific WC percentiles are now available for children from the NHANES III (Fernandez et al, 2004) (see Table C-1 , Table C-2 , Table C-3 , Appendix C ). There are significant differences in WC according to ethnicity and gender. In general, Mexican American boys and girls have higher WCs than other ethnic groups. Note that the 75th percentile of WC of African American and Mexican American girls 16 and 17 years old exceed the WC values of 88 cm (35 inches) identified as the cutoff point for increased risk of obesity-related comorbidities in women.
The mainstay of the treatment of the metabolic syndrome for both adults and children is weight control through dietary intervention and promotion of an active lifestyle to achieve and maintain optimum weight, normal blood pressure, and normal lipid profile for age. The pharmacologic intervention is usually not required in children, but drugs may be used in selected high-risk patients. Each component of the syndrome present should be treated aggressively because the presence of the syndrome indicates a higher risk for CV diseases and diabetes.
Metformin (Glucophage) therapy may be effective in preventing or delaying the development of type 2 diabetes in patients with the metabolic syndrome. In conjunction with low-intensity weight control efforts, 6 months of metformin therapy has shown modest but favorable effects on the features of the syndrome, including reduction of weight, BMI, body fat mass (but not intraabdominal adipose tissue), and total cholesterol levels. It also improved fasting plasma glucose and insulin resistance (Yanovski et al, 2011) . The principles of managing obesity are presented in the section of obesity in this chapter. Treatment of dyslipidemias is presented in some detail in the section on dyslipidemia.
There appears to be a difference in susceptibility to different risk factors for different populations when they gain weight (Grundy, 2002) . For example:
- 1.
The white population of European origin appears to be more predisposed to atherogenic dyslipidemia than other populations when they gain weight.
- 2.
Blacks of African origin are prone to hypertension when they gain weight; they also appear to be susceptible to type 2 diabetes. On the other hand, they develop less atherogenic dyslipidemia than do whites with same degree of weight gain.
- 3.
Native Americans and Hispanics are especially susceptible to type 2 diabetes but less likely to develop hypertension than are blacks.
- 4.
People of South and Southeast Asia also have a high frequency of insulin resistance and type 2 diabetes. They appear more susceptible to CAD than are East Asians.
Dyslipidemia
The pathogenesis of atherosclerosis and death from the coronary atherosclerosis are importantly related to high levels of total cholesterol and LDL-C levels and low levels of HDL-cholesterol (HDL-C) levels. Multiple trials in adults have demonstrated that cholesterol reduction results in reduced angiographic progression of CAD and even modest regression in some cases. Recently, a link has been established between increased levels of triglycerides and coronary heart disease. It is timely for physicians and other health care providers to review and update their knowledge on dyslipidemia.
Quick Review
Biochemistry of Lipids and Lipoproteins
Lipids represent an essential constituent of our daily diet. Four major lipids of plasma are cholesterol, triglycerides, phospholipids, and free fatty acids. Triglycerides form an important energy source for cellular metabolism. Phospholipids are, because of their amphophilic behavior, excellent emulsifiers of fats and constitute the predominant element of all biological membranes. Cholesterol has an ambivalent nature: on the one hand, it is necessary for the stabilization of biological membrane structure and an essential precursor of hormones and bile acids in hepatic metabolism; on the other hand, a surplus of cholesterol is considered to trigger atherosclerosis.
Plasma lipids that are hydrophobic do not circulate freely but rather circulate in the form of lipid-protein macromolecular complexes known as lipoproteins. The nonpolar lipids (cholesterol esters and triglycerides) are present in the lipoprotein core surrounded by a monolayer composed of specific proteins (apos) and the polar lipids (unesterified or free cholesterol and phospholipids). This monolayer allows the lipoprotein to remain miscible in plasma. Lipoproteins function as transport vehicles for water-insoluble lipid fractions and lead them to their sites of metabolism or deposition. Free fatty acids are bound to albumin.
The plasma lipoproteins have been classified into four major groups based on their density: chylomicrons, very low-density lipoprotein (VLDL), LDL, and HDL. Most cholesterol in plasma is transported by LDL (∼65%) with the remainder on HDL (25%) and VLDL (10%). Triglycerides (TGs) are transported primarily by chylomicron and VLDL.
The lipoproteins can be divided into two by the type of apos in the lipoprotein. Apos serve as enzymatic cofactors and recognition elements in binding to specific receptors.
- •
The apo B lipoproteins are atherogenic and include chylomicrons, LVDL, LDL, and lipoprotein(a) [Lp(a)].
- •
The apo A-I–containing lipoproteins are associated with reduced CV disease and include HDL and their subfractions.
Small, dense LDL. Recent studies have shown that it is the size of the LDL particles, not the total concentration of LDL, that is more important in the pathogenesis of CAD. Small, dense LDL particles are expected to be better able to penetrate through the intima of the coronary arteries to deposit in the subendothelial space, where plaque forms. They are also more readily oxidized; only oxidized LDL can enter the macrophages in the lining of the arteries and form cholesterol-rich plaques. The small, dense LDL phenotype usually occurs associated with elevated TG levels (>140 mg/dL) and a decreased HDL level (<40 mg/dL in men; <50 mg/dL in women). These are typical features of obesity, the metabolic syndrome, insulin resistance, and type 2 diabetes mellitus.
Lipid and Lipoprotein Metabolism
A review of simplified lipid and lipoprotein metabolism is presented in Figure 33-1 for readers who wish to quickly refresh the metabolism of lipid and lipoproteins before reading clinical aspects of dyslipidemia. The figure legend provides a shorter summary of the topic.

Exogenous Pathway
After ingestion of fat-containing foods, TGs and cholesterol are absorbed into intestinal cells as fatty acids and free cholesterol. Within the intestinal wall, free fatty acids and cholesterol are reesterified to form TGs and cholesteryl ester, respectively. These lipids are then combined with phospholipids and apos A-I, A-IV, and B-48 to form TG-rich chylomicron particles. Apo B-48 is an obligatory protein.
Chylomicrons rapidly enter plasma via the thoracic duct. In the circulation, chylomicrons acquire additional apos (mainly apo E and several forms of apo C). TG-rich chylomicrons are hydrolyzed by the enzyme lipoprotein lipase (LPL) at the capillary endothelium, leaving a smaller and denser, remnant particle because they have lost much of their TGs. (The free fatty acid products of this hydrolysis are transferred primarily to adipose tissues for storage as TGs or to muscle for β-oxidation.) This particle is called chylomicron remnant and is rich in cholesterol and has gained apo E from HDL (and lost apo A and apo C to HDL).
These remnants are bound and internalized in part via hepatic membrane receptors specific for apo E on the particle. By this mechanism, dietary cholesterol is delivered to the liver, where it plays a role in the regulation of hepatic cholesterol metabolism. In normal persons, chylomicron and chylomicron remnants are very short lived in the circulation, and none of them exists in the plasma after a 12-hour fast. Chylomicron and chylomicron remnants may be atherogenic. Delayed clearance of chylomicron occurs in inherited deficiency of LPL or its activator, apo C-II (type I hypertriglyceridemia).
Endogenous Pathway
Triglycerides synthesized in the liver are packaged with cholesteryl esters and apos B-100, C, and E and then secreted as VLDL. The synthesis of VLDL by the liver is increased by excess carbohydrate, alcohol, or caloric intake and is decreased in the fasting state.
In the capillary beds, TG in the core of the VLDL is hydrolyzed by LPL, with a cofactor apo C-II, to produce a smaller denser particle called VLDL remnant or intermediate-density lipoprotein (IDL), which is analogous to chylomicron remnant. (The surface components, except for apos B-100 and E, are transferred to HDL.) Free fatty acids generated by hydrolysis of TG are delivered to adipose tissue and muscle. Compared with VLDL, VLDL remnants have more cholesterol ester and less TG.
Some of these remnant particles (IDLs) are taken up by the hepatic receptors specific for apo E, and some others undergo conversion to LDL by hepatic TG lipase. Elevation of VLDL remnants may predispose the patient to premature CAD and peripheral artery disease (characteristically seen in Frederickson’s type III hyperlipoproteinemia).
Low-density lipoprotein is usually formed by means of enhanced conversion of VLDL remnants (see Fig. 33-1 ) or by direct hepatic production of apo B–containing lipoproteins. LDLs are almost entirely made up of cholesteryl esters and apo B-100.
The content of cholesterol ester in the LDL particle may vary as much as 40%. LDL particles that contain lower amounts of cholesterol ester are known as small, dense LDL particles. Patients with increased amounts of small, dense LDL particles are at increased risk for CAD. Small, dense LDL is commonly associated with male gender, diabetes, low HDL-C levels, high TG levels, and familial combined hyperlipidemia (FCH).
Low-density lipoproteins are transported to peripheral cells or liver cells. Apo B-100, the only protein found in LDL, is recognized by a high-affinity LDL receptor on the surfaces of hepatic and certain nonhepatic cells, where LDLs are internalized into the cells. By this mechanism, LDL particles can deliver cholesterol to extrahepatic tissues for use in membrane or steroid hormone synthesis. LDL receptor expression by the liver is a major regulator of plasma LDL-C levels. LDL particles have a half-life of 3 to 4 days.
The liver and small intestine secrete HDL as nascent discoid particles composed primarily of phospholipids and apolipoproteins (nascent HDL). (Whereas nascent HDL particles secreted by the intestine are rich in apo A-I and apo A-IV, those secreted by the liver contain predominantly apo A-I and apo A-II.) Nascent lipid-poor apo A-I accepts free (unesterified) cholesterol from tissues, and the free cholesterol transferred to the surface of HDL is esterified by the action of enzyme lecithin–cholesterol acyltransferase (LCAT).
High-density lipoprotein cholesteryl esters may be directly transferred to and selectively taken up by the liver via a hepatic HDL receptor called scavenger receptor class BI (SR-BI). Alternatively, HDL cholesteryl esters may transfer from HDL to VLDL and LDL by the cholesteryl ester transfer protein (CETP), after which it may be taken up by the liver or redistributed to peripheral tissues. Thus, HDLs have two pathways by which they return tissue-derived cholesterol to the liver. The removal of cholesterol from cells by HDL for ultimate disposal in the liver has been termed reverse cholesterol transport. These reactions may explain how HDL and apo A-I can protect against the development of atherosclerosis.
Among the several subtypes of HDL particles, HDL 2 and HDL 3 are clinically important. HDL 2 is closely associated with protection against premature atherosclerosis. Alcohol consumption predominantly increases the HDL 3 subfraction. Lower levels of both subfractions are associated with male gender, hypertriglyceridemia, diabetes mellitus, obesity, uremia, smoking, and the use of androgens and progesterones. Estrogen raises HDL levels.
Cholesterol returning to the liver is converted into bile acids by the enzymatic hydroxylation of cholesterol, or cholesterol and phospholipids are excreted directly into the bile. A large portion of secreted bile acid is reabsorbed in the enterohepatic circulation and recycled. Bile acid sequestrants reduce the reabsorption of secreted bile acids, eventually reducing serum cholesterol levels by increasing the conversion of hepatic cholesterol to bile acids, thus reducing the cholesterol content of the hepatocytes. The reduced hepatic cholesterol stimulates the production of surface receptors for LDL-C, clearing more LDL from the serum.
The hepatic and extrahepatic cells can control their own cholesterol content through a feedback control system. In conditions of cellular cholesterol excess, the cell can (1) suppress endogenous cholesterol production by inhibiting the activity of 3-hydroxy-3-methylglutaryl coenzyme A (HMG-CoA) reductase, the rate-limiting enzyme in cholesterol synthesis; (2) decrease its input of cholesterol by suppressing the production of LDL surface receptors through a feedback mechanism; and (3) promote the removal of cholesterol by increasing its movement to the plasma membrane for efflux. The “statins” work through the first mechanism to reduce endogenous cholesterol production.
Diagnosis of Dyslipidemia
The diagnosis of dyslipidemia is made by measuring blood lipid, lipoproteins, or apolipoprotein factors. If any of the measurements are abnormal, the diagnosis of a specific dyslipidemia is made, such as elevated total cholesterol, LDL-C, apo B, non–HDL-C, and TGs or a lower than normal level of HDL-C.
Measurement of Lipid and Lipoproteins
A lipoprotein analysis is obtained after an overnight fast of 12 hours. The routine lipid profile typically includes total cholesterol, HDL-C, LDL-C, and TGs. An extended profile may also include VLDL cholesterol (VLDL-C), non–HDL-C, and the ratio of total cholesterol to HDL.
The LDL level is usually estimated by the Friedewald formula:
LDL = Total cholesterol − HDL − ( TG / 5 )
This formula is not accurate if the child is not fasting, if the TG level is above 400 mg/100 mL, or if chylomicrons or dysbetalipoproteinemia (type III hyperlipoproteinemia) is present. Methods are currently available to measure LDL-C directly, which allow LDL-C determination on specimens with the TG level above 400 mg/dL. Direct LDL-C measurement does not require a fasting specimen.
The following derivatives of lipid profile may be useful in the assessment of risks for CV disease.
- 1.
Non–HDL-C cholesterol: Serum non–HDL-C (Total cholesterol − HDL-C) is considered a better screening tool than LDL-C for the assessment of CAD risk because it includes all classes of atherogenic (apo B–containing) lipoproteins; it includes VLDL-C, IDLs, LDL-C, and lipoprotein (a) (or Lp[a]). It was found in adults that increased level of non‐HDL-C by 1 mg/dL increases the risk of death from CV disease by 5%.
- 2.
TC to HDL-C ratio: The total cholesterol to HDL-C (TC to HDL-C) ratio is a useful parameter for assessing risk for CV disease. The usual TC to HDL-C ratio in children is approximately 3 (based on TC of 150 mg/dL and an HDL-C of 50 mg/dL). According to the Framingham study, the ratio for average risk was 5.0 for men and 4.2 for women. The higher the ratio, the higher the risk of developing CV disease. The ratio of 3.4 halves the risk of developing CV disease for both men and women.
- 3.
Small, dense LDL particles: In recent years, small, dense LDL particles have been shown to be more important than the total LDL levels in CAD. The size of LDL particles is not routinely measured because the presence of this phenotype is predictable. It occurs in association with elevated TG levels (>140 mg/dL) and a decreased HDL level (<40 mg/dL in men; <50 mg/dL in women). Although not routinely measured, small, dense LDL can be measured directly by various methods in commercial laboratories (including Berkeley HeartLab, Inc. [ www.bhlinc.com ]; Atherotec, Inc. [ www.thevaptest.com ]; and LipoScience, Inc. [ www.lipoprofile.com ]).
Normal Levels of Lipids and Lipoproteins
Table 33-1 shows normal, borderline, and abnormal levels of lipid and lipoprotein levels in children, and Table 33-2 shows those values for young adults. Note that to convert total cholesterol, LDL-C, and HDL-C levels in milligrams per deciliter to millimoles per liter, one divides the mg/dL number by a factor of 38.67. To convert TGs in milligrams per deciliter to millimoles per liter, one divides the mg/dL number by a factor of 88.5.
Category | Low | Acceptable | Borderline | High |
---|---|---|---|---|
Total cholesterol | — | <170 | 170–199 | ≥200 |
LDL cholesterol | — | <110 | 110–129 | ≥130 |
Non-HDL-C | — | <120 | 120–144 | ≥145 |
Triglycerides: 0–9 years | — | <75 | 75–99 | ≥100 |
10–19 years | — | <90 | 90–129 | ≥130 |
HDL cholesterol | <40 | >45 | 40–45 | — |
Apolipoprotein A-1 | <115 | >120 | 115–120 | — |
Apolipoprotein B | — | <90 | 90–109 | ≥110 |
Category | Low | Borderline Low | Acceptable | Borderline High | High |
---|---|---|---|---|---|
Total cholesterol | — | — | <190 | 190–224 | ≥225 |
LDL cholesterol | — | — | <120 | 120–159 | ≥160 |
Non–HDL cholesterol | — | — | <150 | 150–189 | ≥190 |
Triglycerides | — | — | <1155 | 115–149 | ≥150 |
HDL cholesterol | <40 | 40–44 | >45 | — | — |
Classification of Dyslipidemia
Dyslipidemias were traditionally classified by patterns of elevation in lipids and lipoproteins (Fredrickson phenotypes), but a more practical system is to classify them as primary (genetic) or secondary dyslipidemia.
- 1.
Primary dyslipidemia is caused by single or multiple gene mutations that result in either overproduction or defective clearance of TGs and LDL-C or in underproduction or excessive clearance of HDL-C (see later discussion). This entity is much less common than secondary dyslipidemia.
- 2.
Secondary dyslipidemia is caused by associated diseases or conditions and is much more common than primary dyslipidemia (see further discussion later). The majority of the cases found during screening are secondary forms.
Screening of all family members is recommended to determine whether the disorder is a familial one. Family screening is important not only to detect dyslipidemia in other members of the family but also to emphasize the need for all family members to change their eating patterns. Young patients with elevated LDL levels are more likely to have a familial disorder of LDL metabolism. Secondary dyslipidemia can occur in any age group.
Secondary Dyslipidemia
Box 33-3 lists the causes of secondary dyslipidemia. The most common cause of pediatric dyslipidemia is obesity. Medications such as oral contraceptives, isotretinoin (Accutane), anabolic steroids, diuretics, beta-blockers, and estrogens are uncommon causes of dyslipidemia. Medical conditions that include hypothyroidism, renal failure, nephrotic syndrome, and alcohol usage are less common causes of secondary dyslipidemia. Evidence of accelerated atherosclerosis from secondary causes of dyslipidemia is as impressive as that of primary causes.
Metabolic | Metabolic syndrome, diabetes, lipodystrophies, glycogen storage disorders |
Renal disease | Chronic renal failure, nephrotic syndrome, glomerulonephritis, hemolytic uremic syndrome |
Hepatic | Biliary atresia, cirrhosis |
Hormonal | Estrogen, progesterone, growth hormone, hypothyroidism, corticosteroids |
Lifestyle | Obesity, physical inactivity, diets rich in fat and saturated fat, alcohol intake |
Medications | Isotretinoin (Accutane), certain oral contraceptives, anabolic steroids, thiazide diuretics, beta-adrenergic blockers, anticonvulsants, glucocorticoids, estrogen, testosterone, immunosuppressive agents (cyclosporine), antiviral agents (HIV protease inhibitor) |
Others | Kawasaki’s disease, anorexia nervosa, post–solid organ transplantation, childhood cancer survivor, progeria, idiopathic hypercalcemia, Klinefelter syndrome, Werner syndrome |
Most secondary causes of dyslipidemia raise TGs and often lower HDL-C levels, with the exception of estrogen excess with which HDL-C levels increase.
Each child with dyslipidemia should have certain blood tests to help rule out secondary causes of dyslipidemia. The blood tests should include fasting blood glucose and tests of kidney, liver, and thyroid function. When the diagnosis of secondary dyslipidemia is made, one should treat the associated disorder producing the dyslipidemia first, such as diabetes, obesity, or nephritic syndrome, and then treat dyslipidemia using the same guidelines as in primary dyslipidemia.
Selected Primary Dyslipidemias
Primary or inherited dyslipidemia is less commonly found in the screening process. Major primary dyslipidemias found in children include familial hypercholesterolemia (FH), FCH, familial hypertriglyceridemia, and several others. Table 33-3 summarizes changes in the lipid profile seen with primary lipid disorders.
Primary Lipid Disorders | LDL | VLDL | TG | HDL | Others |
---|---|---|---|---|---|
Familial hypercholesterolemia: homozygous | ↑↑ | ||||
heterozygous | ↑ | ||||
FCH: type IIa | ↑ | ||||
Type IV | ↑ | ↑ | |||
Type IIb | ↑ | ↑ | ↑ | ||
Types IIb and IV | ↓ | ||||
Familial hypertriglyceridemia (200–1000 mg/dL) | ↑ | ↑ | |||
Severe hypertriglyceridemia (>1000 mg/dL) | ↑ | ↑↑ | ↑ Chylomicron | ||
Familial hypoalphalipoproteinemia | ↓ | ||||
Dysbetalipoproteinemia (TC: 250–500 mg/dL; TGL: 50–600 mg/dL) | ↑ IDL ↑ Chylomicron remnant |
Familial hypercholesterolemia. FH is autosomal dominant condition caused by a lack of or a reduction in LDL receptors. Whereas heterozygotes have about a 50% reduction in LDL receptors, homozygotes have little or no receptor activity. FH results in extreme elevation of LDL-C that may distinguish the condition from other primary and most secondary causes of dyslipidemia. Genetic testing remains the criterion standard for diagnosis of the condition, although it is not widely used.
1 . Heterozygous disorder. Familial hypercholesterolemia heterozygous disorder is fairly common, occurring in one of every 500 people. It is inherited in an autosomal dominant mode. An evaluation of family members is important in the diagnosis of this condition. In this condition, 50% of siblings and one parent have elevated total and LCL-C levels, but unaffected first-degree relatives have completely normal levels.
In heterozygotes, total cholesterol and LDL-C levels are two to three times higher than normal, present at birth or early in life. Their total cholesterol levels are most often above 240 mg/100 mL, with an average of 300 mg/dL, and their LDL-C levels are above 160 mg/100 mL, with an average of 240 mg/dL. HDL-C is reduced, and TG levels are usually normal. The presence of xanthomas of the extensor tendon in the parents of such children almost confirms the diagnosis. A heterozygous child or adolescent has normal physical findings. Tendon xanthomas are rarely found before the age of 10 years; they develop in the second decade, primarily in the Achilles tendons and extensor tendons of the hands, in only 10% to 15% of patients. These patients are likely to develop premature CV disease; rarely, angina pectoris develops in the late teenage years.
Treatment of heterozygotes includes a diet low in saturated fat and cholesterol and high in water-soluble fibers. Bile acid sequestrants are safe and moderately effective but difficult to tolerate over the long term because of their gritty texture and gastrointestinal (GI) complaints. Statins are safe, effective, and well tolerated and are considered the drug of choice (see later section). The addition to the statin of a bile acid sequestrant or a cholesterol absorption inhibitor (CAI) is often necessary to achieve LDL-C goals. Niacin is generally not used to treat FH heterozygous children.
2 . Homozygous disorder. Homozygosity occurs in children who inherit a mutant allele for FH from both parents; it occurs in about one in a million children. The total cholesterol and LDL-C levels in these children are five to six times greater than normal. Such children have cholesterol levels that average 700 mg/dL but may reach higher than 1000 mg/dL. Clinical signs such as planar xanthomas, which are flat, orange-colored skin lesions, may be present by the age of 5 years in the webbing of the hands and over the elbows and buttocks. Tendon xanthomas, arcus corneae, and clinically significant coronary heart disease are often present by the age of 10 years. The generalized atherosclerosis often affects the aortic valve, with resulting aortic stenosis.
Familial hypercholesterolemia homozygous children respond somewhat to high doses of potent statins and to niacin. CAIs also lowers LDL-C in FH homozygotes, especially in combination with a more potent statin. However, most FH homozygotes invariably require LDL apheresis (with extracorporeal affinity LDL absorption column and plasma reinfusion) every 2 weeks to lower LDL to a range that is less atherogenic. The Liposorber system is an example, which selectively binds apo B–containing lipoproteins (LDL, Lp[a], and VLDL).
Familial combined hyperlipidemia. FCH is an autosomal dominant disorder seen three times more frequently than FH. It occurs in one of every 200 to 300 people. It is characterized by variable lipid phenotypic expression: elevated LDL level alone, elevated LDL with hypertriglyceridemia, or normal LDL with hypertriglyceridemia. Clinically, it may be difficult to separate this entity from FH. The diagnosis of FCH is suspected when a first-degree family member (often a parent or sibling) has a different lipoprotein phenotype than the proband. Levels of total and LDL-C are somewhat lower than in patients with FH, and LDL-C levels fluctuate from time to time, with TG levels fluctuating in the opposite direction. These children usually have plasma total cholesterol levels between 190 and 220 mg/dL. The LDL-C level is usually normal or only mildly elevated. In FCH, most patients lack tendon xanthomas, and extreme hyperlipidemia is absent in childhood. It may occur in children of survivors of myocardial infarction. Their phenotypes often have other characteristics such as hyperinsulinemia, glucose intolerance, hypertension, and visceral obesity. The combined expression of three or more of these traits constitutes the metabolic syndrome (see earlier section).
A cornerstone of management should be aimed at a fat- and cholesterol-restricted diet and a simple carbohydrate-restricted diet (low glycemic index foods) together with attention to other CV lifestyle changes (control of overweight and regular aerobic exercise). Low glycemic index foods are important in reducing carbohydrate-induced hypertriglyceridemia. The statins are the most effective in lowering LDL-C and the total number of atherogenic small, dense LDL particles. Fibric acid and niacin, which are effective in adults, are not ordinarily used in pediatric patients. Drug therapy is reasonable in patients with persistently elevated TGs levels (>350 mg/dL), aimed primarily at preventing an episode of pancreatitis. Metformin has been used to treat obese hyperinsulinemic adolescents with the metabolic syndrome. Metformin may enhance insulin sensitivity and reduce fasting blood glucose, insulin levels, plasma lipids, free fatty acids, and leptin.
Familial hypertriglyceridemia. In children, this autosomal dominant disorder is caused by LPL deficiency, resulting in hepatic overproduction of VLDL-C. TG levels are typically increased. Cholesterol levels are not increased. Eruptive xanthomas and lipemia retinalis (a creamy appearance of the retinal veins and arteries caused by a high concentration of lipids in the blood) can also be found. Metabolic consequences of hypertriglyceridemia include (1) a lowering of HDL-C; (2) production of smaller, denser LDL particles with more atherogenicity; and (3) a hypercoagulable state.
Treatment is based first on a diet very low in fat and simple sugar and lifestyle modification, including increasing exercise, withdrawing hormones (estrogen, progesterone), and limiting alcohol intake. When the level of TGs is between 200 and 500 mg/dL, the goal of the treatment is to reduce CAD. When TG levels reach 500 to 1000 mg/dL, pancreatitis is a major concern. One may consider using drugs such as fibrate or niacin (see later sections for the use of these antilipidemic agents). If pancreatitis develops, which is usually characterized by severe abdominal pain, and elevated plasma levels of amylase and lipase, the patient should be admitted to a hospital for care with intravenous fluid.
Dysbetalipoproteinemia (type III hyperlipoproteinemia). This familial disorder is a rare genetic disorder caused by a defect in apo E, which results in increased accumulation of chylomicron remnants and VLDL remnants. This autosomal recessive disorder is characterized by elevation of both cholesterol and TG equally to greater than 300 mg/dL, but this disorder is not usually seen in childhood. Clinical manifestations may include palmar xanthoma. Patients with this disorder have a moderately increased risk of CV disease. A low-fat diet, a low glycemic index diet, and drug treatment (fibric acid or statin) are very effective.
Familial hypoalphalipoproteinemia (low HDL syndrome). This condition is associated with autosomal dominant inheritance. The underlying mechanism is decreased concentration of apo A-I and apo A-II and absent apo C-III. This condition is associated with isolated low HDL-C levels and mild to moderately increased risk of premature CAD. In Tangiers disease, HDL-C is nearly absent (with markedly enlarged yellow tonsils).
Although specific drug therapy is not routinely recommended in pediatric age groups, a low-carbohydrate and low-fat diet is indicated in children with inherited disorders of HDL metabolism. Exercise and weight loss are also helpful. The most effective way to reduce CV risk in these patients is to maintain low LDL-C levels.
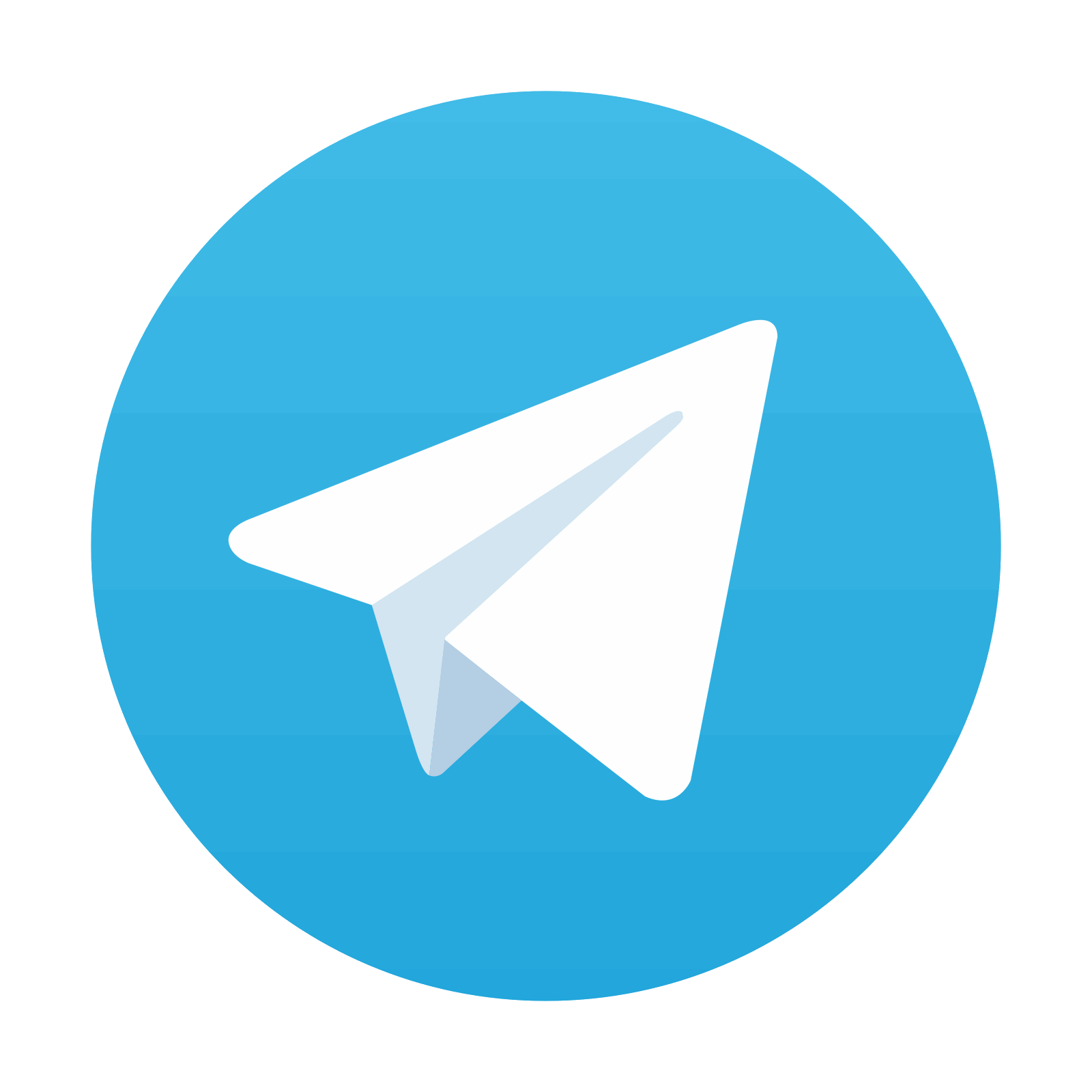
Stay updated, free articles. Join our Telegram channel

Full access? Get Clinical Tree
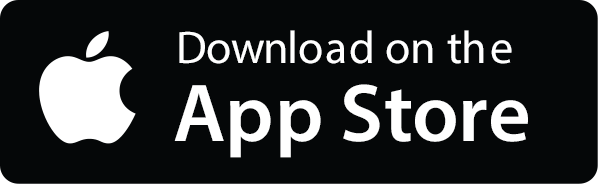
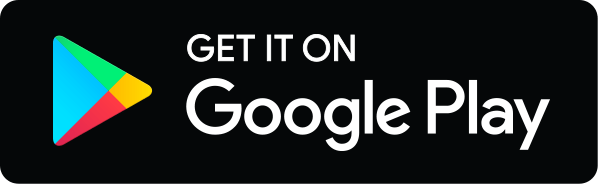