A 51-year-old African American man presents to the emergency department (ED) with a chief complaint of dyspnea and swelling. He has a history of hypertension (HTN) and non-insulin-dependent type 2 diabetes. He works as a long-haul truck driver and reports not having seen a physician in over 3 years due to his work schedule and is no longer taking any home medications. He is saturating 93% on room air, has a pulse rate of 80 bpm, a blood pressure of 194/92 mm Hg, and a temperature of 36.5°C. Examination is remarkable for distention of the jugular veins, diffuse rales on inspiration, and 2+ pitting edema to midthigh. Initial electrocardiogram (ECG) shows left ventricular hypertrophy (LVH) by voltage criteria and left-axis deviation. A chest x-ray shows diffuse interstitial edema. Initial bloodwork is notable for a sodium of 135 mEq/L, creatinine of 1.1 mg/dL, albumin of 3.6 g/dL, hemoglobin of 12.9 g/dL, and brain natriuretic peptide (BNP) of 456 pg/mL. The patient receives oral hydralazine and intravenous (IV) nitroglycerin while in the ED with improvement of his blood pressure to 146/85 mm Hg, but he remains dyspneic with minimal exertion. A transthoracic echocardiogram reveals a hypertrophied left ventricle with moderately depressed left ventricular ejection fraction (LVEF) of 35% to 40% and grade II diastolic dysfunction.
Acute congestion from volume overload is a major driver of hospitalization in heart failure (HF). Loop diuretics remain the cornerstone of decongestive therapy.1,2
Figure 27-1 illustrates the principle behind diuretic therapy in acute HF. An initial clinical evaluation of patients with suspected acute decompensated heart failure (ADHF) should focus on determining fluid status and excluding cardiogenic shock.1
Figure 27-1
The principle of diuretic therapy in acute decompensated heart failure (ADHF). The acutely failing heart is functioning in the flat portion of the Frank-Starling curve, where small increases in blood volume result in large increases in left ventricular end-diastolic pressure (LVEDP) without significantly increasing cardiac output (CO). Inversely, decreasing the intravascular volume can significantly decrease the LVEDP and thus relieve congestive symptoms without significantly decreasing CO.

In general, patients with cardiogenic shock should be adequately stabilized prior to diuretic therapy.1 Patients with pulmonary edema despite relatively normal volume status have hemodynamic congestion (eg, hypertensive emergency, acute valvular pathology) but may still benefit symptomatically from adjunctive diuretic therapy, which exhibits acute venodilatory effects.1 Those patients who are clearly volume overloaded should receive loop diuretics to improve symptoms and functional status. Diuretics should be given intravenously for more rapid onset of action and predictable bioavailability.1
The diuretic strategies discussed in this chapter apply to both heart failure with reduced ejection fraction (HFrEF) as well as heart failure with preserved ejection fraction (HFpEF).
Commercially available loop diuretics include furosemide, bumetanide, torsemide, and ethacrynic acid. All except ethacrynic acid contain a sulfa moiety. Cross-reaction with allergies to other sulfa drugs is vanishingly rare, however. Ethacrynic acid, which is expensive and has more toxicity, should be used only in patients with documented anaphylaxis to the other loop diuretics.1,3
Regarding potency, 40 mg of IV furosemide is equipotent to20 mg of IV torsemide and 1 mg of IV bumetanide. Further comparisons are shown in Table 27-1.3,4 The site of action of all loop diuretics is the luminal side of the ascending loop of Henle. Here, the loop diuretics reversibly antagonize the sodium-potassium-2 chloride cotransporter, thus reducing sodium and chloride reabsorption and collapsing the interstitial sodium gradient that allows the kidney to concentrate urine. Because of this, it is the portion of the drug excreted unchanged that produces the desired diuretic effect.3,5,6
Figure 27-2 illustrates the sites and actions of several important transporters and receptors within the nephron relevant to diuretic therapy.
Figure 27-2
A diagram of the nephron with locations of relevant transporters. Organic acid transporter (OAT) complexes are found in the proximal tubule and are the main pathway by which diuretics enter the nephron. Carbonic anhydrase (CAH) in the proximal tubule is the target of acetazolamide. NKCCs (Na-K-2Cl cotransporters) in the ascending loop of Henle are the primary target of loop diuretics. Thiazide-sensitive Na-Cl (TSCs) symporters in the distal tubule are the primary target of thiazide diuretics. Mineralocorticoid receptors (MCR)/NaKs respond to aldosterone stimulation by upregulating the presence and activity of sodium-potassium ATPase in the collecting duct. Aldosterone antagonists and potassium-sparing diuretics such as amiloride target this system. V2R (vasopressin antagonists) target type 2 vasopressin receptors, which increase free water absorption through upregulating aquaporins in the collecting duct when stimulated.

The loop diuretics are highly bound to serum proteins. Active secretion of this protein-bound component by proximal tubule cells is the main source of active drug in the urine. Free drug is primarily metabolized to inactive metabolites by glucuronidation and very little enters the urine unchanged.5,6
Albumin-bound loop diuretic is unbound and secreted into the proximal tubule by the organic acid transporter, which also mediates renal excretion of urea and uric acid, lactic acid, and many other drugs (notably including nonsteroidal anti-inflammatory drugs [NSAIDs]) and endogenous compounds. High doses of loop diuretic may reduce the urinary excretion of these other compounds, and vice versa.5,6
The dose-response relationship for the loop diuretics is notable for the presence of an effective threshold dose to achieve diuresis. Doses below the threshold will produce essentially no diuresis, while those above the threshold will quickly hit an effective ceiling response, with further dose increases carrying no additional response.7
For a diuretic-naïve patient with normal renal function, a dose of 10 to 20 mg of IV furosemide is generally the threshold dose, and a maximum response is seen with 40 mg. As illustrated in Figure 27-3, the presence of HF tends to increase the threshold and ceiling doses. The presence of intrinsic renal disease will increase the threshold and ceiling doses, while decreasing the responsiveness to an effective dose.7,8
Figure 27-3
The S-shaped curve of the response to loop diuretics in different disease states. Black curve: A healthy patient. Yellow curve: A patient with chronic renal disease has a lower glomerular filtration rate (GFR), and thus has a lower ceiling on his or her response; this patient may also require a higher dose to achieve adequate concentration in the tubule. Cyan curve: A patient with chronic heart failure has increased levels of counter-regulatory hormones promoting salt and water retention, and thus requires a higher dose to respond. Magenta curve: A patient with cardiorenal syndrome has both a lower ceiling and a higher diuretic requirement to reach it.

Relevant clinical differences between loop diuretics are primarily related to bioavailability and will be discussed more in Part 2 of this chapter on outpatient diuretic therapy.
When given intravenously, the bioavailability of all loop diuretics is 100% and no significant therapeutic differences have been noted among them. As such, choice of IV diuretic should be driven primarily by local availability and cost.
For patients who are diuretic-naïve with normal renal function, an initial dose of 20 to 40 mg of IV furosemide or equivalent once to twice per day is generally chosen.
For patients who are on home diuretics, each IV dose should be numerically equal to or greater than their home dose. In a randomized controlled trial, patients receiving initial IV doses equal to 2.5 times their oral home dose showed a trend toward faster improvement compared to those receiving the IV equivalent of their home oral dose. Importantly, the high-dose group did not have a greater frequency of complications.9
IV diuretics should be given either as boluses or as a continuous infusion. A number of smaller trials have suggested reduced complications and improved diuresis associated with continuous infusion, but the larger and more rigorous DOSE trial did not show a difference between strategies.9 Both are considered equally valid approaches in the 2013 AHA/ACC guidelines. From a practical standpoint, doses of less than 200 mg of furosemide equivalent per day are usually more simply delivered as boluses. If individual bolus doses exceed 120 mg furosemide equivalent, or need to be given more often than twice daily, continuous infusion may be reasonably considered.10
A classically reported method of dose estimation suggests the use of patient age plus serum blood urea nitrogen (BUN) to calculate furosemide dose. Although this has not been clinically verified, it does underscore the role of glomerular filtration rate (GFR) in determining a patient’s sensitivity to diuretics and thus the counterintuitive need to give higher doses to patients whose initial serum creatinine is elevated.11
The frequency of systemic drug buildup and toxicity is exceedingly rare at the doses of loop diuretics used clinically. In general, bolus doses up to 200 mg and daily doses up to 400 mg will not result in acute or cumulative toxicity.5,6
There is no generalized agreement as to the ideal rate of volume removal. Most patients will tolerate 0.5 to 2 L of net volume removal per day. It has been hypothesized that more aggressive volume removal may lead to excessive renin-angiotensin-aldosterone system (RAAS) activation and worse outcomes, but this has not been reliably demonstrated in clinical trials. Diuretic goals should be tailored to the needs of the patient based on symptoms, degree of hypervolemia, and clinical stability.1,12
The patient should be closely monitored for response to therapy, with dosing adjustments made frequently if necessary.1
It is well documented that measured intake/output and bed weights are fraught with inaccuracies in the hospital environment. Therefore, monitoring of response should be approached holistically, combining intake/output measurements and daily standing weights (preferably on same scales each day) with careful examination and symptom assessment.13,14
The majority of the diuresis from an IV bolus of furosemide or bumetanide will occur within the first 2 half-lives of the drug(2-4 hours for furosemide and bumetanide) and will be effectively complete by 6 hours after administration. (Remember, Lasix = LAsts SIX.) Therefore, urine output should be monitored especially closely during this period of time to determine if an effective dose was delivered. An effective dose should produce at least 1 to 2 L of diuresis within this time frame and result in at least 50 mmol of natriuresis. With twice a day dosing, an effective dose will allow a negative fluid and sodium balance if the patient is maintained on a typical inpatient cardiac diet with a 2 L fluid restriction and 2 g (90 mmol) sodium restriction.15
Adequate diuretic response can be predicted within the first hour of diuretic administration by a spot test of urine sodium and creatinine, which can be used to predict sodium and volume output for the diuretic bolus according to the validated formulas and examples in Figures 27-4 and 27-5.15
Figure 27-5
Example of how to use the equations in Figure 27-4. Consider 2 seemingly identical patients, both given 40 mg IV furosemide. Urine from both patients is sampled 1 hour later, and predicted sodium and urine outputs calculated.

If a dose of diuretic fails to achieve a significant response, the subsequent dose should be increased. Remembering the short half-life of the loop diuretics and the need to achieve a threshold concentration in the urine, attempting to stack small doses over the course of several hours is rarely effective. For example, if the patient receives 40 mg of IV Furosemide and has not produced at least 500 mL of urine after2 hours, the patient should receive 60 or 80 mg for his or her next dose, rather than attempting to add an extra dose of 20 mg right away.
If the patient is achieving adequate response to each dose, but is not achieving net volume loss, then dosing frequency may be increased up to every 6 hours, or changed to continuous infusion at an equivalent rate. Additionally, the patient’s sodium and water restrictions should be reviewed to ensure they are adequate.
Be aware of the diuretic braking phenomenon: acutely increased delivery of sodium to the distal tubule of the nephron activates the RAAS causing efferent arteriolar constriction and a decrease in the renal perfusion. This in turn may acutely decrease diuretic sensitivity. An effective diuretic dose on inpatient day 1 may no longer be effective by day 2. Again, careful monitoring and dosing adjustment is required.
The potential risks and complications of diuretic therapy have been well documented and include electrolyte imbalance, arrhythmia, alkalosis, renal injury, hemodynamic instability, and gout flare. The majority of these risks can be minimized by careful monitoring.1
Patients undergoing IV diuretic therapy should be monitored on telemetry and have serum levels of sodium, potassium, magnesium BUN, and creatinine checked at least once per day. More frequent electrolyte checks may be appropriate if the patient is experiencing very brisk diuresis, has baseline abnormalities in renal function, or consistently shows labile electrolyte levels.1
Potassium and magnesium should be supplemented as necessary to maintain a serum potassium between 4.0 and 4.5 mEq/L and a serum magnesium >2 mEq/L.
Numerous influences may cause serum creatinine to rise during diuretic therapy, and not all of them necessitate discontinuation of therapy. Typical activation of the RAAS and competitive antagonism of the organic acid transporter during diuretic therapy can result in increases in serum creatinine and BUN without intrinsic renal injury. Worsening venous congestion may similarly reduce renal perfusion and cause increased creatinine (congestive nephropathy). However, it is only decreased renal perfusion or hypotension related to intravascular volume depletion that results in significant renal injury and that will respond to discontinuation of diuretics. Any change in creatinine must be carefully correlated with clinical findings. A rise in creatinine that occurs in a patient who is clinically volume overloaded and without documented heavy diuretic response within the last 24 hours is unlikely due to overdiuresis.16
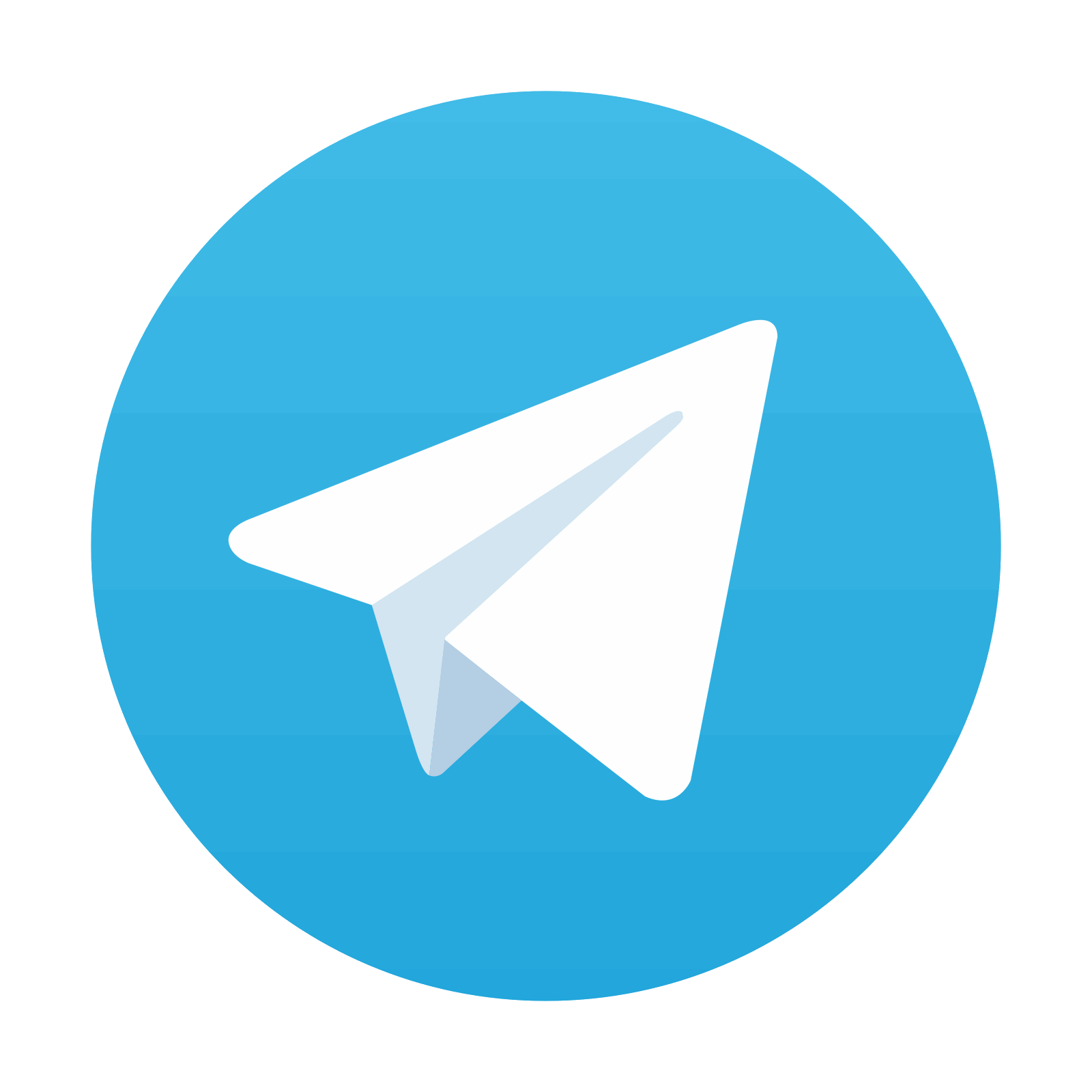
Stay updated, free articles. Join our Telegram channel

Full access? Get Clinical Tree
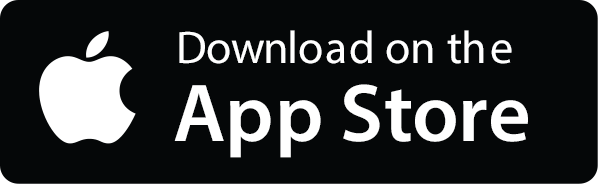
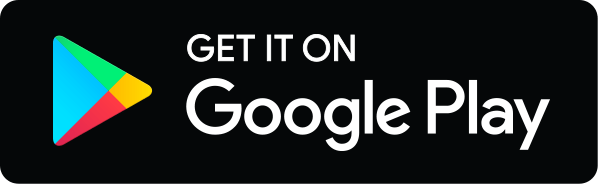