Fig. 1
Activities of endothelial cells. Vasomotor and mitogenic balance is provided by molecules secreted by endothelial cells and vascular smooth muscle cell. These cells have several paracrine functions by producing and secreting several molecules including vasoactive, inflammatory, vasculoprotective, angiogenic, thrombotic and antithrombotic
Table 1
Molecules which are produced and secreted by endothelial cells
Regulation of vascular tonicity | |
Vasodilatation | Nitric oxide |
Prostacyclin | |
Endothelium‐derived hyperpolarizing factors | |
Adenosine | |
Vasoconstriction | Endothelin-1 |
Angiotensin II | |
Thromboxane A2 | |
Reactive oxygen species | |
Balancing of blood fluidity and thrombosis | |
Coagulation | Heparin cofactor 2 |
Factor V | |
Protein S | |
Protein C | |
Thrombomodulin | |
Tissue factor | |
von Willebrand factor | |
Fibrinolysis | Tissue plasminogen activator |
Prostaglandins | |
Plasminogen activator inhibitor type 1 | |
Urokinase | |
Vascular inflammatory and immunological process control | |
Cytokines | Interleukin -1 |
Interleukin-6 | |
Interleukin-8 | |
Monocyte chemoattractant protein-1 | |
Adhesion molecules | Transforming growth factor |
Tumor necrosis factor | |
Vascular cell adhesion protein 1 | |
Intercellular adhesion molecule 1 | |
Selectins | |
Growth factors | Basic fibroblast growth factor |
Insulin like growth factor | |
Platelet derived growth factor | |
Transforming growth factor |
2.1 Regulation of Vascular Tonicity
Vascular tonicity is regulated by atrial natriuretic peptide, eicosanoids, adrenal steroids, sodium, and water excretion and by the control of neurologic, kallikrein- kinin, reno-medullary endothelial systems. Molecules such as endothelin-1 (ET-1), angiotensin II (AT-II), thromboxane A2 (TXA2), and reactive oxygen species (ROS) are known as endothelium‐derived relaxing factors, whereas NO and prostacyclin are known as endothelium‐derived hyperpolarizing factors (EDHFs) (Dawes et al. 2008). In healthy endothelial tissues, a balance between endothelium‐derived relaxing factors (EDRFs) and endothelium‐derived contracting factors (EDCFs) is maintained. Disturbance of this balance causes endothelial dysfunction (Cahill and Redmond 2016).
Nitric Oxide (NO)
Vascular smooth muscle cells release: Endothelium-derived NO, the known most powerful vasodilator which activates the soluble guanylate cyclase. Soluble Guanylate cyclase (sGS) enzyme converts GTP to cyclic GMP (cGMP) which activates protein kinase G that leads decreases in the cytosolic calcium concentrations. NO can also affect cellular activity, independently of sGC activation, by the stimulation of the endoplasmic reticulum calcium ATPase, reducing the intracellular calcium concentration and cause relaxation of the smooth muscle. The release of inflammation, vascular cell proliferation, platelet adhesion, and tissue factor are inhibited by NO (Laher 2014).
NO is synthesized from an L-arginine by the enzyme nitric oxide synthases (NOS) as a free radical (Fig. 2). There are three distinct genes encoding NOS isozymes; neuronal NOS (nNOS or NOS-1), cytokine-inducible NOS (iNOS or NOS-2) and endothelial NOS (eNOS or NOS-3) (Melikian et al. 2009) (Table 2). The production of NO from L-arginine by NOS requires the presence of various co-factors including tetrahydrobiopterin, flavin adenine dinucleotide, flavin mononucleotide, calmodulin (calcium binding protein) and iron protoporphyrin (Palmer et al. 1988). nNOS is expressed in the central and peripheral nervous systems, in cardiac and skeletal myocytes, smooth muscles and ECs. NO produced in the nervous systems by nNOS is associated with the regulation of neuronal excitability and synaptic plasticity, memory and learning processes. It has been suggested that the expression of vascular nNOS is also upregulated by stimulation with AT-II and platelet-derived growth factor (Dawson et al. 1991). iNOS is minimal under physiological conditions and is calcium insensitive. When iNOS is stimulated, it continuously produces NO. Induction of iNOS occurs mainly during infection and chronic inflammation. iNOS is expressed in vascular smooth muscle cells following exposure to pro-inflammatory cytokines. It is reported that inflammation-induced iNOS production in the endothelium is related to the vascular dysfunction by limiting the availability of BH4 for e NOS (Lowenstein and Padalko 2004; Gunnett et al. 2005). eNOS is the major isoform for the regulation of vascular function. The activity of eNOS and the production of NO can be stimulated by shear stress, acetylcholine, bradykinin and histamine by both calcium-dependent and independent ways (Laher 2014). Acetylcholine, bradykinin and histamine bind to specific receptors on the endothelial cell membrane and increase the intracellular concentration of calcium. In a calcium-independent manner, the activation of eNOS is due to the post-translational modification of the enzyme including phosphorylation by NOS kinase and dephosphorylation by phosphatases (Kellogg et al. 2005; Bae et al. 2003). Phosphorylation alters the activity of eNOS, and different sites of phosphorylation can have an opposing effect.The endogenous competitive inhibitor for eNOS is called asymmetric dimethyl arginine (ADMA) (Arora et al. 2013; Zhao et al. 2014). The inhibition of eNOS is correlated with plasma ADMA levels, and plasma ADMA levels are inversely related to endothelium-dependent vasodilation (Vestweber 2012). The acute and chronic rise in the shear stress of blood up-regulates the expression and the activity of eNOS, and thus the release of EDRF/NO (Kolluru et al. 2010; Michel and Vanhoutte 2010). AT-II by binding to its receptor produces bradykinins which stimulate eNOS consequently increases the formation of NO (Yayama et al. 2006).


Fig. 2
Nitric oxide (NO) is synthesized by endothelial nitric oxidase synthase (eNOS) can activate soluble guanylate cyclase. cGMP is produced. Vasodilatation occurs, and angiogenesis is inhibited. NO inhibits both platelet and monocyte functions (Şekil Türkçe)
Table 2
The forms of NO synthase
Gene | Main Localization (s) | Functions | Stimulation | |
---|---|---|---|---|
Neuronal NOS (nNOS;NOS-1) (calcium-dependent) | Chromosome 12 | Central and peripheral nervous systems | Regulation of neuronal excitability and synaptic plasticity, | Angiotensin II |
Platelet-derived growth factor | ||||
Cardiac and skeletal myocytes Smooth muscle and endothelial cells | Memory and learning processes | |||
Inducible NOS (iNOS;NOS-2) (calcium-independent) | Chromosome 17 | Immune system | Participation in anti-microbial and anti-tumor activities (e.g.oxidative burst of macrophages) | Proinflammatory cytokines (Interleukin-1, Tumor necrosis factor α, Interferon γ) |
Cardiovascular system | ||||
Smooth muscle cells | ||||
Endothelial NOS (eNOS; NOS-3) (calcium–dependent) | Chromosome 7 | Endothelium | Regulating vascular function | Shear stress |
Acetylcholine | ||||
Bradykinin | ||||
Histamine |
Additionally, the products of the metabolism of NO are nitrite and nitrate which act as a reservoir of NO. Under certain conditions, several enzymes, such as xanthine oxidoreductase, mitochondrial cytochrome oxidase, aldehyde dehydrogenase 2 and cytochrome P450 reductase, catalyze the reduction of nitrite or nitrate to NO (Weitzberg et al. 2010).
Prostacyclin
Another vasodilator molecule is prostacyclin (also called prostaglandin I2 or PGI2) which is a prostaglandin that belongs to the eicosanoid family of lipid molecules. Prostacyclin is produced in ECs in response to inflammatory mediators, including interleukin (IL)-1 and platelet-derived and epidermal growth factors from prostaglandin H2 (PGH2) by the action of the enzyme prostacyclin synthase (Fig. 3). Like NO, it inhibits platelet activation and act as an effective vasodilator (Cahill and Redmond 2016; Siti et al. 2015). PGI2 is released by healthy ECs and performs its function via paracrine signaling that involves G protein-coupled receptors on both ECs and platelets. PGI2 binds to endothelial prostacyclin receptors and raise cAMP levels in the cytosol. cAMP activates protein kinase A (PKA) which promotes the dephosphorylation of the myosin light chain kinase. Dephosphorylation of the enzyme results in the inhibition of myosinlight-chainkinase. This leads relaxation of the smooth muscle relaxation and vasodilation (Francis et al. 2010). Prostacyclin has also antiproliferative, antithrombotic, anti-inflammatory and antimitogenic effects on vascular cells. On the other hand, prostanoids, such as PGD2 and PGF2, produced in vascular endothelium modulating intracellular Ca2+ concentration produce vasoconstriction (Siti et al. 2015).


Fig. 3
The synthesis of the prostacyclin from prostaglandin (PG) G2 and thromboxane (TX) A2. TXA2 shows interactions in contrast to PGI2. IL-1 Interleukin-1, PDGF Platelet derived growth factor, EGF Epidermal growth factor, R PGI2 receptor
Endothelium‐Derived Hyperpolarizing Factor
The term of EDHF represents a mechanism rather than a specific factor (Luksha et al. 2009). Prostacyclin and NO can be considered as an endothelium-derived hyperpolarizing substance. Because most of the available inhibitors of cyclooxygenase abolish the production of prostaglandins in vascular tissues, any endothelium-dependent hyperpolarization observed in the presence of one of these inhibitors is unlikely to involve prostacyclin. NO can also hyperpolarize, or repolarize, vascular smooth muscle cells by activating, in either a c-GMP dependent or –independent pathways through potassium channels such as K-ATP. NO interacts with other ionic channels of the smooth muscle, including chloride and cationic channels and also influences the membrane potential of the smooth muscle cells indirectly in an autocrine fashion (Félétou and Vanhoutte 2006). Members of a class of arachidonic acid derivatives, the epoxyeicosatrienoic acid, hydrogen peroxide, C-type natriuretic peptide, have been suggested to function as an EDHF in some vascular beds. These molecules are accepted as non-NO–non-PGI2– EDHFs. EDHF is proposed to be a substance and/or electrical signal that is generated or synthesized in and released from the endothelium. Its function is to hyperpolarize vascular smooth muscle cells, causing these cells to relax. EDHFs are able to activate ion channels, and initiate smooth muscle hyperpolarization and relaxation (Luksha et al. 2009). Calcium-activated K+ channels are opened by EDHFs in vascular smooth muscle cells. The effects of EDHF’s are highest on the small arteries, and are very significant for the regulation of organ blood flow, peripheral vascular resistance and blood pressure, particularly when production of NO is depressed (Michel and Vanhoutte 2010; Luksha et al. 2009; Félétou and Vanhoutte 2006; Khazaei et al. 2008).
Endothelins
Endothelins (ETs) are potent vasoconstrictor molecules having a key role in vascular homeostasis (Fig. 4). Although there are three types of ET, vascular ECs produce only ET-1 which has prominent roles (Wang and Zhao 2010). ET-1 is a 21 amino acid peptide that is synthesized from a 39 amino acid precursor named pre-pro endothelin. Active endothelin molecule is generated by the actions of an endothelin converting enzyme (ECE) found on the endothelial cell membranes. There are two basic types of ET-1 receptors: ETA and ETB. Both of these receptors are coupled to a G-protein and to the formation of IP3 (Barton 2011; Kedzierski and Yanagisawa 2001). In blood vessels, the ETA receptor is dominant under normal conditions. ET-1 produces vasoconstriction through activation of L-type Ca+2 channels by binding to ET-A receptors on vascular smooth muscle cells. In addition to the presence of both ETA and ETB receptors on the smooth muscle, ETB receptors are also found on the endothelium, and under the control of vascular tone, considerable cross-talk between ET, NO and prostacyclin occur (Vanhoutte et al. 2009). When ET-1 binds to endothelial ETB receptors, the formation of NO is stimulated but in the absence of smooth muscle endothelin receptor stimulation, NO causes vasodilation. The other effects of ETs include cell growth, embryonic development, renal functions, neurophysiological functions (such as pain signaling), cardiovascular homeostasis, cancer cell growth, endocrine function, inflammation, pulmonary functions (such as bronchoconstriction) and reproductive system functions (Khazaei et al. 2008). ET-1 production and release are stimulated by AT-II, antidiuretic hormone (ADH), thrombin, cytokines, reactive oxygen species, and shearing forces acting on the vascular endothelium. The ET-1 release is inhibited by prostacyclin, atrial natriuretic peptide and NO (Davenport et al. 2016).


Fig. 4
Endothelin (ET); receptors (ETA and ETB), mechanisms and effects. PLC Phospholipase C, PIP Phosphatidyl inositol diphosphate, IP 3 Inositol triphosphate, DAG Diacylglycerol, MAPK Mitogen activated kinase, AAArachidonic acid, PGG 2 Prostaglandin G2, PGI 2 Prostaglandin I2
Angiotensin I and II
Angiotensin II (AT-II) cause structural changes and vasoconstriction in the arterial wall by affecting many cellular and intracellular events in smooth muscle. Two types of AT-II receptors on ECs are determined. AT-II type-1 receptor is particularly involved in the contraction of vascular smooth muscle cells. AT-II-type-1 receptor blockers increase the release of NO and prostaglandins whereas AT II- type-2 receptors provide the activation of the endothelial relaxation (Masuyer et al. 2014; Jiang et al. 2014).
Thromboxane
Thromboxane (TX) A2 is a member of the eicosanoid lipid family. TXA2 is generated from prostaglandin H2 by thromboxane-A synthase. TXA2 acts by binding to the G-protein-coupled thromboxane receptors. Thromboxane is a vasoconstrictor, and it facilitates platelet aggregation (Fig. 3). Therefore, TXA2 shows interactions in contrast to prostacyclin (Bauer et al. 2014; Korbecki et al. 2014).
Adenosine
The vascular ECs releases adenosine to produce vaso-relaxation through activation of purinergic (P2) receptors. Adenosine release is related to local oxygen tension. Also, adenosine metabolites play roles in local vasoregulation and in the physiological control of blood pressure (Ralevic and Dunn 2015).
2.2 Inflammatory and Immune Response of ECs
Many stimuli associated with inflammatory and immune vascular diseases have been reported to induce endothelial cell apoptosis (Winn and Harlan 2005a). Endothelial cells produce and react to a variety of cytokines (these include chemokines, colony-stimulating factors (CSF), Interleukins (IL), growth factors, and interferons (IFN) and other mediators). Therefore, ECs have important roles in defense and inflammation. The chemokines from ECs affect leukocytes (neutrophils, eosinophils), T lymphocytes, natural killer cells and monocytes. Since ECs are located at the tissue-blood interface, they present several chemokines to circulating leukocytes. When production of chemokines is elevated, Tumor Necrosis Factor (TNF)-α and IL-1 for the receptor (so called as decoy receptor) are released into the circulation (Vanhoutte et al. 2009). IL-1 and TNF-α are synergistically effective on the expression of pro-inflammatory genes in various cells. Endothelial cells also produce granulocyte macrophage CSF (GM-CSF), granulocyte CSF (G-CSF), macrophage CSF (M-CSF), the stem cell factors, IL-1 and IL-6 and TNF receptors. ECs by themselves are targets of the inflammatory response. TNF-α and TNF-β are produced by activated macrophages and activated T cells, respectively. These activate ECs and neutrophil aggregation, as well as NO synthesis. Inflammatory disease progression depends on the balance between pro-inflammatory and anti-inflammatory cytokines. ECs involve the systemic anti-inflammatory response by producing anti-inflammatory cytokines such as an IL-1 receptor, IL-10, IL-13, and Transforming Growth Factor (TGF)-β. Anti-inflammatory cytokines can either block the process initiated by pro-inflammatory cytokines or suppress the inflammatory cascade. While cytokines such as IL-4, IL-10, IL-13, and TGF-β suppress the production of IL-1, TNF-α, other pro-inflammatory cytokines block the production of these cytokines (Mai et al. 2013). TGF-β is also produced by macrophages, T cells, and endothelium and generally works as a growth inhibitor of ECs. Additionally, IL-8 stimulates proliferation and migration of ECs and have angiogenic properties (Medzhitov 2008; Levesque et al. 1990).
ECs facilitate leukocyte movement into tissues through adhesion molecules such as E-selectin, P-selectin, intercellular adhesion molecule-1 (ICAM-1) and vascular cell adhesion molecule (VCAM). Resting ECs are considered not to be adhesive to circulating leukocytes. ICAM-2 is expressed on resting ECs, whereas ICAM-1 and VCAM are minimal on resting state and their expression can be increased by cytokines and endotoxin activation. Lymphocytes, platelets, and other leukocytes can interact with ECs under basal conditions via the L-selectin receptor. When lymphocytes are activated, they express integrins, which interact with ICAM and VCAM. L-selectin, as an adhesion molecule, and β2 integrin are involved in the adherence of leukocytes to ECs. Activated ECs also secrete platelet activating factor (PAF) and stimulate the expression of P-selectin and E-selectin. PAF upregulates integrins on leukocytes. Activated platelets binds to CD40 on ECs (Vanhoutte et al. 2009; Tuttolomondo et al. 2012; Tummala et al. 1999; Szmitko et al. 2003).
The endothelium is also capable of expressing various growth factors including G-CSF, M-CSF, GM-CSF, platelet-derived growth factor (PDGF), vascular endothelial growth factor (VEGF), and fibroblast growth factors (FGF). CSFs and growth factors produced by the endothelium are also important for hematopoiesis which increases the number of immune cells in the circulation during inflammation (Croft et al. 2009).
The immune system has important roles in the defense mechanism against infections or in response to tissue injury. Dendritic cells (DC), macrophages, natural killer (NK) T cells, and Toll-like receptors (TLRs) are components of the immune system. ECs actively participate in both innate and adaptive immune responses through producing cytokines and chemokines which recruit phagocytes to the site of infection. Endothelial permeability is also increased, allowing for additional trafficking of immune cells during inflammation. Although ECs at rest do not interact with leukocytes, activated ECs increase the expression of adhesion molecules and chemokines and interact with immune cells during the inflammatory process. ECs also can serve as antigen presenting cells by expressing both MHC I and II molecules and presenting endothelial antigens to T cells during inflammation. Both TLRs (TLR2 and TLR4) and NLRs are expressed in inflamed endothelium. When inflammation is dominated by TH1 cells, ECs express chemokine ligand 10 (CXCL10) and E-selectin, which favors the recruitment of TH1 cells. EC surface molecules such as lymphocyte function-associated antigen (LFA)-3 and ICAM-1 increase the production of IL-2 and IL-4 by T cells. ECs with activated T cells enhance IFN-γ production via OX40 (CD134) signaling (OX40 is a member of the TNFR/TNF superfamily and are expressed on the activated CD4 and CD8 T cells). An anti-angiogenic cytokine derived from ECs, vascular endothelial growth inhibitor functions to suppress ECs proliferation in a cell cycle-dependent manner lipopolysaccharide, which induce ECs to produce IL-1, IL-8, and monocyte chemotactic protein-1 (MCP-1). Like LPS, tumor necrosis factor-α (TNF-α), and interferon-γ (IFN-γ) can induce TLR2 expression via an NF-kB-dependent pathway. ECs also express CD14, a known receptor for LPS and IFN-α, which is an important cytokine in regulating innate immune responses against viruses (Mai et al. 2013; Croft et al. 2009; Rodriguez-Iturbe et al. 2014).
Under healthy conditions, ECs express the lectin-like oxidized low-density lipoprotein (oxLDL) receptor (LOX-1) at low levels. Expression of LOX-1 in ECs is elevated in response to stimulation by oxLDL, pro-inflammatory cytokines, and pro-atherogenic factors such as AT-II. OxLDL also induces cell surface adhesion molecule expression and impair NO production in ECs by increasing superoxide generation. LOX-1 has a role in the mediation of endothelial phagocytosis of aged red blood cells and apoptotic cells. LOX-1-mediated phagocytotic activity can be inhibited by oxLDL. Thus, LOX-1 is important in endothelial-mediated vascular homeostasis and coagulation prevention under physiological conditions (Pirillo et al. 2013; Dunn et al. 2008).
ECs can also induce suppressive immune function in T cells. Mechanistically, after contact with ECs, regulatory T cells upregulate the expression of programmed death-1 receptor and increase the production of anti-inflammatory cytokines IL-10 and TGF-β (Tselios et al. 2014; Pastrana et al. 2012).
Recently, it has been shown that ECs also induce cellular signaling by endothelial microparticles (EMPs). EMPs are small plasma membrane-derived vesicles (0.1–1.5 μm in diameter), are released by various cell types during cell activation or apoptosis (a type of programmed cell death). Microparticle formation induced by various factors, including TNF-α, IL-1β, thrombin, calcium ionophore, and reactive oxygen species. Microparticles express surface antigens from their cells of origin which allow for the identification of their sources. Circulating EMPs are biomarkers of inflammation and contribute to the pathological state. Depending on the nature of the stimulus, EMPs contain endothelial proteins such as ICAM-1, integrin, and cadherin. EMPs also have endothelial nuclear materials such as microRNA, RNA, and DNA, which can induce intracellular signaling via the transfer of these nuclear materials and proteins to target cells. EMPs also have pro-coagulant and pro-adhesive properties, which promote coagulation and vascular inflammation. EMPs were also found to induce the maturation of plasmacytoid dendritic cells. Plasmacytoid dendritic cells matured by EMPs secrete pro-inflammatory cytokines IL-6 and IL-8 (Yuana et al. 2013; Bernal-Mizrachi et al. 2003; Helbing et al. 2014a).
2.3 The Link Between Hemostasis and Coagulation and ECs
The endothelium plays a pivotal role in the regulation of the hemostatic balance, and endothelial and smooth muscle cells express several proteins participating in hemostasis. Hemostasis is a complex event. Multiple interactions between blood cells and the damaged vessel wall, the coagulation proteins, and blood cells and the cell-cell interactions are required in the hemostatic process (Fig. 5). In physiological state, healthy ECs express antiplatelet and anticoagulant molecules that prevent platelet aggregation and fibrin formation, respectively. Injury to endothelium leads to loss of protective molecules and the appearance of adhesive and pro-coagulant activities. When coagulation proteins are activated by their specific receptors on the vascular cell surface, in turn, these cells lead to the expression of genes involved in coagulation, angiogenesis, leukocyte adhesion and regulation of the vascular wall tone (Stenina 2003; Yau et al. 2015).


Fig. 5
Endothelium has both anticoagulant and coagulant activities (see text); PAR-1 Protease-activated receptor-1, t-PA Tissue type plasminogen activator, u-PA urokinase type plasminogen activator, vWF von Willebrand factor (Şekilde Türkçe Karakterler mevcut)
Tissue factor (TF) is the receptor for factor VII and is a pro-coagulant. It is inhibited by tissue factor pathway inhibitor (TFPI), which is synthesized by ECs and is one of the most important endothelium-derived inhibitors of the blood coagulation cascade. TF activates factor X, which then combines with factor Va to convert prothrombin to thrombin. Thrombin has pro-coagulant activity. It binds to thrombomodulin which is expressed on the ECs surface. Thrombomodulin requires for the pro-coagulant effects of thrombin as a cofactor in normal vessels. The thrombin- thrombomodulin complex activates protein C. This process is forced by the endothelial protein C receptor (EPCR). Activated protein C (APC) is an effective anticoagulant through the inactivation of factor Va. Thrombin is also chemotactic for polymorphonuclear leukocytes and is a potent inducer of platelet activating factor (PAF) expression in ECs. Thrombin is also involved in the process of inflammation and can up-regulate endothelial cell P-selectin expression through von Willebrand factor (vWF). Endothelium also produces and secretes vWF, mediating platelet adhesion and shear stress-induced aggregation. vWF, which is also synthesized within megakaryocytes and the α-granules of platelets, is a multimeric adhesion glycoprotein. vWF is essential for platelet adhesion to collagen via the platelet receptor glycoprotein Ib-FV-FIX at sites of vascular injury. The vWF binds and stabilizes factor VIII and is a cofactor for platelet binding to exposed extracellular matrix in injured vessel walls (Vanhoutte et al. 2009; Stenina 2003; Yau et al. 2015; Steffel et al. 2006; Esmon 2006).
Under physiological conditions, the endothelium prevents thrombosis. Endothelial protease-activated receptors (PARs) serve as sensors for proteases and initiate a cascade of cell signals upon activation by thrombin, APC, FXa, the TF/FVIIa/FXa complex, high concentrations of plasmin, and matrix metalloproteases. Thrombin-mediated activation of PAR-1 is responsible for the production of NO and prostacyclin, which limits platelet activation, induces the activation of Weibel-Palade bodies, releasing VWF and t-PA, and mediates the surface exposure of TF. Thus, PARs (especially PAR-2) play an important role in the pro-coagulant response upon stimulation, and this induces pro-inflammatory responses (Lacave et al. 1989; Lijnen and Collen 1997).
Platelets play a fundamental role in preventing blood loss by forming the platelet hemostatic plug and to serve as a platform for coagulation factors. Platelet-endothelium interactions play an important role in the activation and regulation of platelets. While an intact endothelium inhibits the adhesion of platelets, through the release of NO and PGI2, activated ECs express a variety of molecules and receptors that increase platelet adhesion to the site of injury. In ECs, Weibel-Palade bodies store, vWF, P-selectin, angiopoietin-2, t-PA, and ET-1, which are active participants of platelet adhesion, leukocyte recruitment, inflammation modulation, fibrinolysis, and vasoconstriction, respectively. Following vascular insult or in the presence of vasoactive agents such as histamine, bradykinin, and thrombin, endothelial Weibel-Palade bodies fuse with the plasma membrane and release these products into the space wherein they perform their specific functions (Francis et al. 2010). Normal ECs also produce enzymes called ectonucleotidases, which dephosphorylates ADP to AMP and then to adenosine and inhibit platelet aggregation, and release matrix metalloproteases (MMPs) to cleave platelet aggregates. TXA2 produced by activated platelets, has prothrombotic properties, stimulating activation of new platelets as well as increasing platelet aggregation. Platelet aggregation is achieved by mediating expression of the glycoprotein complex GP IIb/IIIa in the cell membrane of platelets. Circulating fibrinogen binds to these receptors on adjacent platelets, further strengthening the clot (Yau et al. 2015; Steffel et al. 2006; Esmon 2006; Perutelli et al. 1992; Lacave et al. 1989; Lijnen and Collen 1997).
ECs synthesize and secrete plasminogen activator (PA) to degrade the clot and plasminogen activator inhibitor (PAI), and thus provide anticoagulant and pro-coagulant regulatory mechanisms, respectively. Additionally, MMPs are released from ECs to cleave platelet aggregates. Fibrin degradation is trigged by some fibrinolytic molecules, such as tissue –type PA (t-PA) and urokinase-type PA (u-PA). t-PA is predominantly found in ECs while u-PA is expressed in ECs, macrophages, renal epithelial cells and some tumor cells. T-PA convert plasminogen to plasmin. Both plasminogen and plasminogen activators (t-PA and u-PA) bind to specific cellular receptors; assembly of components of the fibrinolytic system at the endothelial cell surface results in stimulation of fibrinolytic activity. Thus, t-PA provides an essential method for removal of blood clots (Salame et al. 2000; Shih and Hajjar 1993; Barnathan et al. 1990).
2.4 Hemodynamic Factors and Endothelial Cell
Flow rate and pressure in the blood vessels also affect the smooth muscle tone. The increase in flow velocity (shear stress), via ion channels (calcium, potassium and sodium) stimulates eNOS activity and the synthesis of NO from ECs. The increase in pressure reduces both the stress and release of endothelin from NO; vascular shear stress can also influence the coagulant potential of ECs. Arterial shear stress can induce the transcription factors. Likewise, reduced venous shear stress can induce hypoxia and stimulate the release of P-selectin and von Willebrand factor from ECs. The nature of the shear stress also has a significant influence on the type of thrombi that forms. Arterial clots form under high shear stress after atherosclerotic plaque rupture and are rich in platelets (called as white clot). In contrast, venous thrombi develop under low shear stress and are rich in fibrin and red blood cells (called as red clot). It has been indicated that plasma viscosity is a major determinant of capillary blood flow, and alteration in plasma viscosity contributes to impaired blood flow and to increased cardiovascular risk (Reneman et al. 2006; Ballermann et al. 1998; Li et al. 2005b; Ercan et al. 2003).
2.5 Angiogenesis
Since ECs are an important component of blood vessels, they can be triggered to induce angiogenesis upon stimulation (Francis et al. 2010). VEGF is an angiogenic factor produced by ECs, with specific receptors on the endothelium. The formation of new blood vessels from pre-existing endothelium is mediated by VEGF. VEGF also contributes to the inflammatory response through stimulation of the release of adhesion molecules, MMPs and NO, via the transcription factor activator protein-1 (AP-1) (Kim and Byzova 2014; Jaipersad et al. 2014).
The coagulation system plays a major role in the development of angiogenesis. Activated protein C stimulates angiogenesis in brain endothelium, and cross-linked fibrin serves as a scaffold for ECs to synthesize new blood vessels. Platelets contain a rich source of vasoactive agents and chemokines, such as serotonin, TA2, PAF and pro-angiogenic growth factors, such as vascular VEGF. VEGF can stimulate/upregulate eNOS and has physiological role for the normal endothelial control of vasomotor tone (Jaipersad et al. 2014).
An anti-angiogenic cytokine derived from ECs, vascular endothelial growth inhibitor functions to suppress EC proliferation in a cell cycle-dependent manner. These compounds stimulate ECs proliferation and promote the growth of new blood vessels. The expression of TF has been shown to induce tumor angiogenesis through TF-FVIIa-dependent PAR-2 activation which induces the expression of VEGF, IL-8, and MMP-7. It has been suggested that TF isoform may play a prominent role in promoting the angiogenesis (Mai et al. 2013; Yau et al. 2015).
Laminar shear stress is also a potent antiapoptotic stimulus in ECs. Some postulated mechanisms of protection include up-regulation of NOS, as well (Ercan et al. 2014).
3 Endothelial Dysfunction
Healthy endothelium has some athero-protective role including promotion of vasodilation, antioxidant and anti-inflammatory effects, inhibition of both leukocyte adhesion and migration and smooth muscle cell proliferation and migration. Healthy endothelium has anticoagulant and profibrinolytic effects, as well as the inhibitory effects on platelet aggregation and adhesion. Impaired endothelium-dependent vasodilation is also associated with the state of endothelial activation which is characterized by elevated pro-inflammatory and pro-coagulatory events (Fig. 6). The major factors for endothelial dysfunction are a reduction of the NO bioavailability, impairment in the response of vascular smooth muscle to the vasodilators, the elevated sensitivity of ECs against vasoconstrictors, increased production of the vasoconstrictor substances, or elevated shear stress (Fig. 7). Traditional and nontraditional risk factors for cardiovascular events, diabetes mellitus, atherosclerosis and hypertension are associated with enhanced ROS or increased oxidative stress. Increased oxidative stress is considered as a major mechanism involved in the pathogenesis of endothelial dysfunction. Disturbance of NO metabolism (elevated degradation of NO, inactivation of NO, or presence of NO inhibitors; Fig. 8) may be due to the elevation in oxidative stress (González et al. 2014; Bonetti et al. 2003).




Fig. 6
Oxidative stress induced endothelial dysfunction

Fig. 7
A balance between endothelium‐derived relaxing factors (EDRFs) and endothelium‐derived contracting factors (EDCFs)

Fig. 8
Nitric oxide (NO) bioavailability
3.1 NO and Endothelial Dysfunction: The Link with Oxidative Stress
Oxidative stress has been implicated in the pathophysiology of many cardiovascular conditions, including hypertension. ROS significantly increase the influence of stimulants such as inflammation, radiation, high partial oxygen pressure, advanced age, obesity, and chemical substances. Oxidative stress that increases on a cellular level results in oxidative damage by altering the structure of molecules such as deoxyribonucleic acid, amino acid, protein, lipid, and carbohydrate (Fig. 9). A particularly important radical for cardiovascular biology is superoxide, which is formed by the one-electron reduction of oxygen. Superoxide can serve as both an oxidant and as a reductant and is a progenitor for other ROS. Other radicals include the hydroxyl radical, lipid peroxyl radical, and alkoxyl radicals. Other molecules, including peroxynitrite, hypochlorous acid, and hydrogen peroxide are not radicals but have strong oxidant properties and are, therefore, included as ROS. Another a group of molecules is the reactive nitrogen species (RNS) including NO, the nitrogen dioxide radical, and the nitro sodium cation. The main sources for oxidative excess in the vasculature are adenine dinucleotide phosphate (NADPH) oxidase (NOX), xanthine oxidase, the mitochondrial and uncoupled NOS (Zhao et al. 2014; Bonetti et al. 2003; Ferroni et al. 2006).


Fig. 9
Oxidative stress has been implicated in the pathophysiology of many cardiovascular conditions, including hypertension. ROS significantly increase the influence of stimulants such as inflammation, insulin resistance, dyslipidemia, advanced age and obesity which are related to decreased nitric oxide bioavailability
NOX catalyzes the reduction of molecular oxygen by NADPH as an electron donor, thus generating superoxide. Superoxide anion is a major determinant of NO synthesis and availability, and can act as a vasoconstrictor. Superoxide combines with NO, which is synthesized by eNOS, to form peroxynitrite, in turn, peroxynitrite oxidizes and destabilizes eNOS to produce more superoxide. Superoxide also leads to BH4 oxidation, which is a cofactor for NO synthesis. Vascular superoxide is derived primarily from NOX when stimulated by hormones such as AT-II and ET-1. Xanthine oxidase is also an important source for oxygen free radical present in the vascular endothelium. It involves purine metabolism. During this process oxygen is reduced to superoxide (González et al. 2014).
eNOS is an important source of superoxide and peroxynitrite. In addition AT-II, acting through the AT1 receptor stimulates NOX causing the accumulation of superoxide, hydrogen peroxide, and peroxynitrite. Peroxynitrite is generated from NO in the increased oxidative stress conditions. It plays proatherogenic roles by leading to oxidation of LDL and degradation of the eNOS cofactor. ROS upregulate VCAM-1, ICAM-1 and MCP-1. Oxidative excess is also linked to a pro-inflammatory state of the vessel wall. Inflammation decreases NO bioavailability. On the other hand, under pathological conditions, EDHF can compensate for the loss of NO in arteries. The effects of EDHF are greatest at the level of small arteries. The changes in the EDHF action are of critical importance for the regulation of organ blood flow, peripheral vascular resistance, and blood pressure (Luksha et al. 2009; Ceriello 2008).
3.2 Asymmetric Dimethylarginine and Endothelial Dysfunction
Asymmetric dimethylarginine (ADMA) is endogenous competitive inhibitor of eNOS. It is created in protein methylation, a common mechanism of post-translational protein modification, which is catalyzed by N-methyltransferases. ADMA is eliminated by excretion through the kidneys or metabolism to citrulline by the enzyme dimethylarginine dimethylaminohydrolase (DDAH). ADMA is one of the molecules associated with oxidative stress. Oxidative stress increases the plasma ADMA levels by increasing the activity of enzymes that take part in the production of ADMA and by decreasing the activity of enzymes that take part in metabolizing ADMA. The increased ADMA levels decrease the release of NO by inhibiting NOS. As NO decreases, hemodynamic changes and endothelial dysfunction occurs. Overexpression of DDAH also decreases ADMA levels and increase eNOS activity. Protein arginine methyltransferases, which produce methylated arginines, were shown to be upregulated by shear stress, and this upregulation was associated with enhanced ADMA generation (Endemann and Schiffrin 2004; Papageorgiou et al. 2015; Siervo et al. 2011).
3.3 LDL Oxidation and Endothelial Dysfunction
Oxidation plays a role in the pathogenesis of atherosclerosis. The oxidation of LDL triggers the uptake of the uptake of oxLDL by macrophages and the formation of foam cell. Also, oxidation processes may result in oxidized lipids with pro-inflammatory effect (Fig. 10). The other lipids which present in the blood vessel wall lead to inflammation and atherosclerosis (Stancel et al. 2016; Ouweneel and Van Eck 2016).


Fig. 10
oxLDL activates macrophages and generation of foam cells. Foam cells trigger the both production and secretion of growth factors and cytokines which stimulate cells proliferation and matrix degradation. MCP-1 Monocytechemotactic factor-1
3.4 Homocysteinemia and Endothelial Dysfunction
Homocysteine (Hcy) is a sulfhydryl-containing amino acid. It is synthesized from the demethylation of methionine. The presentation of either methyl tetrahydrofolate or betaine, Hcy may be converted into methionine by methylation reaction or may be metabolized to cysteine by the sulfuration reaction. It has been shown that hyperhomocysteinemia is a major and independent risk factor for cardiovascular disease. Hcy cause arteriosclerosis by damaging the endothelium either directly or by altering oxidative status. In presence of hyperhomocysteinemia, Hcy autoxidation occurs, which may stimulate the production of hydroxyl radicals, as known as oxidative stress initiators. Also, Hcy mediates LDL-autoxidation and changes the redox thiol status in mitochondrial gene expression. Homocysteine and/or adenosine exposure of ECs cause apoptosis (McCully 2015; Pushpakumar et al. 2014).
3.5 Coagulation and Inflammation Pathways and Endothelial Dysfunction
Endothelial dysfunction is responsible for inflammation and blood coagulation. During endothelial dysfunction, ECs become activated and contribute to the pathogenesis of thrombosis. Hypoxic conditions often lead to endothelial dysfunction and promote the release of VWF from ECs. Inflammation can be accompanied by thrombosis. Proinflammatory cytokines, such as TNF-α and IL-1, upregulate the production of TF and VWF, while attenuating the expression of thrombomodulin, NO and prostacyclin. Patients with systemic inflammation show an impaired protein C system due to impaired protein C synthesis and impaired protein C activation. While protein C is synthesized by hepatocytes, ECs can regulate protein C activation through the expression of thrombomodulin. As such, thrombomodulin levels are significantly down-regulated by the presence of pro-inflammatory cytokines, such as TNF-α and IL-1, resulting in diminished protein C activation. These events result in a shift from anti-thrombotic to pro-thrombotic conditions (Yau et al. 2015; Goldenberg and Kuebler 2015; Kleinegris et al. 2012).
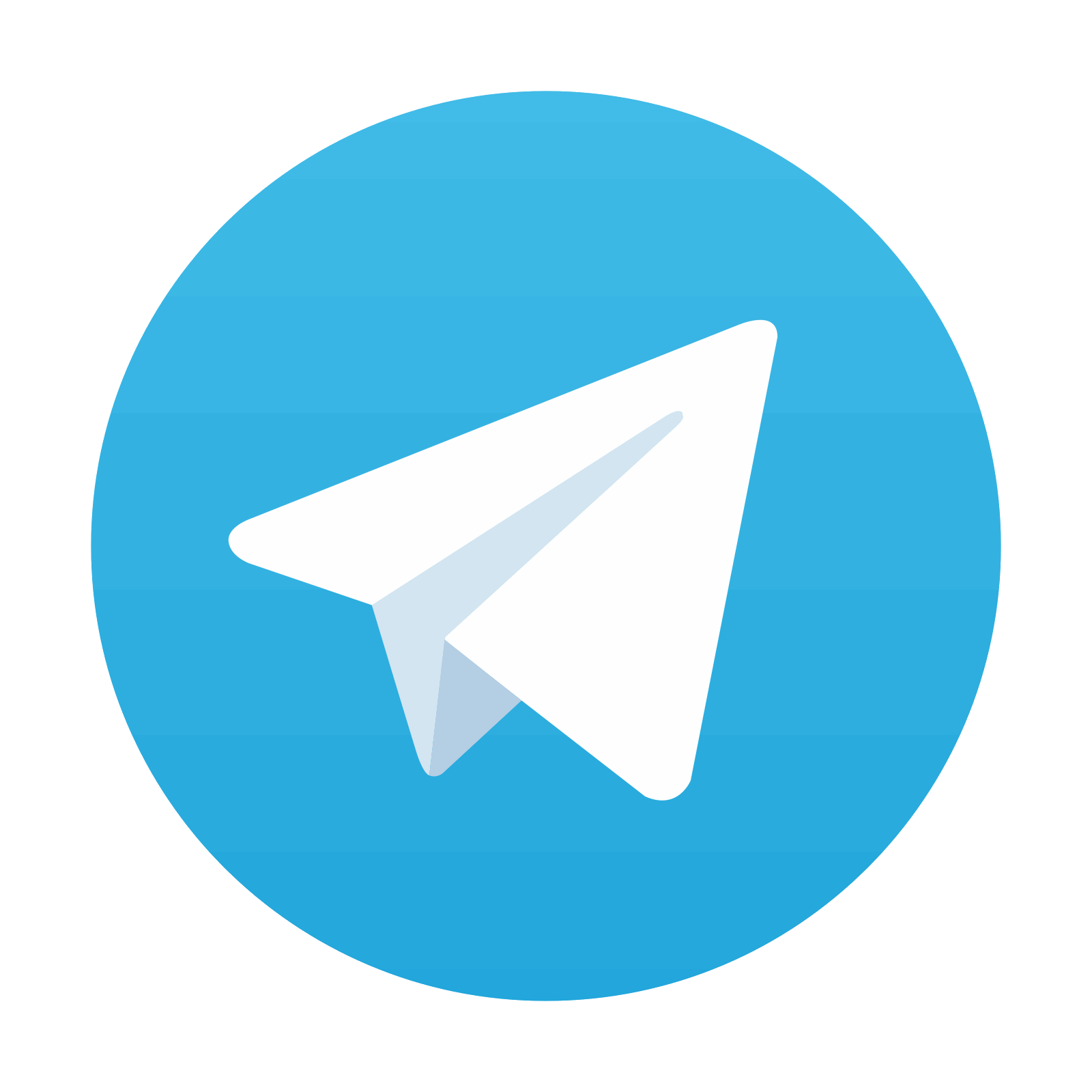
Stay updated, free articles. Join our Telegram channel

Full access? Get Clinical Tree
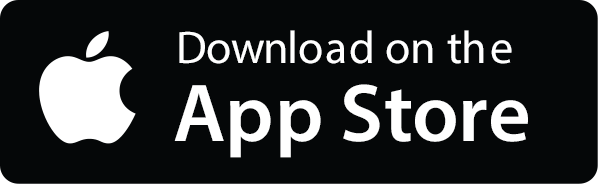
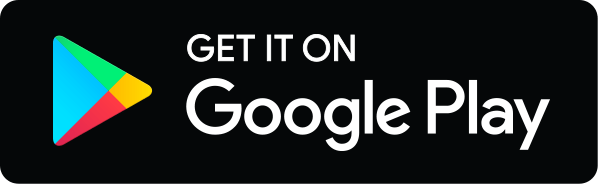