, Rohit Arora3, 4, Nicholas L. DePace5 and Aaron I. Vinik6
(1)
Autonomic Laboratory Department of Cardiology, Drexel University College of Medicine, Philadelphia, PA, USA
(2)
ANSAR Medical Technologies, Inc., Philadelphia, PA, USA
(3)
Department of Medicine, Captain James A. Lovell Federal Health Care Center, North Chicago, IL, USA
(4)
Department of Cardiology, The Chicago Medical School, North Chicago, IL, USA
(5)
Department of Cardiology, Hahnemann Hospital Drexel University College of Medicine, Philadelphia, PA, USA
(6)
Department of Medicine, Eastern Virginia Medical School Strelitz Diabetes Research Center, Norfolk, VA, USA
Heart Rate Variability Failed and Has Been Fixed
Background
The 1996 Circulation and European Heart Journal contained articles [1, 2] that standardized HRV measures, including spectral, statistical, and time-domain analyses of HRV. The articles also reference some nonlinear analyses. The article “Heart rate variability: Standards of measurement, physiological interpretation, and clinical use” in Circulation opens by stating: “The apparently easy derivation of this measure has popularized its use. As many commercial devices now provide an automated measurement of HRV … a seemingly simple tool. However, the significance and meaning of the many different measures of HRV are more complex than generally appreciated, and there is a potential for incorrect conclusions and for excessive or unfounded extrapolations” [1, p. 1043, 2].
The final statement continues to be profoundly prophetic. The “Background” section of the standard article cites the foundational work for P&S monitoring (spectral analysis of respiratory activity with concurrent spectral analysis of HRV): “In 1981, Akselrod et al. [3] introduced power spectral analysis of heart rate fluctuations to quantitatively evaluate beat-to-beat cardiovascular control” [1, p. 1043, 2].
Heart Rate Variability Alone
FRF
All measures based solely on HBI analyses (HRV-alone or btbBP) are mixed measures of autonomic activity. They provide no additional information, but merely assess whether your patient’s ANS is functioning. You knew that already – they are breathing!
HRV-alone monitoring is analogous to checking your patient’s heart and not checking their lungs.
The HRV standard articles state that spectral analysis is the only way to assess both branches of the ANS [1, 2]. However, measures based solely on the HBI (HRV alone or btbBP) are but one measure of the ANS and therefore, according to basic mathematical principles, cannot independently or simultaneously assess P&S activity. All HBI-alone measures require assumption or some other additional information. This is supported by the standard articles’ statements that “Vagal activity is the major contributor to the HF component” and that “LF as reflecting both sympathetic activity and vagal activity.” And in another statement they state: “It is important to note that HRV measures fluctuations in autonomic inputs to the heart rather than the mean level of autonomic inputs. Thus, both autonomic withdrawal and saturatingly high level of sympathetic input lead to diminished HRV” [1, p. 1051, [2, 4].
Spectral Analysis
Spectral analysis of HBI often involves the fast Fourier transform (FFT). This results in several HRV-alone measures as defined in the standard articles: ultralow frequency (ULF), very low frequency (VLF), low frequency (LF), and high frequency (HF). What the ULF and VLF parameters measure are still largely unknown, but it comprises about 95 % of the total spectral power [1, 2].
The effect of respiratory activity on the HRV spectrum is depicted in the next two figures. Respiratory activity is measured by spectral analysis, which indicates the frequencies of respiratory sinus arrhythmia over the period of observation. Again, the modal (or average) frequency of respiratory sinus arrhythmia indicates the center frequency of the parasympathetic activity as measured by RFa. This includes the parasympathetic activity in the LF range, as opposed to the standard which assumes the parasympathetic activity is only in the HF range and that the sympathetic activity is assumed to only be in the LF range. The separation of P&S activity into HF and LF bins does not always occur, as evidenced in Figure 3.1 showing the spectrum in a single slice in time from 32 s of a normal subject at rest, breathing at 0.15 Hz, which is nine breaths per minute. There is a similar figure in Dr. Low’s second edition of Clinical Autonomic Disorders [7, p. 326]. The bottom graph is the respiratory activity (RA) spectrum and the top graph is the HRV spectrum. Note that at this breathing rate, the high-frequency (RFa, respiratory frequency area) and low-frequency (LFa, low-frequency area) peaks are fairly well differentiated. However, breathing at this rate may be artificially high for the patient and is not representative of the true parasympathetic activity. In fact, the more artificially high the rate, the more the sympathetics will be stimulated, causing false positives for the SE and false negatives for the parasympathetic insufficiency.


Fig. 3.1
A time slice from the time–frequency analyses of heart rate variability (HRV) with concurrent analyses of continuous respiratory activity (RA). The peak mode of the (lower) respiratory power spectrum is the “fundamental respiratory frequency,” or FRF [5]. The FRF follows the patient’s breathing activity and is the center frequency of the parasympathetic measure RFa (respiratory frequency area). The remaining portion of the low-frequency range is the sympathetic measure LFa (low-frequency area). There is a similar figure in [6, p. 326]
The effect of a broad range of respiratory frequencies is depicted in Fig. 3.2. Figure 3.2 shows the time–frequency RA and HRV spectral data from the same patient performing a series of paced breathing challenges from 1 s per breath (1.0 Hz) to 20 s per breath (0.05 Hz). Each data slice along the time axis represents 32 s. The upper graph shows the variation in respiratory frequency over time. The effects of changes in the respiratory frequency over time on the HRV frequency content are demonstrated. Notice two main features: (1) how the ridge of peaks that are the LF content stays consistent along the breathing rate axis (breaths per minute or left-hand margin of the plot) and (2) how the ridge of peaks that are the HF content (driven by respiratory activity) mirrors the changes in the ridge of peaks in the respiratory activity power spectrum in the upper plot. Further, notice how the HF content merges with the LF content as the respiratory frequency approaches 0.15 Hz (9 breaths per minute, or 6 2/3 s per breath). This is the reason for the need to (artificially) maintain the breathing rate over nine breaths per minute in HRV-alone assessment to attempt to approximate and differentiate the parasympathetic from sympathetic activity. Of course, the (artificially) higher breathing rate may also accentuate sympathetic activity if the higher breathing rate causes hyperventilation. There is a similar figure in Dr. Low’s second edition of Clinical Autonomic Disorders [7, p. 326].


Fig. 3.2
Time–frequency spectra for concurrent respiratory activity (RA, top) and HRV (bottom) analyses. Note the way the RA peaks are reflected in the HRV spectrum. There is a similar figure in [6, p. 327]
P&S Monitoring, by incorporating Respiratory Activity analysis with concurrent HRV analysis, checks both the heart and the lungs. P&S Monitoring provides more information to guide therapy and document improved patient outcomes.
Speculation regarding what the ULF frequency range (≤0.003 Hz) of the HRV spectrum represents includes that it is a mixed measure of arterial contraction and P and S activity. Speculation regarding what VLF frequency range (0.003–0.04 Hz) of the HRV spectrum represents is that it is a mixed measure of baroreceptor reflex activity as it affects beat-to-beat BP and P and S activity. The LF (0.04–0.15 Hz) parameter is considered to be a measure of sympathetic activity as modulated by the parasympathetic activity. The HF (0.15–0.40 Hz) parameter is considered to be a measure of the parasympathetic activity as long as the respiratory frequency is high (>0.15 Hz). The LF/HF ratio is thought to reflect mostly sympathetic modulation during postural change [1, 2].
By definition, all of the spectral domain HRV-alone parameters, but the HF term, include the components of P and S activity. This is due to the fact that the frequency of sympathetic activity is limited to frequencies < 0.15 Hz. In other words, the reaction time of the SNS is limited to a maximum of three to five heartbeats. Although the PSNS is able to act faster than the SNS [3] (as fast as one to two heartbeats, or < 0.4 Hz), it does not mean that it always acts faster. (A faster PSNS enables the PSNS to prevent an SNS-induced tachyrhythm from turning into fibrillation or becoming worse.) So just like the sympathetic activity, the parasympathetic activity is also included in ULF, VLF, and LF, as well as HF [3]. Therefore, except for HF, all spectral analysis measures represent a mixture of P and S activity. None provide unambiguous measures of the sympathetic activity. Furthermore, the HF parameter is only a measure of the parasympathetic activity if the subject’s respiratory frequency is >0.15 Hz [1, 2]. In addition, the HF region, being fixed, is necessarily wide to ensure that it can capture the parasympathetic activity regardless of the level of respiratory frequency. The rest is considered to be noise, including harmonics from LF and lower frequencies. This indicates that the HF term is also mixed. A direct comparison between HRV-alone and P&S monitoring methodologies is presented in peer-reviewed publications [8–10] and numerous abstracts [11–22].
Statistical Analysis
Some of the more common statistical analyses of HRV, also known as time-domain analyses of HRV, are sdNN, rmsSD, pNN50, rangeHR (max–min HR), and max/minHR. The sdNN is the standard deviation of the normal-to-normal or beat-to-beat intervals. Since variance is mathematically equal to the total power of spectral analysis, sdNN reflects all the cyclic components responsible for HRV in the period of recording. While sdNN is not a well-defined quantity, sdNN is best correlated with the total spectral power [Table 3 in 1 or 2], not LF, as commonly assumed. Therefore, sdNN is a mixed measure of P and S activity.
The rmsSD is the root mean square of the standard deviation. The pNN50 is the percent of the beat-to-beat intervals that are greater than 50 ms. Both are measures of more or less HR variability. High-frequency activity causes variability. Therefore, more or less variability indicates more or less parasympathetic activity. “All of these measurements of short-term variation estimate HF variations in heart rate” and therefore contain no sympathetic activity information [1, 2]. However, these are thresholded, relative, qualitative parameters that do not trended well clinically.
RangeHR and max/minHR are measures of the total HRV and therefore are more correlated with sdNN. Total HRV is a gross measure of ANS activity, indicating whether the ANS is working. This provides little additional clinical information. Again, independent simultaneous measures of P&S activity are required to titrate therapy (e.g., beta-blockers or antidepressants) and document patient responses to therapy, treatment, disease, disorder, injury, lifestyle, or genetics. They are useful, however, in automatically determining the presence of ectopic activity in the EKG trace. P&S monitoring utilizes rangeHR to indicate the presence of high-quality ectopy (possible arrhythmia). Normal ranges for rangeHR at rest and for DB and PC are between 15 and 50 bpm, and for Valsalva, the range is between 15 and 60 bpm. A rangeHR greater than 50 or 60 is indicative of possible high-quality ectopy.
Nonlinear Analyses
It has been suggested that healthy hearts show chaotic variability and fractal fluctuations that may be broken by disease [23]. Nonlinear analyses describe long-term regulation mechanisms and require longer data sets. Nonlinear methods are far less developed and more complex than the linear methods. There are problems with their theoretical foundations and their practical applications. They are sensitive to initial conditions and dynamic changes in physiology and pharmacology, therefore requiring a long period of stability before analyzing the patient’s condition. This is similar to the signal stationarity requirements of Fourier transforms. Nonlinear analyses are dependent on prior knowledge of disease state and patient subpopulation to achieve the required specificity [1, p. 1050, 2]. Anecdotally, evidence suggests that nonlinear analyses provide, at most, a 10 % improvement in specificity and sensitivity for a well-known and highly specified subpopulation of patients. For general clinical applications, this is hardly worth the extra time and effort required to acquire these measures of autonomic activity.
Cardiovascular Autonomic Neuropathy (CAN) is very low P activity, and is associated with:
Low ejection fraction,
Poor cardiac output,
Arrhythmias,
Sudden Cardiac Death;
Congestive Heart Failure
Cardiomyopathies,
Poor circulation, including Angina,
Greater morbidity, and
A 50 % increase in the 5 yr mortality rate
Very low P activity with high SB often requires an ICD as therapy.
HRV-alone cannot assess this condition. – DePace, et al., 2012
Time-Domain Ratios
The time-domain ratios include the E/I ratio, Valsalva ratio, and the 30:15 ratio. These ratios are measured during the DB challenge, Valsalva challenge, and head-up PC (stand) challenge, respectively. These challenges are known as the Ewing challenges [24–26]. The DB challenge is paced breathing at six breaths/min for 1 min and is a parasympathetic stimulus. The Valsalva challenge is a series of five short (≤15 s) Valsalva maneuvers and is a sympathetic stimulus. You may remember that the Valsalva maneuver is a parasympathetic challenge. This is true for longer Valsalva maneuvers (>20 s). The head-up PC challenge is a net sympathetic challenge. From an autonomic perspective, the head-up PC challenge stimulates both P and S branches, providing insight as to how well the two branches coordinate, including while standing to prevent dizziness or lightheadedness.
The E/I ratio is the HBI during peak exhalation (“E”) divided by the HBI during peak inhalation (“I”) during the DB challenge. The Valsalva ratio is the longest HBI during a 15 s Valsalva maneuver divided by the shortest. The 30:15 ratio is the 30th HBI (approximately 30 s after a quick PC) divided by the 15th HBI (approximately 15 s after a quick PC). All ratios are always greater than one. Clinical findings show that the higher the ratio, the better [26]. Higher ratios indicate more HRV. Since it is known that sympathetic activity is low frequency limited and more variability results from higher-frequency components, it is therefore a fundamental fact that more HRV is purely a function of more parasympathetic activity relative to sympathetic activity. This confirms the understanding that more parasympathetic activity is recommended, since it is associated with reduced mortality and morbidity [27, 28]. However, it is also understood that too much parasympathetic activity is associated with, among other disorders, depression [29] and that depression is associated with greater morbidity and mortality [30–32]. For example, depression is relatively common in patients with cardiovascular disease [33, 34]. In patients with coronary heart disease (CHD), depression is associated with a two to four times increased risk of cardiac mortality compared with patients with CHD who are not depressed [30–32, 35]. Depression is associated with increased risk of major adverse cardiac events (MACE) in unstable angina [36]. There is evidence that depression is associated with dysregulation of the ANS and hypothalamic–pituitary–adrenal axis, blood hypercoagulability, and increased inflammation [34, 37]. Without independent and simultaneous P&S monitoring, excess parasympathetic activity cannot be differentiated from normal parasympathetic activity in patients, resulting in missed diagnoses, inappropriate dosing, and poor outcomes.
While the Ewing challenges stimulate the two branches of the ANS as discussed above, the time-domain ratios are only qualitative, thresholded measures of the parasympathetic activity alone. Again, they are measures more or less HRV. Granted, more variability indicates more health, but this information is incomplete. Activity in the SNS is omitted. More or less HRV is based on the levels of respiratory sinus arrhythmia (RSA, the only arrhythmia you want). More or less RSA is solely a function of the vagus nerve (RSA is also known as the cardiovagal reflex). The vagus nerve comprises the majority of the PSNS outside the brain. Furthermore, more or less variability requires more or less high-frequency activity. Only the parasympathetics are able to operate at higher frequencies. The sympathetics are limited to lower frequencies [1, 2]. Therefore, more variability is uniquely a function of the parasympathetic activity, even during Valsalva and standing. Therefore, the time-domain ratios only provide qualitative, or relative, measures of more or less parasympathetic activity. They miss the sympathetics, and also they do not provide clinical trending information.
Difficulties with Time-Domain Ratios
The manuscript, Difficulties with Time Domain Ratios as Measures of Parasympathetic and Sympathetic Activity, first published here, is authored by Drs. A. I. Vinik, R. R. Arora, and G. L. Murray and is presented in its entirety.
Introduction
Time-domain ratios are HRV-alone measures often utilized to detect diabetic autonomic neuropathy (DAN) as an early indicator of cardiovascular autonomic neuropathy (CAN). Time-domain ratios include the exhalation–inhalation (E/I) ratio, Valsalva ratio, and the 30:15 ratio. The E/I ratio is from the DB or paced breathing challenge. While a relaxed patient breaths at six breaths per minute, the interval between the two heartbeats (HBI) at peak exhalation is computed. Similarly, HBI is computed for peak inhalation. The ratio of these two time intervals is the E/I ratio. The Valsalva ratio is computed as the ratio of the longest HBI to the shortest HBI during a fifteen second Valsalva maneuver. For the 30:15 ratio, the patient is asked to perform a rapid head-up postural change (PC; i.e., standing or tilt) followed by 5 min of quiet standing. The 30:15 ratio is then computed as the ratio of the HBI 30 s after the PC to the HBI 15 s after the PC. All three of these ratios indicate more or less variability in instantaneous HR (IHR), and normal is considered to be values higher than an age-dependent threshold.
Autonomic neuropathy may be described in terms of resting tachycardia, orthostasis, orthostatic tachycardia and bradycardia syndromes, hyperhidrosis, sleep apnea, exercise intolerance, silent ischemia, or silent myocardial infarction. These disturbances, if left untreated, usually proceed to CAN. CAN is a process characterized by progressive damage to the autonomic nerve fibers that innervate the heart and blood vessels, resulting in abnormalities in HR control, vascular dynamics, and organ function [27, 28]. The CAN process leads to CVD and early cardiac death. The American Diabetes Association and the American Association of Clinical Endocrinologists have included as part of the standard of care among diabetic patients ANS testing, shortly after diagnosis and “more than once per year” [38, 39] (p. S37), [40, 41]. CAN is marked by increased morbidity and mortality associated with progressive retinal degeneration, progressive kidney failure, and progressive heart failure (potentially including cardiomyopathy) [27, 28]. In this way, CAN leads to significantly reduced life expectancy, increased numbers of medications that must be taken, and increased hospitalization rates [42]. CAN, known as a “silent killer,” carries a 50 % mortality rate. In diabetic patients, CAN is associated with a 500 % increase in mortality over a 5-year period [42]. Aggressive disease management is recommended in these patients and is the goal of treatment [27, 28, 42]. Disease management, however, is often confounded by autonomic imbalance and the symptoms caused by the imbalance, leading to the addition of therapies that often exacerbate the primary disease. The establishment and maintenance of autonomic balance improves outcomes [42] and may enhance quality of life and reduce morbidity and mortality. CAN has been quantitatively defined as very low, resting, parasympathetic activity [6]. CAN indicates increased risk of sudden cardiac death. CAN, compounded by a relative sympathetic excess (SE), is associated with high risk of sudden cardiac death [43].
All three time-domain ratios are HRV-alone measures. By definition, they all are measures of the total autonomic activity as it relates to variations in IHR [1, 2]. In other words, they are mixed measures of P and S activity. Moreover, changes in any of the three time-domain ratios (including the Valsalva ratio) are qualitative, or relative, indices of changes in the parasympathetic activity. Again, by definition [6], the time-domain ratios are measures of more or less variation in IHR. More variation is caused by higher-frequency activity. Sympathetic activity is known to be low frequency limited [6]. However, the parasympathetic activity is not. Therefore, more (or less) higher-frequency activity of the PSNS causes more (or less) variation in IHR. As discussed in the previous paragraph, CAN and its association with increased morbidity and mortality and high risk of sudden cardiac death [44] require specific quantification of P and S activity. HRV-alone measures provide quantitative, or relative, measures of the total autonomic activity. Three patient examples are presented to illustrate the clinical impact of this difference.
Patient 144
Patient 144 is a 25-year-old, 5′4″, 170 lb female diagnosed with hypertension, asthma, fibromyalgia, and mastocytosis. The patient was prescribed 6.25 mg carvedilol bid after baseline P&S assessment. Improvements with medication include lower BP, decreased headaches, and increased HRV as indicated by the time-domain ratios (see Table 3.1). Typically, the Valsalva ratio is incorrectly regarded as a sympathetic index. It is known that hypertension is associated with high sympathetic activity [45]. If the Valsalva ratio were truly an index of sympathetic activity, Patient 144 should have demonstrated normal Valsalva ratio prior to treatment. This is not the case. Rather, her Valsalva ratio is low (see the top graph, Fig. 3.3) which, by definition [1, 2], indicates a relatively low level of the parasympathetic activity, substantiating the previous interpretation. Hypertension is associated with high sympathetic activity which may induce low parasympathetic activity. Carvedilol is a double cocktail, including two adrenergic (sympathetic) antagonists: an alpha-blocker and a beta-blocker. The normalization of the Valsalva ratio posttreatment (see the bottom graph Fig. 3.3) is a result of reducing sympathetic activity by sympathetic blockade, thereby normalizing parasympathetic activity, as indicated by the normalization of the Valsalva ratio. HRV assessment does not answer the question of whether too much P or S activity is demonstrated. The patient also presented with mastocytosis, asthma, and fibromyalgia. Since the parasympathetic activity is associated with growth, mastocytosis may be associated with abnormal parasympathetic stimulation of the mast cells. It is known that asthma (and COPD) patients are presenting with early CVD. From an autonomic perspective, CVD is associated with SE and asthma is associated with PE. Perhaps the low Valsalva ratio, indicating low parasympathetic activity, suggests SE is induced by beta-2 adrenergic agonist (bronchodilator). This may be an early indicator of (preclinical) CVD or hypertension. Independent P&S measures are needed to fully document this patient’s condition. Further, the pain from fibromyalgia may present as SE (associated with heightened pain sensitivity, tachycardia or hypertension, and nighttime sleeplessness) and PE (associated with fatigue, poor circulation, possible gastrointestinal upset, etc.), exacerbating the SE. While carvedilol helps to relieve SE associated with CVD, hypertension, and pain, it may exacerbate asthma and underlying PE associated with fibromyalgia. Since there are no known upper limits to the time-domain ratios, PE cannot be detected with time-domain ratios. Upon follow-up P&S assessment, Patient 144 demonstrated higher E/I and 30:15 ratios, indicating more relative parasympathetic activity. Therefore, the time-domain ratios alone do not help to titrate therapy specifically for the individual, thereby limiting overall management of the patient.

Table 3.1
Pre- and posttreatment time-domain ratio results for Patient 144
TD ratios | Pretreatment | Posttreatment |
---|---|---|
E/I ratio | 1.25 | 1.35 |
Valsalva ratio | 1.32* | 1.61 |
30:15 ratio | 1.24 | 1.51 |

Fig. 3.3
Pre- and posttreatment time-domain (Valsalva) ratio results for Patient 144
The P&S results are presented in Table 3.2. Borderline indications with symptoms are considered abnormal. Patient 144’s pretreatment results show three abnormal values : (1) her parasympathetic response to DB is borderline low, indicating the presence of autonomic dysfunction prior to autonomic neuropathy; (2) her parasympathetic response to Valsalva is borderline high, indicating PE; and (3) her sympathetic response to standing is borderline low, indicating SW. Patient 144’s DB response is displayed in the top graph in Fig. 3.4. The gray area indicates the age-adjusted normal ranges. The area between gray and green is borderline abnormal. Her PE in response to Valsalva is displayed in the middle graph in Fig. 3.4. Since Valsalva challenge is a sympathetic challenge, the parasympathetic activity is expected to decrease. Her parasympathetic response is a 385.3 % increase over rest (baseline). Due to the symptoms, this increase is near enough to the borderline region (400–600 %) to be considered abnormal. As discussed above, PE is associated with fibromyalgia. The bottom graph in Fig. 3.4 presents the patient’s P&S responses to PC (standing). The normal physiological parasympathetic response to standing (F) is a decrease from resting (A). This is represented by the vertical blue line down from “A” to “F.” The normal physiological sympathetic response to standing (F) is an increase from (A) of more than 120 % but less than 500 %. The bottom insert in Fig. 3.4 displays her low sympathetic response in response to standing, indicating SW. Patient 144’s BP response to standing is a 5 mmHg drop, indicating possible orthostasis. SW, also indicating poor blood volume and possibly poor circulation, may also exacerbate the symptoms of fibromyalgia.

Table 3.2
Pre- and posttreatment P&S results for Patient 144
P&S results | Bx S | Bx P | SB | DB P | V S | V P | PC S | PC P |
---|---|---|---|---|---|---|---|---|
Pretreatment | 1.94 | 0.95 | 2.04 | 34.07* | 61.88 | 4.61* | 2.22* | 0.26 |
Posttreatment | 1.65 | 1.56 | 1.06 | 72.08 | 71.03 | 5.41 | 1.83* | 0.74 |

Fig. 3.4
Patient 144’s pretreatment P&S monitoring challenge responses: DB, Valsalva, and PC
P&S monitoring posttreatment results include a normalization in the parasympathetic response to DB and to Valsalva. Her parasympathetic response to Valsalva is now a 246.8 % change from resting. These results support the relief of clinical symptoms. The relief is further documented in the changes in her resting responses. The effect of the therapy, carvedilol, is shown in the resting results (see Table 3.2). Her initial baseline or resting sympathetic (Bx S) response decreases as expected. This is accompanied by an increase in her resting parasympathetic (Bx P) response. Overall, her SB became closer to 1.0. Although SW upon standing persists, the magnitude of the sympathetic abnormality and the magnitude of the abnormal BP response are both decreased. Often, relief of SW is realized with proper daily hydration. P&S monitoring provides more details regarding the patient’s condition.
Patient 145
Patient 145 is a 41-year-old, 5′9″, 210 lb male diagnosed with autonomic dysfunction, dyslipidemia, and nonalcoholic steatohepatitis (NASH) syndrome. The patient was prescribed 25 mg nortriptyline bid after the baseline P&S assessment. Improvements with medication include decreased syncopal episodes (postprandial fainting). The time-domain ratio history is presented in Table 3.3. Fainting is associated with PE [45]. It is known that the PSNS uniquely innervates the gastrointestinal tract. Therefore, postprandial fainting may confirm PE. PE cannot be documented with time-domain ratios. The pretreatment HRV-alone assessment indicates the patient’s ANS is well maintained (time-domain ratios are all normal). Only from symptoms is autonomic dysfunction indicated. Low-dose nortriptyline, an anticholinergic, acts to reduce the parasympathetic activity. From the first posttreatment HRV-alone assessment (over a year earlier), Patient 145 demonstrates decreases in the Valsalva and 30:15 ratios and an increase in the E/I ratio. The E/I ratio change is counterintuitive. It suggests an increase in relative parasympathetic activity (again, higher time-domain ratios indicate more variability in IHR, which indicates more parasympathetic activity relative to sympathetic activity). The anticholinergic should have reduced this measure. The DB challenge (from which the E/I ratio is computed) is a model of patient responses after a large meal and prior to sleeping for the night. The fact that the E/I ratio increases may indicate that, while the symptoms have been relieved, P and S have not yet returned to balance. Without the sympathetic measure, this cannot be objectively confirmed. If the Valsalva ratio is considered a relative sympathetic measure (which it is not), the small decrease in the Valsalva ratio is also counterintuitive. As argued above, given that the Valsalva ratio is a relative parasympathetic measure, then there is no confusion, except perhaps to suggest that a greater decrease may have been expected. The low 30:15 ratio results (see Fig. 3.5) from the first posttreatment study correlate with the expected outcomes from an anticholinergic. After a year on the therapy, the E/I ratio is slightly lower than prior to treatment, and the Valsalva and 30:15 ratios have rebounded and are significantly higher than pretreatment. Without a knowledge of sympathetic activity, these HRV-alone results seem to indicate that Patient 145 has had his autonomic balance restored and perhaps may be weaned from the therapy (if a follow-up study shows stability; if not, perhaps reduce therapy to maintenance dosing). More specific P&S measures would enable these types of therapy options. The patient also presented with autonomic dysfunction, dyslipidemia, and NASH syndrome. Specifying the autonomic dysfunction is not possible with time-domain ratios. Neither dyslipidemia nor NASH syndrome has known autonomic implications. Therefore, further insight into autonomic dysfunction is not possible with the HRV-alone information.

Table 3.3
Pre- and posttreatment time-domain ratio results for Patient 145
TD ratios | Pretreatment | Posttreatment | Follow-up |
---|---|---|---|
E/I ratio | 1.22 | 1.28 | 1.18 |
Valsalva ratio | 1.45* | 1.37 | 1.62 |
30:15 ratio | 1.35 | 1.05* | 1.45 |

Fig. 3.5
Patient 145’s low 30:15 ratio results from the first posttreatment study. This correlates with the expected outcomes from an anticholinergic
Patient 145’s P&S monitoring results confirm syncope. The top graph in Fig. 3.6 presents the parasympathetic (blue) and sympathetic (red) trend data for the P&S study. The six phases of the study, as indicated by the letters across the top of the graph, are as follows: A) resting baseline (Bx, 5 min), B) deep breathing (DB, 1 min), C) baseline (1 min), D) Valsalva challenge (1:35 min), E) baseline (2 min), and F) PC challenge (standing, 5 min). Typical normal responses for a 24-year-old subject are presented in the bottom graph in Fig. 3.6. From the trend plots in the top graph in Fig. 3.6, the patient’s resting baseline (A) response seems to be within normal limits. This is confirmed in the “A: Baseline” response plot (upper left graph) in Fig. 3.8. Again, the gray area indicates normal resting P&S activity. Normal SB is indicated in the area between the outer diagonal lines.


Fig. 3.6
Patient 145’s pretreatment P&S monitoring results confirm syncope. The top graph presents the parasympathetic (blue) and sympathetic (red) trend data for the P&S assessment study. Normal responses are presented in the bottom graph for a 24-year-old subject
Patient 145’s resting response (the point “A”) is in the middle of the gray area, confirming a normal resting response. This also indicates no autonomic neuropathy. Patient 145’s parasympathetic response to DB (section “B” in the patient’s trend plot, Fig. 3.6) also seems to be within normal limits. This is confirmed in the “B: Deep Breathing” response plot (upper right graph, Fig. 3.8). The DB response data are age adjusted, and the normal range is presented in the gray area. Between gray and green are the borderline areas. The patient’s DB response is borderline low. Patient 145’s sympathetic response to Valsalva (section “D” in the patient’s trend plot, Fig. 3.6) seems low. This is confirmed in the “D: Valsalva” response plot (lower left graph) in Fig. 3.8. The plot is similar in form to that for DB. The lower right graph in Fig. 3.8 presents the PC (stand) responses for Patient 145, averaged over 5 min. The averaging process often masks the instantaneous SE seen in the trend plot (see the top graph in Fig. 3.6; the peak (red) sympathetic response to PC (section “F”) is comparable or taller than that for Valsalva (section “D”); this is physiologically abnormal). In addition to the instantaneous findings (trend plot), the average PC responses suggest further abnormalities. In the case of Patient 145, the average parasympathetic activity increases abnormally from resting (A) to standing (F), indicating PE. Stand PE is confirmed in Fig. 3.7. Also from this figure, it may be argued that Patient 145’s parasympathetic response to Valsalva is borderline high, given his symptoms and a 390.8 % increase. From the PC response plot (lower right graph, Fig. 3.8), his average sympathetic activity decreases slightly over the 5 min interval, indicating (borderline) SW (associated with orthostasis). Together, the PE (indicating vagal excess) and the instantaneous stand SE (associated with syncope) indicate vasovagal syncope, with possible orthostasis, and an abnormal decrease in BP of 9/18 mmHg.



Fig. 3.7
The pretreatment parasympathetic responses from P&S monitoring of Patient 145

Fig. 3.8
The pretreatment response plots of the P&S monitoring results from Patient 145: (upper left graph) resting baseline P&S responses, (upper right graph) parasympathetic response to DB, (lower left graph) sympathetic response to Valsalva, and (lower right graph) P&S responses to PC (stand) challenge
During the first posttreatment test, Patient 145 demonstrates 81 arrhythmia beats, which inflated the resting, DB, and Valsalva responses (see the top graph in Fig. 3.9). Arrhythmia invalidates the time-domain ratio measures, even though the E/I and Valsalva ratios look normal (see Table 3.3). The effects of arrhythmia on the P&S monitoring results are to also inflate the numbers. This is the reason for the “not applicable” (n/a) indications in the posttreatment row of Table 3.4. However, the P&S monitoring SB results remain readable [46]. The patient’s SB is normal (normal SB, 0.4< SB <3.0), indicating that arrhythmia does not have an autonomic component. Based on the stand baseline results (section “E” in the top graph in Fig. 3.9), SW persists.


Fig. 3.9
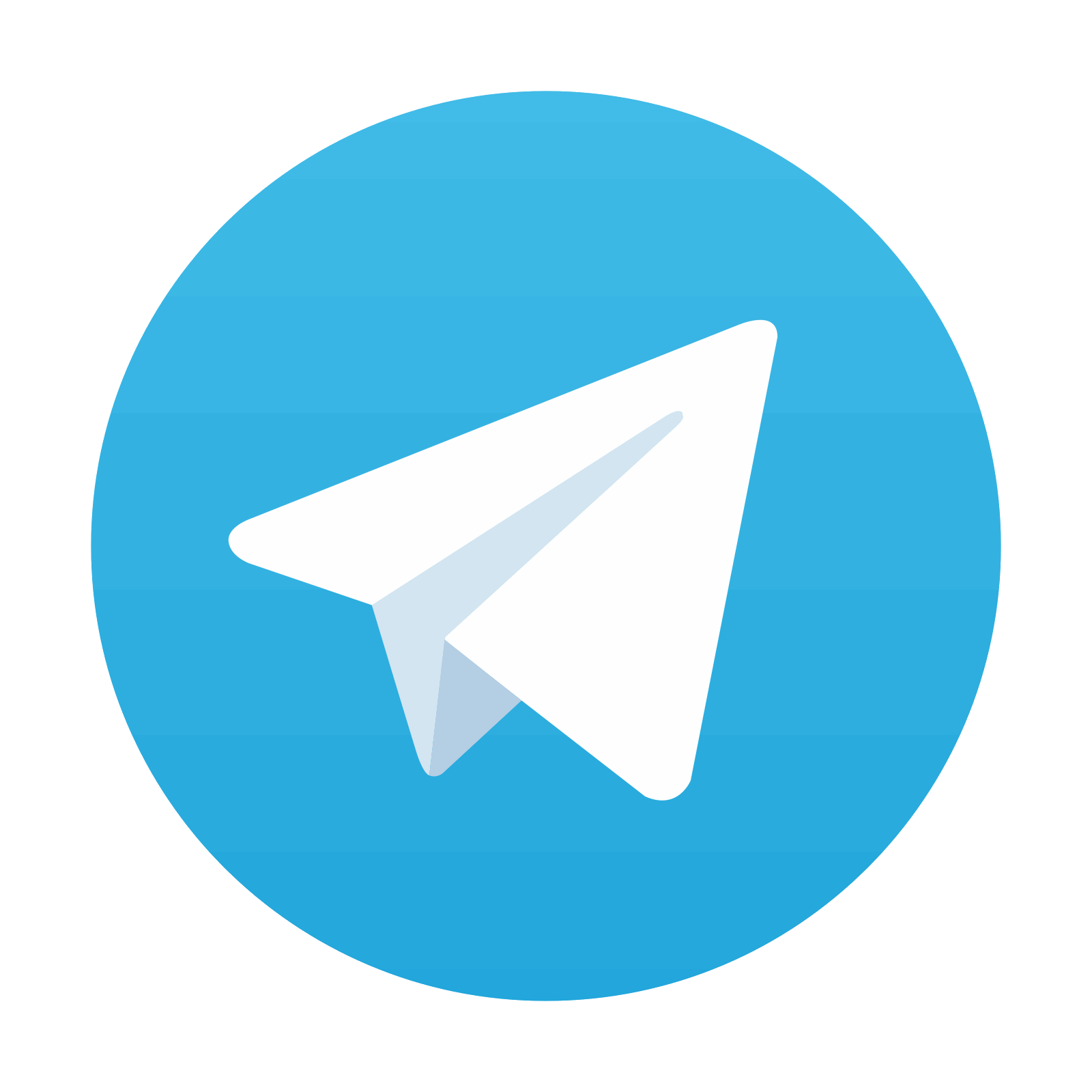
The first posttreatment and second posttreatment follow-up P&S assessment trend plot for Patient 145 is presented (top and bottom graphs, respectively)
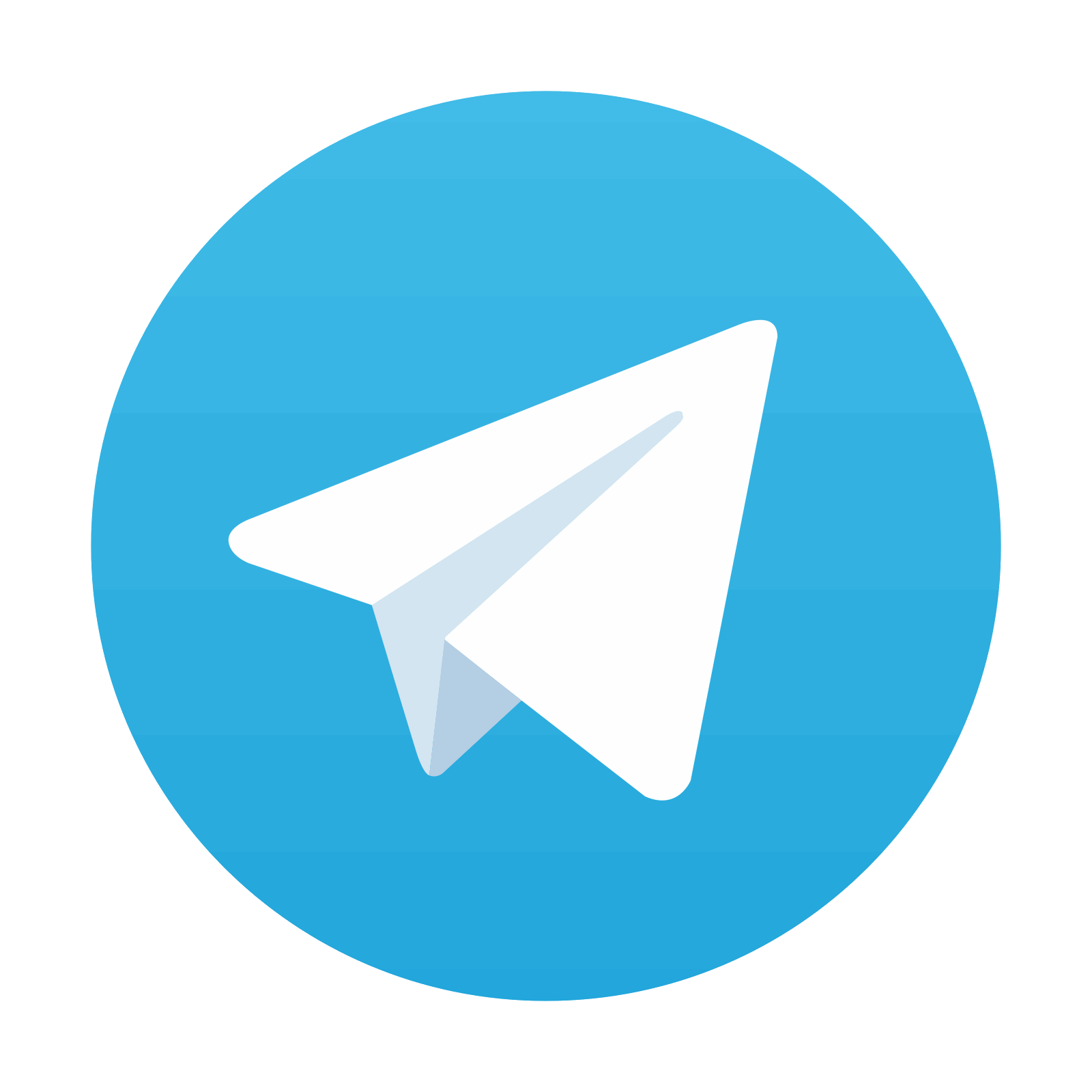
Stay updated, free articles. Join our Telegram channel

Full access? Get Clinical Tree
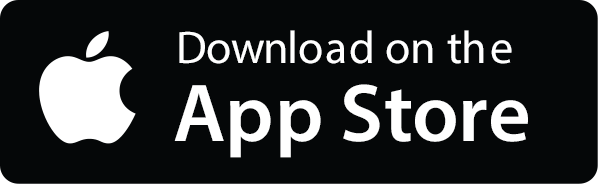
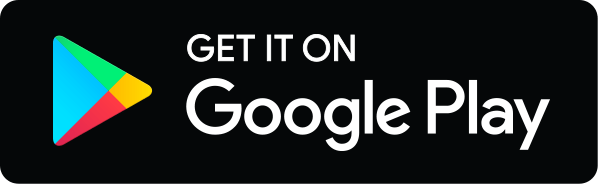
