The concept of delivering antiproliferative drugs via balloon angioplasty has been around for several decades. However, the specific idea of developing a drug-coated balloon (DCB) system originated from the seminal work of professor Ulrich Speck in the use of contrast agents as carriers of antiproliferative drugs.1 At the early stages of his research, it was found that paclitaxel solubility was significantly increased when mixed when contrast agents. Experimental data showed that a repeated intracoronary bolus injection of a taxane-iopromide formulation resulted in significant reduction of neointimal proliferation in the porcine model of restenosis.2,3 In 2001, the concept of mixing iopromide with paclitaxel in a form of a balloon coating was reduced to practice and validated at the experimental level.4 This original DCB formulation was then clinically validated in small randomized controlled studies in the coronary in-stent restenosis5 and de novo superficial femoral artery stenosis settings.6,7 Stimulated by these early clinical results, several DCB programs were started following a similar technologic approach of using hydrophilic carriers as a method to transport paclitaxel into the vessel wall after balloon dilatation.
The development of drug-eluting stents (DESs) dramatically reduced the long-term rate of reintervention and improved clinical outcomes among patients undergoing percutaneous coronary interventions.4 However, despite their continuous technologic evolution and well-validated clinical efficacy, long-term complications (eg, target lesion revascularization) continue to occur.8 Several autopsy and in vivo imaging studies suggest delayed healing of the permanently implanted stent as the most important biologic mechanism responsible for these events.9,10 As a result, stent-based drug delivery technologies continue to evolve aiming to minimize the amount of permanent implantable components (ie, polymer) left behind following DES implantation.11
The concept of developing a balloon-based drug delivery system to prevent arterial restenosis was based on the hypothesis that a durable effect on neointimal proliferation could be achieved following single-drug delivery and potentially minimizing the impact of the chronically implantable components.12 This technologic approach appeared to contradict historical experimental and clinical data suggesting that sustained tissue exposure to therapeutic levels of drug was necessary to effectively inhibit neointimal proliferation.13 Due to its particular delivery mechanism and resulting pharmacokinetic profile, the promising results shown by the early DCB trials were originally challenged under the premise that sustained drug release was needed to attain long-term clinical efficacy.5,6,8
All DCBs developed to date use paclitaxel due to its high lipophilic profile, potent antiproliferative effect, and chemical stability following tissue delivery.14 Paclitaxel has shown to inhibit cell proliferation and migration due to an irreversible stabilization of intracellular microtubules, resulting in inhibition of cell replication during metaphase and anaphase of mitosis.15 Early experimental data showed that drug carriers are needed to transfer and maintain paclitaxel tissue levels over time.16 Thus, the pharmacokinetic characteristics of each DCB largely depend on the carrier and manufacturing process used to develop the coating. DCBs achieve the short-term transfer and long-term retention of paclitaxel to the arterial wall by different biologic mechanisms.17 Experimental data have shown that paclitaxel transfer and retention are not necessarily interrelated phenomena and largely depend on drug morphology and resulting solubility attained during the coating process (Fig. 32-1).18,19 At present, although a potential mechanism of action explaining long-term drug retention yielding sustained biologic efficacy following DCB drug delivery is still a matter of debate, important lessons have been learned regarding the pharmacokinetic behavior of some of these technologies. Early experimental data confirmed that paclitaxel transfer into the vessel wall occurs rapidly following balloon inflation.4 In addition, several tissue pharmacokinetic studies showed that the short-term tissue levels of paclitaxel following balloon delivery were several-fold higher compared with DES.20 Finally, most of the pharmacokinetic studies using clinically available DCB technologies have confirmed that paclitaxel tissue levels are successfully maintained over time (Fig. 32-2).17
Several experimental studies suggest that the presence of paclitaxel deposits on the surface of the vessel wall contribute to the biologic process of sustained local drug delivery. A recent study suggested that following DCB dilatation, a large proportion of the paclitaxel coating is retained on the vessel surface and is not actually dissolved into the tissue (Fig. 32-3).20 In this study, it seemed apparent that arterial tissue levels were directly related to the sustained retention of drug on the surface of the vessel wall, thereby providing a constant concentration gradient from the vessel surface into the arterial wall over time. Interestingly, at 7 days, vessel wall concentrations began to equalize to the vessel surface levels, providing an explanation regarding the lack of tissue toxic effects despite the apparent supratherapeutic tissue levels found in pharmacokinetic studies.20 These results largely depended on the degree of paclitaxel crystallinity and seemed to be consistent with previous reports showing that specific binding to intracellular proteins occurs primarily in the subintimal space and determines arterial transport properties and microtubule binding of paclitaxel.21 As a consequence, the observed tissue half-life of paclitaxel delivered by DCB relates to the slow dissolution of paclitaxel deposits from the vessel surface into arterial tissue in a time-dependent fashion.22 Thus, the proposed mechanism of action reconciles the apparent contradiction between the observed short term supratherapeutic tissue levels seen right after balloon delivery and the resulting vessel healing profiles seen at the experimental level. However, the mechanism of action of DCB is likely technology dependent and likely will unveil several mechanistic pathways that will help the future development of DCB technologies.
Vessel healing largely depends on the pharmacokinetic features of each DCB technology. Bench data suggest that during balloon transit to the target lesion, approximately 10% to 15% of the drug is lost into the bloodstream. Also, at the time of balloon inflation, coating fragmentation occurs and paclitaxel particles are either transferred to the vessel wall or lost into the distal bloodstream (Fig. 32-4).16 Despite the high paclitaxel tissue levels achieved immediately after DCB delivery, tissue levels rapidly decline in 72 hours, and most of the paclitaxel remains attached to the surface of the vessel wall.4 After 7 days, paclitaxel tissue levels are comparable (although slightly higher) to the ones reported for DES.20
The vessel healing characteristics of arteries treated with DCB vary according to the presence or absence of stents. In the absence of the stent, the vessel healing profile is characterized by neointimal inhibition, presence of fibrin, and smooth muscle cell loss persistent over 90 days and resolving at 180 days.16 In contrast, the vascular healing profile of bare metal stents (BMSs) followed by DCB dilatation is similar to the one reported for paclitaxel-eluting stents (Fig. 32-5).23 Finally, a significant amount of emphasis has been put on the phenomenon of particulate embolization into the distal bloodstream. In vitro data have shown that the degree of particulate formation is significantly higher compared to DES. However, it has also been described that these particles are usually small and unlikely to be trapped into the distal microcirculation. Experimental data also suggest that microembolization is a rare finding and perhaps clinically irrelevant. However, due to the potential clinical adverse events derived from this phenomenon, the clinical introduction of these technologies for the treatment of specific critical vascular territories (eg, below the knee) has been slow and limited.
Although current-generation coronary DESs are considered to be standard of care,24 significant challenges still remain in specific clinical subsets in which DES outcomes are still suboptimal (eg, in-stent restenosis). In addition, DCBs have the potential to improve clinical outcomes in patients in whom the use of long-term dual antiplatelet therapy is limited (eg, bleeding risk). Thus, at present, the use of DCB has been limited to this specific population subset. In addition, although the use of DCB for the treatment of de novo lesions without the use of stents (“leaving nothing behind”) is theoretically appealing, the risk of abrupt vessel closure following balloon angioplasty and the high clinical success of DES have largely limited the introduction of this approach.
The first publication of DCB use in humans was described for BMS in-stent restenosis (ISR).5 In this multicenter study, 52 patients with coronary BMS-ISR were randomized to receive angioplasty with either an uncoated balloon or an iopromide-paclitaxel–coated DCB. At 6 months, the DCB group demonstrated a significant benefit in the primary end point of angiographic in-segment late lumen loss (LLL) (0.74 ± 0.86 vs 0.03 ± 0.48 mm; P = .002) as well as in the 6-month secondary end points of minimal lumen diameter (MLD) and binary restenosis. An additional 56 patients with coronary ISR were randomized, and the combined cohort of 108 patients was followed for 5 years, confirming the durability of the antiproliferative effect.25
The PEPCAD (Paclitaxel-Eluting Percutaneous Transluminal Coronary Angioplasty Catheter in Coronary Disease) trial program investigated the SeQuent Please DCB (B. Braun, Berlin, Germany) using the same iopromide-paclitaxel coating. PEPCAD II was a multicenter, randomized trial of the DCB versus the Taxus DES (Boston Scientific, Marlborough, MA) in 131 patients with coronary BMS-ISR.26 The primary end point of 6-month in-segment LLL was significantly less with the DCB compared with the DES (0.17 ± 0.42 vs 0.38 ± 0.61 mm; P = .03). At 12 months, target lesion revascularization (TLR) trended in favor of the DCB (6% vs 15%; P = .15), suggesting that the DCB was at least as effective as the DES for BMS-ISR and avoiding the need of a second DES implantation.26,27 Since then, 8 randomized trials have been published studying the SeQuent Please DCB in BMS-ISR25,26,28 and DES-ISR.29-34 A meta-analysis35,36 to assess the clinical efficacy of DCB for the treatment of DES-ISR from 5 studies29-34 including a total of 864 patients showed that DCB was superior to plain old balloon angioplasty (POBA) and comparable to first-generation DES for treatment of DES restenosis. Based on these trials, the 2014 European Society of Cardiology/European Association for Cardiothoracic Surgery guidelines on myocardial revascularization state (Class I, Level of Evidence A) that DCBs are clinically recommended for the treatment of ISR (within BMS or DES).24 Almalla et al37 reported that treatment of 86 patients with DES-ISR using the SeQuent DCB is associated with lower rates of major adverse cardiac events (MACE) and TLR at 1-year follow-up compared to second-generation DES (Xience V; Abbott Vascular, Santa Clara, CA). However, the latest results of the RIBS IV trial,38 which compared SeQuent DCB with the Xience Prime DES in 309 patients with DES-ISR, showed that patients treated with everolimus-eluting stents achieved superior 9-month LLL compared to DCB (everolimus-eluting stent 2.03 vs DCB 1.80; P = .004). At 1 year, freedom from TLR was higher in the patients treated with everolimus-eluting stents than in the DCB group (96% vs 87%; P = .008; Table 32-1). As a result, the use of DCB for DES-ISR needs to be considered on individual basis and the decision based on specific anatomic and clinical characteristics of each patient.
Authors | Trial Name | No. of Patients | PCB (no.) | BA (no.) | DES (no.) | Angiography FU | Clinical FU | Primary End Point | Angiography Results at FU | MACE Definition | Clinical Results at FU |
---|---|---|---|---|---|---|---|---|---|---|---|
Habara et al29 | 2011 | 50 | 25 | 25 | — | 6 months | 6 months | 6-month LLL | LLL: PCB 0.18 ± 0.45 mm vs BA 0.72 ± 0.55 mm, P = .001 | Death, MI, or TLR | TLR: PCB 4.3% vs BA 41.7%, P = .003; freedom form MACE: PCB 96% vs BA 60%, P = .005 |
Rittger et al31 | PEPCA-DES, 2012 | 110 | 72 | 38 | — | 6 months | 6 months | 6-month LLL | LLL: PCB 0.43 ± 0.61 mm vs BA 1.03 ± 0.77 mm, P < .001 | Cardiac death, MI, or TLR | TLR: PCB 15.3% vs BA 36.8%, P = .005; MACE: PCB 16.7% vs BA 50%, P < .001 |
Habara et al30 | 2013 | 87 | 53 | 34 | — | 6 months | 6 months | TVF | LLL: PCB 0.18 ± 0.38 mm vs BA 0.51 ± 0.52 mm | Death, MI, or TVR | Binary restenosis (%): PCB 9.1% vs BA 36.4% |
Byrne et al32 | ISAR-DESIRE 3, 2015 | 402 | 137 | 134 | 131 (PES, Taxus) | 6-8 months | 12 months-3 years | 6- to 8-month %DS | %DS: PCB 38% vs PES 37.4% (P = NS), vs BA 54.1% (P < .0001); LLL: PCB 0.37 ± 0.59 mm vs PES 0.34 ± 0.61 mm (P = NS), vs BA 0.70 ± 0.69 mm (P < .001) | Death, MI, or TLR | 3-year MACE: PCB 38.0% vs PES 37.7% (P = NS), vs BA 55.7% (P < .001); TLR: PCB 33.3% vs PES 24.2% (P = NS), vs BA 50.8% (P < .001) |
Xu et al34 | PEPCAD China ISR, 2014 | 215 | 109 | — | 106 (Taxus) | 9 months | 12 months | LLL | LLL: PCB 0.46 ± 0.51 mm vs PES 0.55 ± 0.61 mm (P = .32) | Death, MI, or TLR | TLR: PCB 16% vs PES 14% (P = .84); TLF: PCB 17% vs PES 18% (P = .69) |
Alfonso et al38 | RIBS IV, 2014 | 309 | 154 | — | 155 (EES, Xience) | 6-9 months | 12 months | 8-month LLL | LLL: EES 0.18 ± 0.6 mm vs PCB 0.30 ± 0.6 mm; P = NS | Death, MI, or TVR | Freedom from TLR: EES 96% vs PCB 87% (P = .008); freedom from MACE: EES 90% vs PCB 82% (P = .047) |
The initial concept for the use of DCB in coronary de novo lesions included the combination with a BMS to create a “polymer-free” DES. However, results from the PEPCAD III trial comparing such a BMS/DCB combination with the Cypher DES (Cordis, Hialeah, FL) showed this concept to be an inferior approach.39 Other concepts combining BMS and DCB as primary therapies have also shown similar results.40-44 These results have been partially explained by suboptimal percutaneous coronary intervention (PCI) technique (ie, geographic miss) and/or inferior DCB coatings.45,46 Nevertheless, even with optimized PCI techniques and more advanced coatings, the biologic effect of the DCB/BMS combination has proven to be clinically inferior compared to current-generation DESs.44,46 The PEPCAD I study was a nonrandomized study investigating the safety and efficacy of the SeQuent Please DCB with provisional BMS implantation in small vessel (mean reference vessel diameter, 2.36 mm) de novo lesions in 120 patients.48 At 6 months of follow-up, in-segment LLL was significantly less with DCB alone compared with DCB plus BMS (0.18 and 0.73 mm, respectively), with the majority of the ISR being noted at the stent edges. In patients with adjunctive BMS implantation, geographical miss was thought to be a strong predictor of the occurrence of future ISR.48,49
As a consequence of these findings, the so-called “DCB-only” strategy has been favored for the coronary use of DCB technologies. This concept includes careful lesion preparation, and depending on the result after predilatation, the operator can decide whether to proceed with DCB only in case of an acceptable angiographic result or use a stent or scaffold in case of major dissection (type C or higher), significant residual stenosis, or reduced flow. This approach aims to avoid the use of unnecessary stents and shorten the duration of dual antiplatelet therapy (~4 weeks in DCB-only procedures).50,51 This strategy was successfully investigated in 2 large registries52,53 and the randomized BELLO study. In this trial, 182 patients with small coronary vessel disease were treated with either the IN.PACT Falcon balloon (Medtronic, Dublin, Ireland) applying the DCB-only approach or the Taxus stent. The primary end point of LLL at 6 months was significantly lower in the DCB arm (0.08 ± 0.38 vs 0.29 ± 0.44 mm; P = .001), demonstrating superiority of the DCB-only approach over DES in terms of angiographic end points.54 Three-year follow-up (N. Ruparelia, unpublished data, May 2015) has been recently completed in more than 90% of the patients and shows a lower incidence of MACE events in the DCB group compared with the paclitaxel-eluting stent group (14.4% vs 30.4%; P = .015). However, TLR rates in the DCB group were comparable to those in the paclitaxel-eluting stent group at 6 months, 1 year, and 3 years (4.4% vs 7.6%, P = .37; 4.4% vs 9.8%, P = .25; 6.7% vs 13.0%, P = .14, respectively). An interesting application is in the setting of ST-segment elevation myocardial infarction (STEMI). First results of the DEB-AMI (Drug-Eluting Balloon in Acute ST-Segment Elevation Myocardial Infarction) trial55 showed that in STEMI patients, DCB followed by BMS implantation failed to show angiographic superiority to BMS only. Six-month angiographic (LLL: DES 0.21 ± 0.32 mm vs BMS 0.74 ± 0.57 mm and DCB+BMS 0.64 ± 0.56 mm; P < .01) and clinical results of DES (MACE: DES 4.1% vs BMS 23.5% and DCB+BMS 20.0%; P = .02) were superior to both BMS and DCB. In a recent report, Camaro and colleagues (unpublished data, May 2015) assessed the angiographic and clinical outcomes of the use of DCB in the STEMI setting. In this study, a total of 130 patients were randomly assigned to predilatation with a paclitaxel-coated balloon (PCB) versus POBA, followed by implantation of a Genous endothelial progenitor cell capture stent (OrbusNeich, Fort Lauderdale, FL; the DEBORA 2 randomized trial). At 9 months, the mean minimal lumen late loss was 0.18 ± 0.60 mm in the DCB group and 0.55 ± 0.52 mm in the POBA group (P < .01). No significant difference with regard to MACE was observed between groups (8.1% vs 9.5%; P = .773) at any time interval of clinical follow-up to 12 months. Finally, although the potential favorable vascular effects of the DCB-only strategy (eg, positive remodeling) have been shown in small observational studies,56 the lack of prospective comparative data has limited DCB use to specific clinical applications at the present time.
In contrast to the coronary field, peripheral vascular interventions are less dependent on the use of metallic stents. In long femoropopliteal lesions, the regular use of metallic stents has resulted in high mechanical and clinical failures rates.57 In addition, the treatment of ISR is a major clinical problem with no clear therapeutic alternatives. Thus, the use of DCB in the peripheral territory has gained more traction compared to the coronary field. However, the most important limitation of DCB use in this vascular territory is the potential for suboptimal angiographic results and dissections, leading to the use of “spot” metallic stenting. Although the use of DCB for de novo lesions has been clinically validated, the combination with BMS for this particular application is less clear. In addition, the use of adjunctive atherectomy and other lesion preparation techniques has also been proposed as a way to reduce the incidence of adjunctive stenting and enable the wider use of DCB in this particular vascular territory.
Several first-in-human randomized trials using different DCB technologies in femoropopliteal lesions have validated the safety and efficacy of this technology compared to POBA (Fig. 32-6).6,7,58-61 A meta-analysis including 381 patients (DCB, n = 186; POBA, n = 195) and using TLR as the primary end point has been recently published.62 At a median follow-up of 10.3 months, DCB use versus POBA reduced TLR (12.2% vs 27.7%; odds ratio [OR], 0.22; 95% confidence interval [CI], 0.13-0.38; P < .00001), angiographic restenosis (18.7% vs 45.5%; OR, 0.26; 95% CI, 0.14-0.48; P < .0001), and 6-month LLL (–0.05-0.50 mm vs 0.61-1.7 mm; mean difference, –0.75 mm; 95% CI, –1.06 to –0.45; P < .00001). In addition, the first DCB study performed in the superficial femoral artery (SFA) territory (THUNDER) has already reached 5-year follow-up demonstrating durability of the drug effect (TLR: DCB 21% vs POBA 56%; P = .0005).63
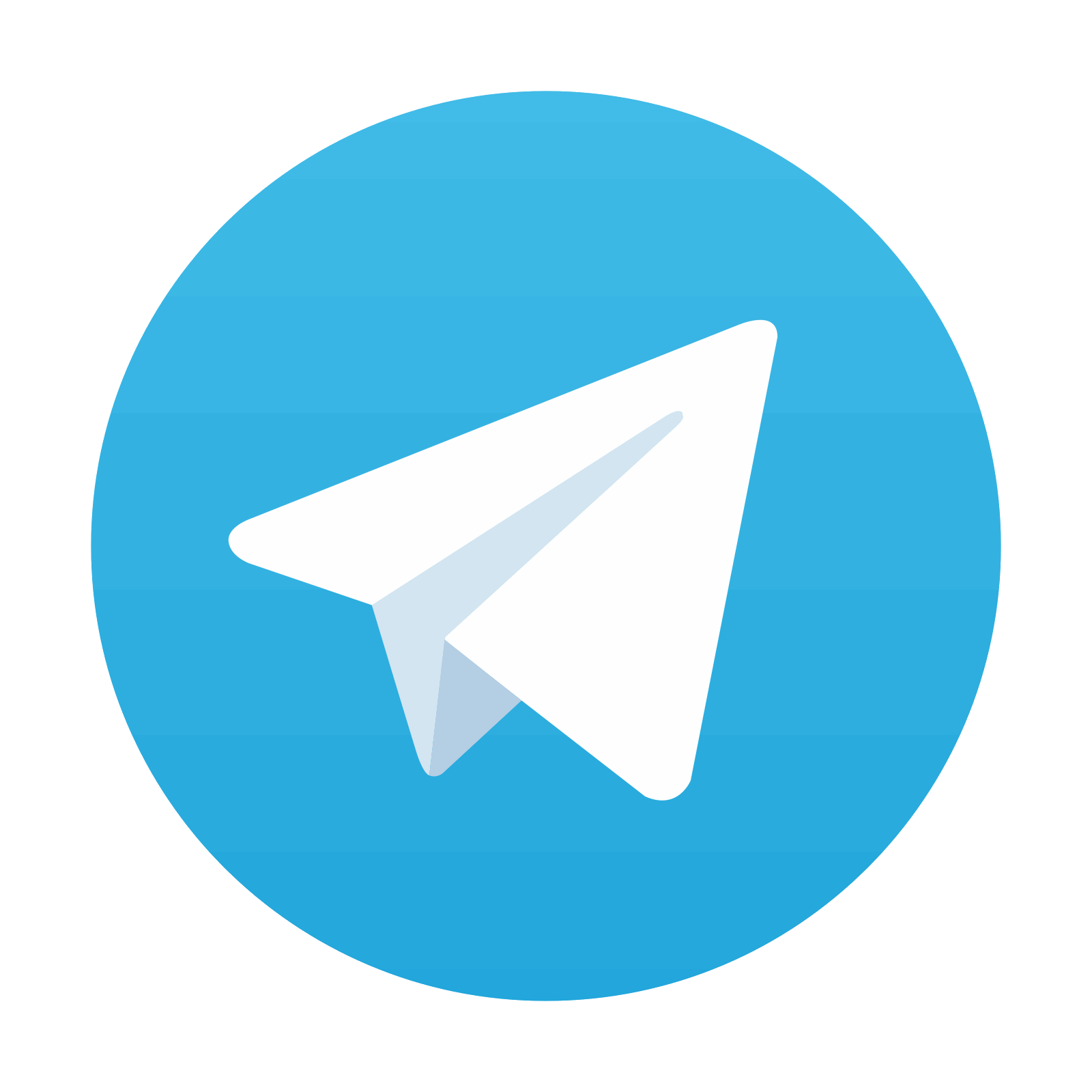
Stay updated, free articles. Join our Telegram channel

Full access? Get Clinical Tree
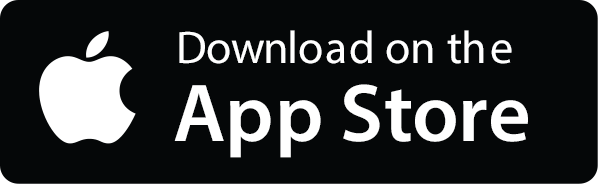
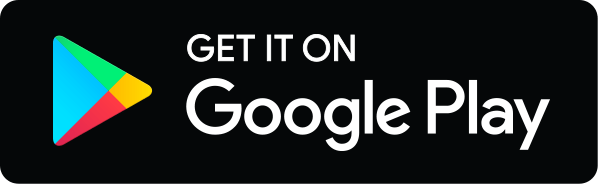