Distal Protection—Filter Devices
Jacqueline Saw
Jay S. Yadav
Deepak L. Bhatt
Technological advances and evolution in the twentieth century have enabled revolutionary percutaneous catheter-based treatments of cardiovascular atherothromboembolic diseases. Since the first experimentation of coronary angioplasty by Gruntzig in 1976 (1), percutaneous coronary interventions (PCI) have been avidly adopted worldwide. In the United States alone, over one million PCI were performed in the year 2000 (2). The growing experience and understanding of the limitations associated with balloon angioplasty (BA) fueled the development of devices to target vessel recoil, restenosis, and abrupt vessel closure. The subsequent introduction of coronary stents and atherectomy devices, together with broadening indications of PCI for more challenging thrombotic and saphenous vein graft (SVG) lesions, brought to light the importance of distal embolization and its association with periprocedural myocardial infarctions (MI). The significance of distal embolization extends beyond compromised epicardial patency to the interference of microvascular perfusion. Such mechanical disruptions not only cause myocardial necrosis of varying severity, but also induce platelet activation and inflammation. Therefore, both the pharmacologic and mechanical means of preventing distal embolization and its sequelae are under active investigation.
EMBOLIZATION USING PERCUTANEOUS CORONARY INTERVENTION
Although several etiologies exist for periprocedural MI related to PCI (side-branch occlusion, dissection, abrupt closure, etc.), distal embolization is undoubtedly the predominant cause. In recent studies, the incidence of sidebranch occlusions and abrupt closures was infrequent with PCI, <3% and <1% of patients, respectively (3,4). In contrast, embolization has been shown to occur with essentially all PCI modalities (5), during multiple stages of the intervention, and it is proportional to the invasiveness of the procedure and the degree of atherothrombotic burden. Yadav et al. (6) showed that atheroembolic debris was captured in emboli protection devices (EPD) among each of over 50 patients who underwent routine coronary angioplasty and stenting. The placement of coronary stents is associated with a slightly higher incidence of periprocedural MI compared with BA alone (4,7,8), which could be partially accounted for by the “cheese grater” effect (Fig. 13.1). In the study by Kini et al. (7), creatine kinase-MB (CKMB) elevation was more common with the use of nonballoon devices compared to angioplasty alone (19.5% versus 11.5%, p <0.01).
The use of debulking atherectomy devices, both directional and rotational coronary atherectomy, particularly predisposes to distal embolization, with periprocedural MI occurring at least twice as often (8,9). In an analysis of the Evaluation of c7E3 Fab in the Prevention of Ischemic Complications (EPIC) trial, the use of directional atherectomy was associated with roughly two times higher non-Q-wave MI incidence (9.6% versus 4.9%, p = 0.006) compared with BA (9). Fortunately, those who were randomized to abciximab had a 71% reduction in non-Q-wave MI when directional atherectomy was used. In another study, among 4,484 patients who underwent successful PCI, the use of directional atherectomy was associated with a 4.1 odds ratio of CKMB elevation (10).
The degree of plaque burden is also a major determinant of distal embolization. In the study by Kini et al., the presence of diffuse coronary atherosclerosis and systemic
atherosclerosis was a significant predictor of periprocedural CKMB elevation (7). The PCI of degenerative SVG depicts this concept perfectly, reflecting the high incidence of embolization as a consequence of massive plaque burden (11). Patients with acute coronary syndromes also harbor the pathological substrate that predisposes to embolization, where the presence of a disrupted fibrous cap and platelet thrombi promotes inflammation and downstream embolization.
atherosclerosis was a significant predictor of periprocedural CKMB elevation (7). The PCI of degenerative SVG depicts this concept perfectly, reflecting the high incidence of embolization as a consequence of massive plaque burden (11). Patients with acute coronary syndromes also harbor the pathological substrate that predisposes to embolization, where the presence of a disrupted fibrous cap and platelet thrombi promotes inflammation and downstream embolization.
PATHOPHYSIOLOGY OF EMBOLIZATION
The clinical manifestation of distal embolization constitutes a spectrum, ranging from epicardial occlusion, no reflow or slow flow (with epicardial patency), to asymptomatic cardiac enzyme elevation. This variability is dependent upon the quantity and size of the embolic particles (12) and subsequent platelet activation and inflammation. Pathologically, angioplasty and stenting mechanically disrupt the endothelium, exposing the subendothelial matrix and activating the cascade of platelet adhesion, activation, aggregation, and thrombus formation. As a consequence, small atherothrom-boembolic debris, which may consist of plaque or vessel-wall constituents (e.g., lipid, collagen, endothelial cells) and platelet-thrombi, can be liberated to distal vessels. The lodging of these embolic particles in downstream microvasculature not only causes microvascular obstruction, but creates a nidus for inflammation. It has been shown in an experimental canine model that microvascular occlusion disrupts endothelial integrity distally and upregulates P-selectin expression by endothelial cells, with subsequent adherence of platelets and leukocytes (13). The formation of such multicellular plugs, together with the activation of platelets releasing vasoactive amines (thus increasing vascular tone), further potentiates microvascular occlusion and causes myocardial necrosis, which manifests as cardiac enzyme elevation.
CLINICAL IMPORTANCE OF PERIPROCEDURAL MI
The measurement of cardiac enzymes (e.g., CK, CKMB, and troponin) following PCI has provided insight into the occurrence of distal embolization and the correlation to long-term clinical adverse events. It has been shown that CK or CKMB elevation occurs in 5% to 30% of patients following PCI (14). Although the threshold of cardiac enzyme elevation for periprocedural MI definition is not universally established, a CKMB elevation greater than 3 times the upper limit of normal is most commonly accepted (14). Troponin is a more sensitive marker of myocardial necrosis and has been shown to be elevated in 25% to 50% of patients following PCI (15, 16, 17).
Periprocedural MI following PCI has been associated with adverse long-term outcomes, including mortality. This was demonstrated in the landmark Evaluation of C7e3 for Prevention of Ischemic Complications (EPIC) trial (18): The greater the degree of postprocedural CK elevation, the higher the subsequent risk of mortality (Fig. 13.2) (19). The risk of death is at least doubled when CK elevation is more than 5 times the upper limit of normal. Other studies also have corroborated the significance of minimal cardiac enzyme elevation post-PCI (20,21). In a retrospective analysis of 4,664 patients who underwent successful PCI at the Cleveland Clinic, CK elevation between 2 and 5 times the upper limit of normal was associated with worse adverse long-term outcome. Specifically, CK elevation was associated with an increased risk of cardiac death (risk ratio 2.19, p = 0.0001) (20). In a smaller retrospective analysis involving 373 patients, CK elevation of as little as 1 to 3 times the upper limit of normal conferred an increased mortality risk at ˜3.5-year follow-up. For every 100 U/L increase in CK, the relative risk of cardiac mortality was 1.05 (95% confidence interval, 1.03 to 1.08) (21). Similarly, troponin elevation following PCI has been shown to correlate with higher mortality risk, especially with large elevations (≥8 times the upper limit of normal) (17,22).
STRATEGIES TO PREVENT DISTAL EMBOLIZATION
Given the frequency and significance of distal embolization, both pharmacologic and mechanical strategies to
mitigate microvascular occlusion and injury have been attempted. Pharmacologic therapies are discussed only briefly in this chapter. Intravenous GPIIb/IIIa inhibitors have been convincingly shown to reduce periprocedural MI and subsequent long-term mortality (23). In a pooled analysis of five abciximab placebo-controlled PCI trials, Bhatt et al. showed that abciximab reduced 30-day death or MI irrespective of the type of interventional device used (Fig. 13.3) (5). Pretreatment with the thienopyridines, ticlopidine and clopidogrel, also has been shown to reduce periprocedural MI (24, 25, 26, 27). Similarly, the use of a direct thrombin inhibitor, hirudin, in the setting of PCI for unstable angina patients, has been shown to reduce troponin release (28). Nevertheless, these agents are not completely protective against distal embolization, thus necessitating a combination strategy with a mechanical barricade. This is especially true during percutaneous interventions of vessels with large atheromatous and thrombotic burdens. In the setting of SVG interventions, the use of GPIIb/IIIa inhibitors has not been shown to lower periprocedural ischemic events in a pooled analysis of five randomized trials (29).
mitigate microvascular occlusion and injury have been attempted. Pharmacologic therapies are discussed only briefly in this chapter. Intravenous GPIIb/IIIa inhibitors have been convincingly shown to reduce periprocedural MI and subsequent long-term mortality (23). In a pooled analysis of five abciximab placebo-controlled PCI trials, Bhatt et al. showed that abciximab reduced 30-day death or MI irrespective of the type of interventional device used (Fig. 13.3) (5). Pretreatment with the thienopyridines, ticlopidine and clopidogrel, also has been shown to reduce periprocedural MI (24, 25, 26, 27). Similarly, the use of a direct thrombin inhibitor, hirudin, in the setting of PCI for unstable angina patients, has been shown to reduce troponin release (28). Nevertheless, these agents are not completely protective against distal embolization, thus necessitating a combination strategy with a mechanical barricade. This is especially true during percutaneous interventions of vessels with large atheromatous and thrombotic burdens. In the setting of SVG interventions, the use of GPIIb/IIIa inhibitors has not been shown to lower periprocedural ischemic events in a pooled analysis of five randomized trials (29).
Mechanical EPD can be divided into balloon occlusion and filter devices. The balloon occlusion devices can be further subdivided into proximal and distal occlusion types, which are fully discussed in the next chapter. Table 13.1 describes the potential advantages and disadvantages of filters versus occlusion devices. The remainder of this chapter focuses on filter EPD.
FILTER EMBOLI PROTECTION DEVICES
The prototypical filter device consists of a guidewire with an integral filter basket. When these devices are deployed, they allow antegrade blood flow through the filter pores, but trap those embolic debris that are larger than the pore size. Once deployed, the filter guidewire functions as a conventional angioplasty guidewire, accommodating balloons and stents with filter protection. The only filter EPD that is currently FDA approved is the EPI FilterWire EX device (Boston Scientific, Natick, Massachusetts) for SVG percutaneous interventions. Several other filter EPDs have been developed and are available under clinical trials of coronary, carotid, and peripheral interventions (Table 13.2).
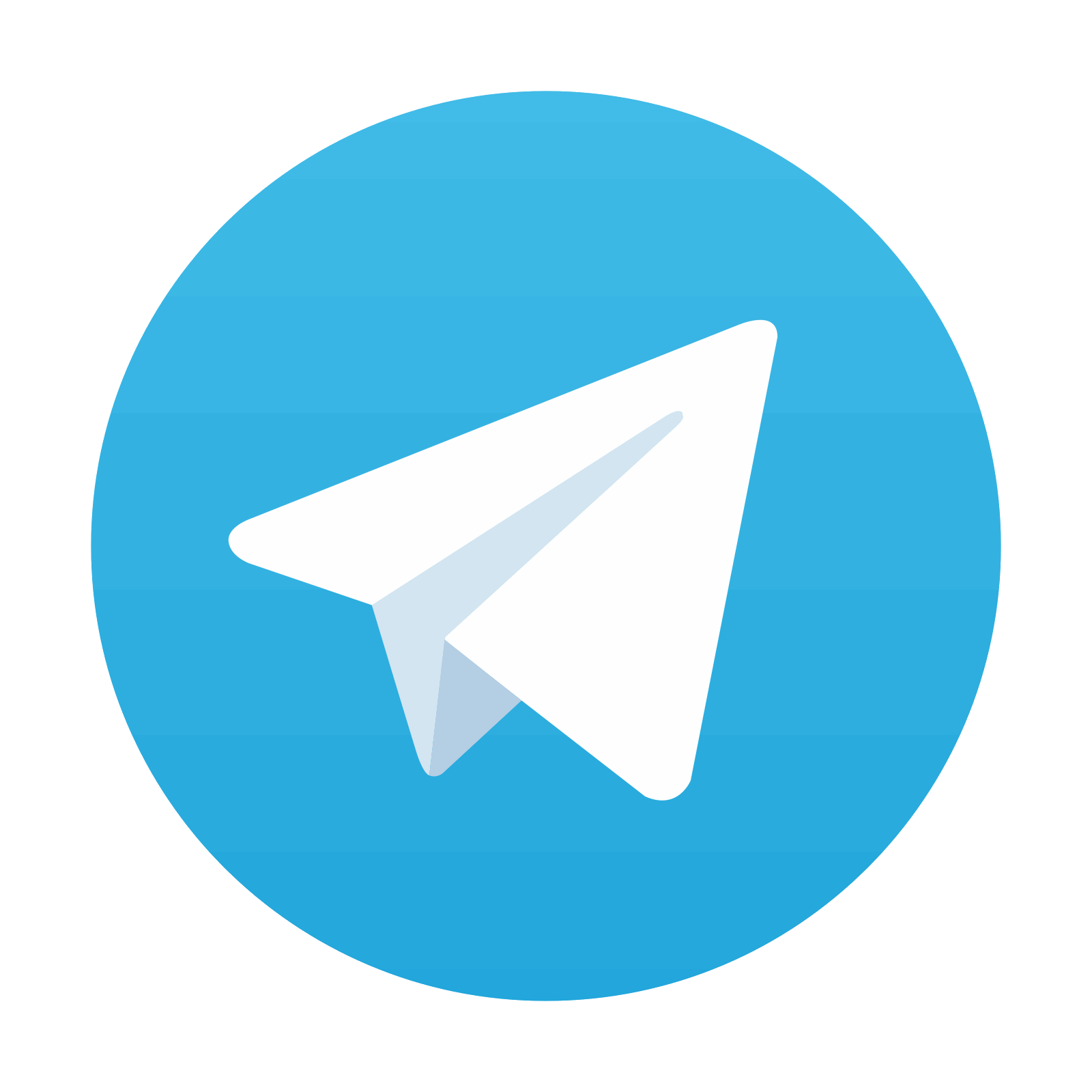
Stay updated, free articles. Join our Telegram channel

Full access? Get Clinical Tree
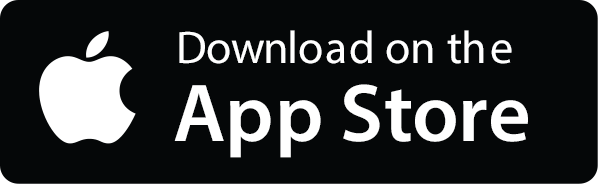
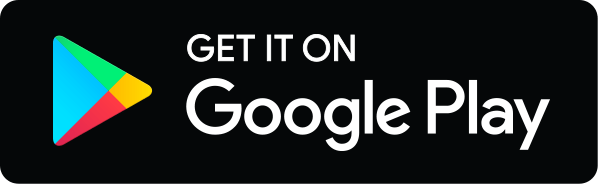