Duplex Scanning Prior to Flap Procedures for Breast Reconstruction
Molly J. Zaccardi
Jean Hadlock
Richard P. Rand
There have been substantial advances over the past four decades in the area of postmastectomy breast reconstruction. At the present time, it is usually possible to provide most patients with a high-quality reconstruction using either alloplastic implant products or autogenous tissue flaps. In some cases, a combination of the two techniques is required. However, most plastic surgeons would agree that the best reconstructions come from a patient’s own tissue. Using the patient’s tissue provides a natural-feeling breast that can be sculpted by the surgeon to match the opposite breast. For those patients requiring unilateral reconstruction, an implant is much more limited in its ability to match the opposite natural breast. In addition, the frequent use of radiation therapy in modern breast cancer treatment has a significant impact on reconstructive options. Radiated tissues will not generally allow for tissue expansion and implant reconstruction without a high risk of developing a hard and misshapen breast mound. For that reason, autogenous tissue flap reconstructions are best in radiated patients because they bring their own healthy blood supply and replace much of the damaged tissue with nonradiated skin and fat.
FLAP PROCEDURES FOR BREAST RECONSTRUCTION
The most common source of tissue used to restore the breast comes from the lower abdomen. In the early 1980s, surgeons discovered that the same tissue that is disposed of in a tummy tuck could be transferred to the chest to create a breast mound. The patient benefits by receiving the most natural reconstruction of the breast while also obtaining a flat, tighter abdomen. There are multiple ways to transfer the vascularized tissue from the abdomen based on various blood flow options, as listed in Table 36.1.
The blood supply to the transverse rectus abdominis myocutaneous (TRAM) flap travels down through the rectus muscle from the continuation of the internal mammary vessels in the chest as the superior epigastric artery and vein. Maintaining this blood supply allows the surgeon to elevate and rotate the flap and transfer it to the chest through a tunnel made over the xiphoid. Once on the chest, it is shaped into the breast mound. Using only one rectus abdominis muscle is called a “single-pedicle flap”; using both muscles is the “double-pedicle flap.” Both of the muscles are used in unilateral reconstruction cases when there is a midline scar in the lower abdomen that blocks blood flow across the scar or when the surgeon feels the need to use all of the tissue from the abdomen to make the appropriate breast size. The disadvantage of this approach is the significant sacrifice in abdominal wall integrity and function. In bilateral pedicled TRAM flap cases, both muscles must be used to supply blood to each side independently.
Pedicled TRAM flaps, particularly the single-pedicled variety, have the least robust blood flow because of the long distance from the origin of the blood supply to the flap. As a result, flaps based on the more dominant perfusion from the inferior epigastric artery system have been developed. However, because the origin of this pedicle is in the pelvis, it tethers the flap in that region and requires that it be disconnected and transferred. This is referred to as a “free flap” because it is temporarily separated from the body before being reattached in a new location. This requires the use of microsurgical techniques to reestablish blood flow to the flap in the area of the breast. The two most common recipient vessels for the free flap are the internal mammary artery and vein adjacent to the sternum and the thoracodorsal pedicle in the axilla.
In order to minimize the functional compromise to the abdominal wall, surgeons have continued to devise new methods to provide the flap with blood flow with little or no muscle sacrifice. These are all microsurgical procedures and include “muscle-sparing” free TRAM flaps in which only a small cuff of muscle is taken with the flap. Also, the deep inferior epigastric perforator (DIEP) and superficial inferior
epigastric artery (SIEA) flaps take no muscle with them at all. There has been much discussion among surgeons as to which of these microsurgical options is best. Fundamentally, it comes down to a balance between providing sufficient blood flow to the flap for its complete survival and minimizing the functional insult to the abdominal wall. Each of these operations is a one-time-only proposition and cannot be redone if the tissue does not survive. Consequently, all efforts must be made to maximize the blood flow to the flap, and the risk of outright flap loss should be less than 1%. Marginal blood flow leading to fat necrosis also needs to be rare, because fat necrosis creates hard lumps in the flap that may mimic recurrent cancer. To address these issues, we developed the technique of using color-flow duplex imaging to assess the blood supply of potential flaps for breast reconstruction. The ability to preoperatively map (locate and mark) the arterial perforators on the abdominal wall assists the surgeon in planning the procedure to ensure that the dominant vessels are included in the flap. This evaluation is useful for all of the flaps described in this chapter, but it is most important in those flaps that depend on fewer perforators for their perfusion.
epigastric artery (SIEA) flaps take no muscle with them at all. There has been much discussion among surgeons as to which of these microsurgical options is best. Fundamentally, it comes down to a balance between providing sufficient blood flow to the flap for its complete survival and minimizing the functional insult to the abdominal wall. Each of these operations is a one-time-only proposition and cannot be redone if the tissue does not survive. Consequently, all efforts must be made to maximize the blood flow to the flap, and the risk of outright flap loss should be less than 1%. Marginal blood flow leading to fat necrosis also needs to be rare, because fat necrosis creates hard lumps in the flap that may mimic recurrent cancer. To address these issues, we developed the technique of using color-flow duplex imaging to assess the blood supply of potential flaps for breast reconstruction. The ability to preoperatively map (locate and mark) the arterial perforators on the abdominal wall assists the surgeon in planning the procedure to ensure that the dominant vessels are included in the flap. This evaluation is useful for all of the flaps described in this chapter, but it is most important in those flaps that depend on fewer perforators for their perfusion.
TABLE 36.1 MULTIPLE APPROACHES TO TRANSFER OF TISSUE FROM THE ABDOMEN FOR BREAST RECONSTRUCTION BASED ON VARIOUS BLOOD FLOW OPTIONS | ||||||
---|---|---|---|---|---|---|
|
THE VASCULAR LABORATORY AND TRAM FLAP SURGERY
In the 1980s, a series of reports described the intraoperative use of a continuous-wave Doppler device to map the perforator arteries for TRAM flap surgery.1,2,3,4,5 These authors were primarily concerned with identifying variations in the anatomy of the blood vessels and selecting the flap segment most likely to have adequate perfusion. Michelow et al5 evaluated 66 patients undergoing breast reconstruction and found that approximately 80% had a single vascular axis coursing through the rectus abdominis muscle, while nearly 20% displayed two axes (Fig. 36.1). In this patient population, the majority of vascularity was found in the middle third of the rectus abdominis muscle; however, in approximately 28% of the patients, the blood supply was located in the medial or lateral third of the muscle. Many surgeons prefer not to use the entire rectus abdominis muscle in an effort to reduce complications such as postoperative hernias. The goal in a muscle-sparing procedure is to harvest a segment of muscle that has adequate perfusion. Rozen et al6 performed computed tomography angiography (CTA) in eight consecutive patients undergoing flap surgery for breast reconstruction. The CTA results revealed three distinct patterns of deep inferior epigastric artery branching (Fig. 36.2). The majority of perforators are in the region of the umbilicus, but the locations are rarely symmetrical from side to side. The anatomy of the rectus abdominis muscle and perforating arteries is shown in Figure 36.3.
In 1990, Miles Cramer from the vascular laboratory at the University of Washington and Dr. Richard Rand from the University of Washington Division of Plastic Surgery set out to improve on the intraoperative examinations of the early 1980s and introduced preoperative duplex ultrasound imaging to further reduce the risk of tissue loss in breast reconstruction surgery.7 They performed duplex scanning (B-mode imaging, pulsed Doppler spectral analysis, and color-flow Doppler) in 13 patients and 2 normal volunteers (all female)
between March and November 1991. Their hypothesis was that duplex scanning would provide more detailed anatomic and physiologic information than the continuous-wave Doppler examinations performed by others. In addition, this critical information could be obtained preoperatively to aid in surgical planning. Cramer et al7 were successful in documenting the following features:
between March and November 1991. Their hypothesis was that duplex scanning would provide more detailed anatomic and physiologic information than the continuous-wave Doppler examinations performed by others. In addition, this critical information could be obtained preoperatively to aid in surgical planning. Cramer et al7 were successful in documenting the following features:
Patency and continuity of the deep inferior epigastric arteries (Figs. 36.4 and 36.5)
Patency and continuity of the internal mammary arteries (Fig. 36.6)
Peak systolic velocities and diameter measurements for the perforator arteries (Fig. 36.7)
Locations of perforator arteries by zone and side, with reference to the umbilicus
Ability to mark each perforator artery on the skin with a high level of accuracy (Fig. 36.8)
They concluded that the preoperative duplex examination allows the surgeon to incorporate a greater number of perforators into the flap and provides reliable information to identify any abnormal or unusual anatomy. The approach described by Cramer et al7 has been the protocol at the University of Washington prior to flap procedures for breast reconstruction until recently when CTA has also been used to visualize and evaluate perforator vessels.8
VASCULAR EVALUATION PRIOR TO FLAP SURGERY
The duplex evaluation performed in the vascular laboratory prior to flap procedures for breast reconstruction can be divided into three parts. First, patency of the inflow vessels to the perforator arteries of the rectus abdominis muscle must be documented. Second, the perforator arteries are assessed for flow (peak systolic velocity), size (diameter), and number. The locations of the perforator arteries are also marked on the skin preoperatively. Third, patency of the internal mammary, subclavian, and axillary arteries is documented. Depending on the type of surgical procedure to be done, one of these arteries may become the sole source of perfusion to the flap.
![]() FIGURE 36.4. Color-flow image and Doppler spectral waveform from a common femoral artery (CFA) showing the origin of the deep inferior epigastric artery (IEA). |
Evaluate Inflow to the Flap Tissue
The inflow vessel to the perforator arteries of the rectus abdominis muscle is the deep inferior epigastric artery, which originates from the distal external iliac or proximal common femoral artery. The iliac and common femoral arteries are evaluated by duplex scanning, using the B-mode image to locate the vessels and pulsed Doppler waveforms to document the peak systolic velocities and flow patterns. The velocity criteria for lower extremity arteries established by Jager et al9 are applied. A normal external iliac or common femoral artery is characterized by a triphasic flow pattern and uniform peak systolic flow velocities in the range of 80 to 120 cm/s. The normal deep inferior epigastric artery peak systolic velocities were noted by Cramer et al7 to range from approximately 40 to 45 cm/s. Stenosis is suspected if there is a focal velocity increase followed by a turbulent, poststenotic flow pattern. Lower extremity arterial duplex scanning is discussed in detail in Chapter 12.
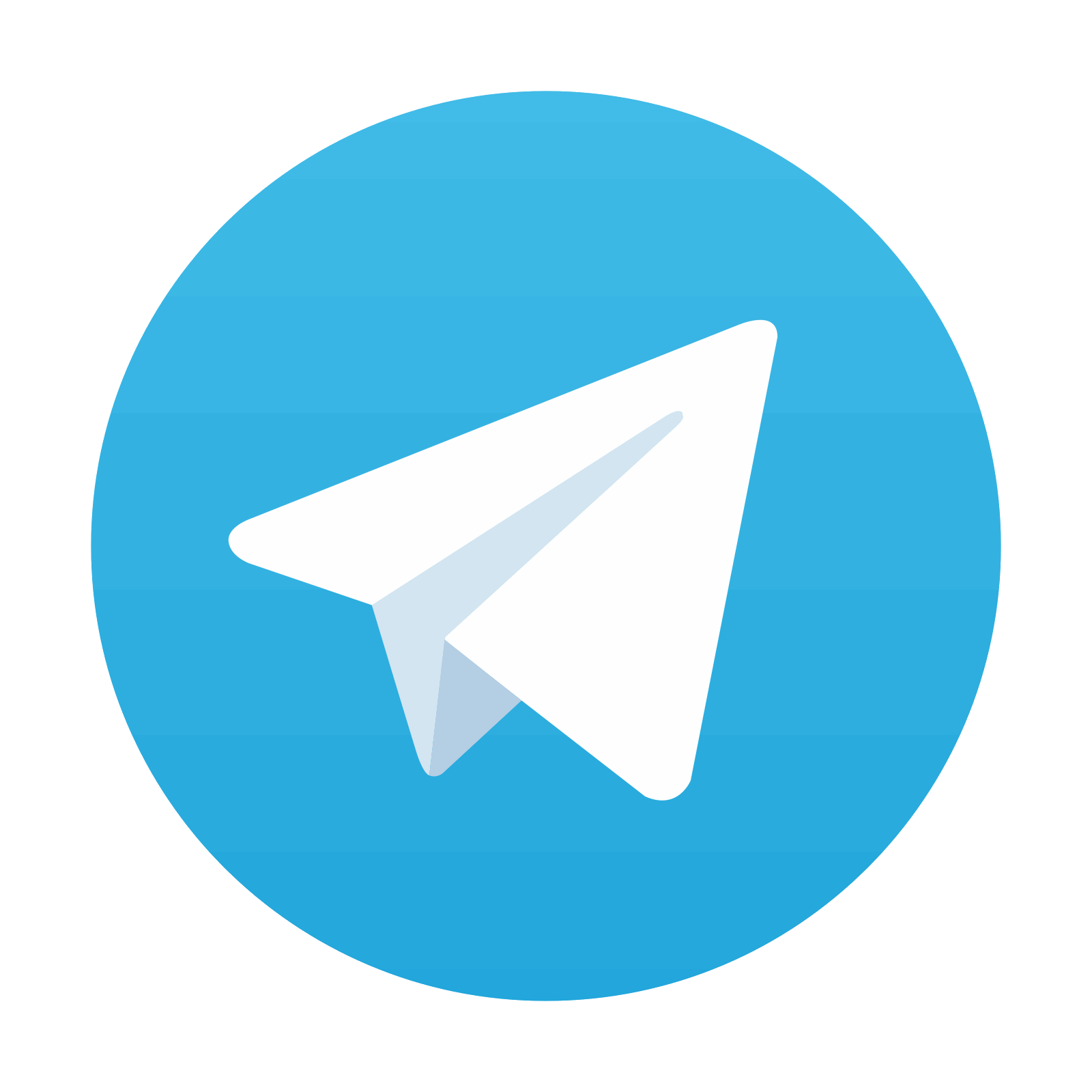
Stay updated, free articles. Join our Telegram channel

Full access? Get Clinical Tree
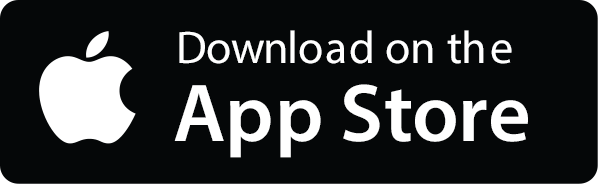
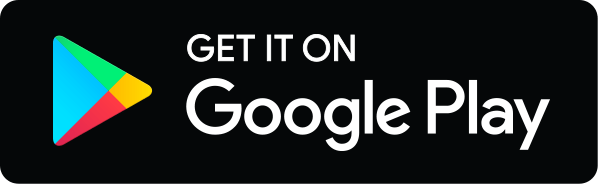