Chapter 30 Diseases of the lung parenchyma and pleura




Pulmonary Collapse
Collapse may be caused by two different mechanisms. The first of these is loss of the forces opposing the elastic recoil of the lung, which then decreases in volume to the point at which airways are closed and gas is trapped behind the closed airways. The second is obstruction of airways at normal lung volume, which may be due to many different causes. This also results in trapping of gas behind the obstructed airway. Whatever the cause of the airway closure, there is rapid absorption of the trapped gas because the total partial pressure of gases in mixed venous blood is always less than atmospheric (see Table 26.2). This generates a sub-atmospheric pressure more than sufficient to overcome any force tending to hold the lung expanded.
Pulmonary collapse during anaesthesia is described in Chapter 22.
Loss of Forces Opposing Retraction of the Lung
The lungs are normally prevented from collapse by the outward elastic recoil of the ribcage and any resting tone of the diaphragm. The pleural cavity normally contains no gas but, if a small bubble of gas is introduced, its pressure is subatmospheric (see Figure 3.5). Pulmonary collapse due to loss of forces opposing lung retraction may be considered under five headings as follows.
Voluntary reduction of lung volume. It seems unlikely that voluntary reduction of lung volume below closing capacity will cause overt collapse of lung in a subject breathing air. However, in older subjects, there is an increase in the alveolar/arterial Po2 gradient, suggesting trapping of alveolar gas (see Figure 22.11).
Excessive external pressure. Ventilatory failure is the more prominent aspect of an external environmental pressure in excess of about 6 kPa (60 cmH2O), which is not communicated to the airways (page 396). However, some degree of pulmonary collapse could also occur and this is a normal consequence of the great depths attained by diving mammals while breath holding. An approximately normal lung volume is maintained during conventional diving operations when respired gas is maintained at the surrounding water pressure, though this does not occur with surface diving or snorkelling (page 297).
Loss of integrity of the rib cage. Multiple rib fractures or the old operation of thoracoplasty may impair the elastic recoil of the ribcage to the point at which partial lung collapse results. This depends entirely on the extent of the injury to the ribcage, but multiple adjacent ribs fractured in two places will usually result in collapse. However, extensive trauma to the rib cage also causes interference with the mechanics of breathing which is generally more serious than collapse (page 396).
Space occupation of the pleural cavity. Air introduced into the pleural cavity (pneumothorax) reduces the forces opposing retraction of the lung and this is a potent cause of collapse. The same effect occurs when the pleural cavity is occupied by an effusion, empyema or haemothorax. Pleural disease is discussed on page 444 et seq.
Absorption of Trapped Gas
Gas trapped beyond the point of airway closure is absorbed by the pulmonary blood flow. The total of the partial pressures of the gases in mixed venous blood is always less than atmospheric (see Table 26.2), although pressure gradients for the individual component gases between alveolar gas and mixed venous blood may be quite different.
The effect of respired gases. If the patient has been breathing 100% oxygen prior to obstruction the alveoli will contain only oxygen, carbon dioxide and water vapour. Because the last two together normally amount to less than 13.3 kPa (100 mmHg), the alveolar Po2 will usually be in excess of 88 kPa (660 mmHg). However, the Po2 of the mixed venous blood is unlikely to exceed about 6.7 kPa (50 mmHg), so the alveolar/mixed venous Po2 gradient will be of the order of 80% of an atmosphere. Absorption collapse will thus be rapid and there will be no nitrogen in the alveolar gas to maintain inflation. This has important implications during anaesthesia, when 100% oxygen is commonly administered (page 336).
The situation is much more favourable in a patient who has been breathing air, as most of the alveolar gas is then nitrogen, which is at a tension only about 0.5 kPa (4 mmHg) below that of mixed venous blood.1 Alveolar nitrogen tension rises above that of mixed venous blood as oxygen is absorbed and eventually the nitrogen will be fully absorbed. Collapse must eventually occur but the process is much slower than in the patient who has been breathing oxygen. Figure 30.1 shows a computer simulation of the time required for collapse with various gas mixtures.2 Nitrous oxide/oxygen mixtures may be expected to be absorbed almost as rapidly as 100% oxygen. This is partly because nitrous oxide is much more soluble in blood than nitrogen, and partly because the mixed venous tension of nitrous oxide is usually much less than the alveolar tension, except after a long period of inhalation.
When the inspired gas composition is changed after obstruction and trapping occur, complex patterns of absorption may ensue. The inhalation of nitrous oxide, after airway occlusion has occurred while breathing air, results in temporary expansion of the trapped volume (Figure 30.1). This is caused by large volumes of the more soluble nitrous oxide passing from blood to alveolus in exchange for smaller volumes of the less soluble nitrogen passing in the reverse direction. This phenomenon also applies to any closed air space in the body, such as closed pneumothorax, gas emboli, bowel, and the middle ear with a blocked pharyngotympanic (Eustachian) tube. It is potentially dangerous and may contraindicate the use of nitrous oxide as an anaesthetic.
Magnitude of the pressure gradients. It needs to be stressed that the forces generated by the absorption of trapped gases are very large. The total partial pressure of gases in mixed venous blood is normally 87.3 kPa (655 mmHg). The corresponding pressure of the alveolar gases is 95.1 kPa (713 mmHg), allowing for water vapour pressure at 37°C. The difference, 7.8 kPa (58 mmHg or 78 cmH2O), is sufficient to overcome any forces opposing recoil of the lung. Absorption collapse after breathing air may therefore result in drawing the diaphragm up into the chest, reducing ribcage volume or displacing the mediastinum. If the patient has been breathing oxygen, the total partial pressure of gases in the mixed venous blood is barely one-tenth of an atmosphere (see Table 26.2) and absorption of trapped alveolar gas generates enormous forces.
Effect of reduced ventilation/perfusion ratio. Absorption collapse may still occur in the absence of total airway obstruction provided that the ventilation/perfusion () ratio is sufficiently reduced. Older subjects, as well as those with a pathological increase in scatter of
ratios, may have substantial perfusion of areas of lung with
ratios in the range 0.01–0.1. This shows as a characteristic ‘shelf’ in the plot of perfusion against
(Figure 30.2). These grossly hypoventilated areas are liable to collapse if the patient breathes oxygen (Figure 30.2B). If the
ratio is less than 0.05, ventilation even with 100% oxygen cannot supply the oxygen that is removed (assuming the normal arterial/mixed venous oxygen content difference of 0.05 ml.ml−1). As the
ratio decreases below 0.05, so the critical inspired oxygen concentration necessary for collapse also decreases (Figure 30.2C). The flat part of the curve between
ratios of 0.001 and 0.004 means that small differences in inspired oxygen concentration in the range 20–30% may be very important in determining whether collapse occurs or not. There is no difficulty in demonstrating that pulmonary collapse may be induced in healthy middle-aged subjects breathing oxygen close to residual volume.3,4
Diagnosis of Pulmonary Collapse
The diagnosis may be made on physical signs of decreased air entry and chest dullness but reliance is usually placed on chest radiography. Pulmonary opacification is seen, along with indirect signs of thoracic volume loss such as displacement of interlobular fissures, raised diaphragms, and displaced hilar or mediastinal structures.7 In the upright position, collapse is commonest in the basal segments, often concealed behind the cardiac shadow unless the exposure is appropriate. Areas of atelectasis are clearly seen with computerised tomography (see Figure 22.10).
Collapse results in a reduction in pulmonary compliance, but the value of this in diagnosis is limited by the wide scatter of normal values. A sudden reduction in compliance may give an indication of collapse, provided, of course, that control measurements were available before collapse. Collapse also reduces the functional residual capacity and arterial Po2. However, in a patient with impaired oxygenation a reduction in arterial Po2 cannot distinguish between the three very common conditions of pulmonary collapse, consolidation and oedema.
Principles of Therapy
Therapy depends on the physiological abnormality. Factors opposing the elastic recoil of the lung should be removed wherever possible. For example, pneumothorax, pleural effusion and ascites may be corrected. In other cases, particularly impaired integrity of the chest wall, it may be necessary to treat the patient with artificial ventilation. Re-expansion of collapsed lung often requires high pressures to be applied (page 336), but it is usually possible to restore normal lung volume.
When collapse is caused by regional airway obstruction, the most useful methods in both treatment and prevention are by chest physiotherapy, combined when necessary with tracheobronchial toilet, through either a tracheal tube or a bronchoscope. Fibreoptic bronchoscopy alone will often clear an obstructed airway and permit re-expansion, particularly with lobar atelectasis.8
Voluntary maximal inspirations are effective in clearing areas of absorption collapse in subjects who had been breathing oxygen near residual volume.4 This manoeuvre is the basis of the ‘incentive spirometer’, which is used to prevent postoperative lung collapse.
With artificial ventilation a logical approach is hyperinflation of the chest or an artificial ‘sigh’. Some ventilators were designed to provide an intermittent ‘sigh’ but evidence of its efficacy was never found. Current strategies to prevent pulmonary collapse during artificial ventilation are described in Chapter 32.
Pulmonary Consolidation (Pneumonia)
Effects on gas exchange. Patients with pneumonia are commonly hypoxic. Consolidated areas of lung behave in a similar fashion to collapse, forming an intrapulmonary shunt through which mixed venous blood flows. In addition, there is an increase in areas with low ratios (<0.1), but the contribution of these areas to impaired oxygenation is believed to be small because of hypoxic pulmonary vasoconstriction. Administration of oxygen to patients with pneumonia causes a further widening of the scatter of
ratios, implying a reduction in hypoxic pulmonary vasoconstriction,9 but nevertheless results in a considerable improvement in arterial Po2. In comparison with collapsed lung, consolidation is commonly associated with a worse pulmonary shunt and therefore more severe hypoxia. Many of the inflammatory mediators released as part of the response to infection act as local pulmonary vasodilators, in effect over-riding hypoxic pulmonary vasoconstriction.
Pathophysiology10
Airway inflammation was described in detail in Chapter 28. Invasion of the lower respiratory tract with viruses and bacteria leads to further inflammatory changes characterised by migration of neutrophils from the circulation into the lung tissue. Depending on the pathogen involved, the stimulus for this migration may originate from the lung epithelial cells or alveolar macrophages. Chemokines released from these cells initiate neutrophil margination, and a range of proinflammatory cytokine pathways begin. Once in the lung tissue and activated, neutrophils are highly effective killers of the invading pathogen (page 455). As part of this process an inflammatory exudate develops that leads to consolidation of the lung tissue. The exudate is a complex mixture or invading organisms, inflammatory cells (dead and alive), immunoglobulins and other immune mediators, fluid transudate from increased capillary permeability, and products resulting from destruction of lung tissue as a result of protease activity.
Margination of neutrophils. Before a neutrophil can contribute to the inflammatory response it must stick to the blood vessel wall (margination), migrate across the endothelium, interstitium and epithelium, and become activated ready to contribute to pathogen removal (see Figure 31.2). These activities are controlled by an extensive series of cytokines in a very similar fashion to airway inflammation (see Figure 28.1). Lymphocytes again play an important role, but in parenchymal inflammation macrophages have an important control function instead of the eosinophils and mast cells involved in airway inflammation.
Neutrophil margination has been extensively studied in the systemic circulation. Selectins expressed on the surface of endothelial cells transiently bind the neutrophil causing it to roll along the blood vessel wall. Eventually, different adhesion molecules on the endothelial cell (e.g. intercellular adhesion molecule-1, ICAM-1) bind to specific receptors on the neutrophil surface (e.g. β2 integrins CD11/CD18) causing a more firm adhesion to the endothelium. Once ‘caught’ by the endothelial cell, cytokines are released and neutrophil activation begins. The way in which neutrophils are marginated in the lung differs from elsewhere in the body.11,12 Adhesion to endothelial cells occurs predominantly in the pulmonary capillary, rather than in venules as in the systemic circulation. Adhesion to the capillary wall can occur by either a CD11/CD18-dependent mechanism, or by another mechanism that seems to be independent of all the adhesion molecules normally required for margination in a systemic capillary.13 Selectin-induced rolling of neutrophils may not occur. Adhesion is facilitated by a slow transit time for neutrophils across pulmonary capillaries. Human neutrophils are of similar size to red blood cells, but are much less deformable, so neutrophils take up to 120 seconds to traverse a pulmonary capillary compared with less than a second for a red blood cell.11 Inflammatory mediators may cause changes to the biomechanical properties of neutrophils, in particular, a stiffening of the cell that will further impede its movement through the pulmonary capillary.14 Once adhered to the pulmonary capillary wall neutrophils may become flattened, leaving some capillary lumen available for blood flow. In this position, emigration into the pulmonary tissue begins and the neutrophil moves through small holes in the capillary basal laminae, being guided by chemokines released from epithelial cells and possibly assisted by fibroblasts in the interstitial space (Figure 30.3).15
Interstitial Lung Disease and Pulmonary Fibrosis
Diffuse pulmonary inflammation occurs in a wide variety of conditions, which are summarised in Table 30.1. Pneumonitis may simply resolve, as in pneumonia, leaving no permanent damage, but with long-term inflammation varying degrees of pulmonary fibrosis develop.
Table 30.1 Causes of interstitial pneumonitis and pulmonary fibrosis
CAUSES | SUBGROUPS | EXAMPLES |
---|---|---|
Drug induced | Anti-cancer Antibiotics Others | Bleomycin, busulphan, cyclophosphamide, methotrexate Isoniazid, nitrofurantoin, sulphonamides Amiodarone |
Dust | Inorganic Organic | Silicosis Asbestosis Farmer’s lung |
Infections | Viral Other | Viral pneumonia HIV Mycoplasma Opportunistic infections |
Systemic disease | Connective tissue disease Others | Rheumatoid arthritis, scleroderma systemic lupus erythematosus, ankylosing spondylitis Sarcoidosis, histiocytosis, uraemia |
Miscellaneous | Acute inflammation Inhalation injury Radiation lung damage Cryptogenic fibrosing alveolitis | Acute lung injury Smoke, cadmium, sulphur dioxide |
Clinical features. vary according to the aetiology. Pneumonitis alone (i.e. without fibrosis) may be asymptomatic at first, progressing to a cough and dyspnoea, and in severe cases gives rise to systemic symptoms such as fever. When accompanied by fibrosis, dyspnoea becomes worse, and basal inspiratory crackles are present on examination. Lung function tests show a typical restrictive pattern with similar reductions in both forced vital capacity and forced expiratory volume in one second (page 96). Diffuse reticular shadows develop on chest radiography, and high-resolution CT scanning of the lungs shows either ‘ground glass’ appearances, which correlate with pneumonitis, or ‘honeycombing’, which represent more advanced fibrosis.
Causes of Pulmonary Fibrosis
These have been summarised in Table 30.1.
Drug induced fibrosis may follow lung injury induced by oxygen toxicity (page 387) precipitated by, for example, bleomycin, but the mechanism of this response is poorly understood.
Inorganic dusts.16 Occupational exposure to asbestos fibres (asbestosis) or silica (silicosis) for many years leads to pulmonary fibrosis. Inhaled dust particles between 1 and 3 μm in diameter reach the alveoli and are ingested by macrophages. Different dust types have variable persistence in the lung, some being rapidly cleared and others persisting within the pulmonary macrophage for many years. In addition, the total (lifetime) fibre burden probably correlates with the degree of resulting fibrosis.
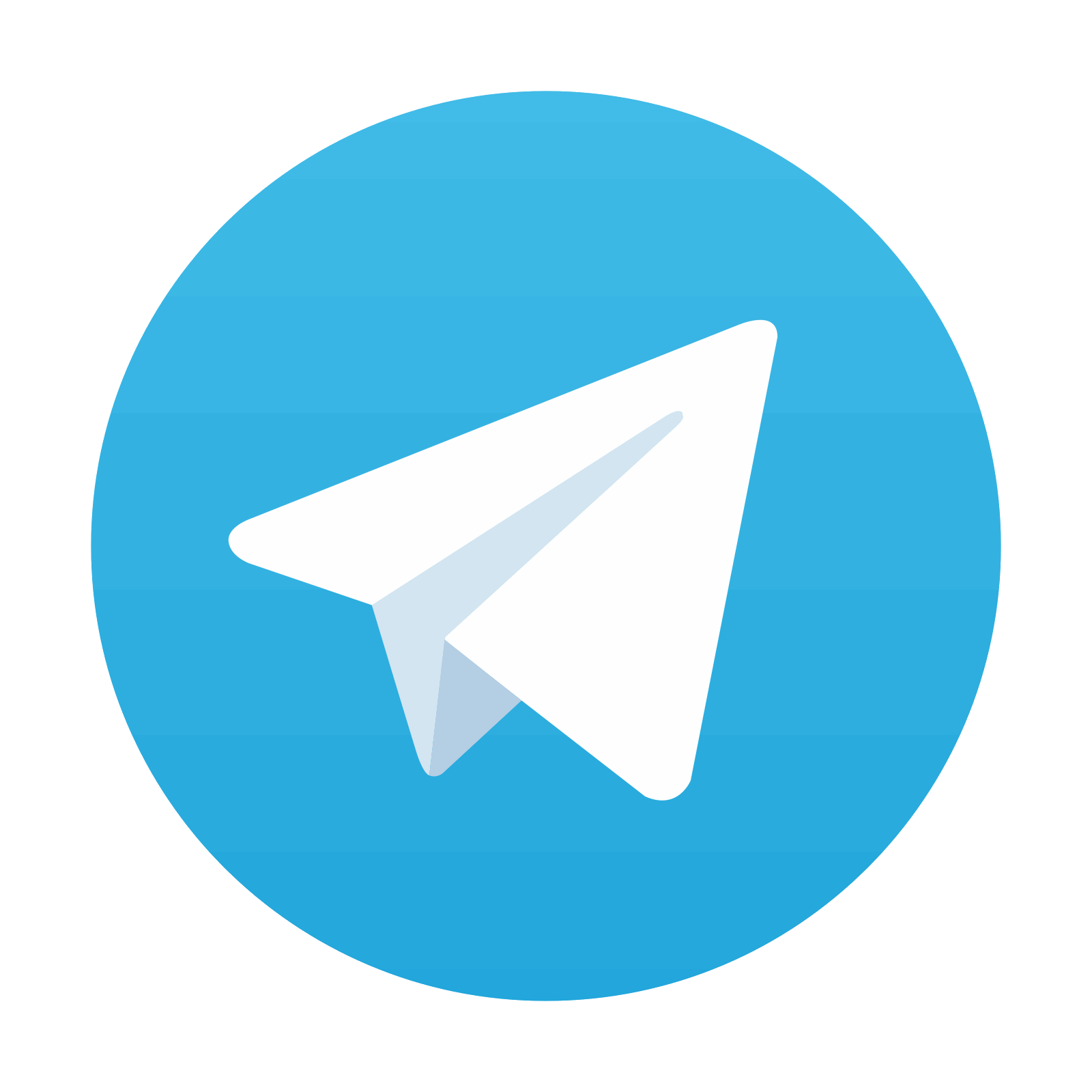
Stay updated, free articles. Join our Telegram channel

Full access? Get Clinical Tree
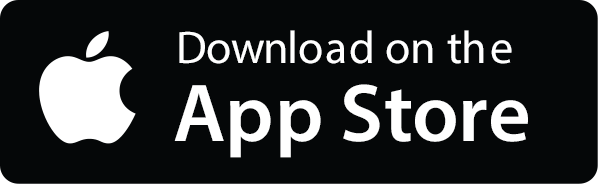
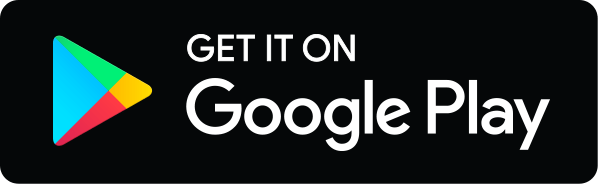