Fig. 5.1
Proposed roles and targets of SIRTs in cardioprotection
5.3 Dietary Sodium
Sodium, primarily consumed as salt (sodium chloride), is an essential nutrient because sodium and chloride are required to maintain extracellular fluid volume and plasma osmolality. The role of dietary sodium in health and disease has been a topic of great interest and debate for many years.
Experimental, epidemiological, and intervention studies have shown a positive relation of habitual dietary sodium intake and blood pressure (Elliott et al. 1996; Sacks et al. 2001). Equally well established is the association of increasing blood pressure and CVD morbidity and mortality (Gordon and Kannel 1982). However, findings from previous studies, independently from blood pressure, evaluating the direct relationship between sodium intake and cardiovascular events, have been conflicting. For example, although some have reported a positive association between sodium intake and cardiovascular outcomes (Strazzullo et al. 2009; Umesawa et al. 2008; Cook et al. 2007a), others have not (Geleijnse et al. 2007; Yang et al. 2011), and some have reported an inverse association (Cohen et al. 2006; Stolarz-Skrzypek et al. 2011). A meta-analysis (Cochrane review) of seven RCTs evaluating reduced sodium intake with follow-up of at least 6 months did not detect a significant reduction in CVD mortality or morbidity (Taylor et al. 2011). Furthermore, salt restriction increased the risk of all-cause mortality in those with heart failure. Recent observational analyses of participants in two hypertension treatment trials revealed a “J-shaped” relation of sodium intake to cardiovascular outcomes (O’Donnell et al. 2011). CVD was lowest between 4.0 and 5.9 g/day, with continuous increases in risk with sodium levels above and below that range. The authors concluded that CVD risk increases meaningfully when daily sodium intake falls below 3 g or rises to above 6 g/day (Alderman and Cohen 2012). The mechanisms responsible for an increased CVD associated with low sodium intake cannot be established by previous studies. A number of the possible mechanisms are proposed: increases in sympathetic nerve activity, plasma renin activity and aldosterone, serum cholesterol and triglyceride levels, insulin resistance, and adrenaline secretion have all been confirmed by multiple RCTs (Graudal et al. 2012; Petrie et al. 1998; Grassi et al. 2002). These biological explanations for harm at low sodium intake need further explication.
While blood pressure in the population is only modestly responsive to alterations in sodium intake, some individuals manifest large blood pressure changes in response to acute or chronic salt depletion or repletion and are termed “salt sensitive”. Weinberger et al. (1986) demonstrated that salt sensitivity existed not only in hypertensive persons but also in normotensive persons. In addition, Weinberger et al. (2001) reported that salt sensitivity in both normotensive and hypertensive persons has been associated with increased CVD morbidity and total mortality, which were independent of alterations of blood pressure. A number of recent studies, using modern molecular genetic techniques, indicate that blood pressure salt sensitivity is under the control of many genes each of which has only relatively mild effects. Candidate genes that regulate the salt sensitivity have implicated aberrations in ion channels, ion channel regulation, aldosterone signaling, vasoconstriction, and inflammation (Sanada et al. 2011; Kanbay et al. 2011; see Table 5.1). Even people with normal blood pressure as well as hypertension that are salt sensitive need to take steps to reduce their salt intake in order to prevent salt-induced blood vessel stiffness, hypertension, and CVD.
Table 5.1
Candidate genes involved in salt sensitivity
1. Genes of the renin-angiotensin system |
Angiotensin-converting enzyme |
Angiotensinogen |
Angiotensin type 1 receptor |
2. Genes related to aldosterone and other mineralocorticoids |
Aldosterone synthase |
Serum-/glucocorticoid-regulated kinase 1 |
11-β-hydroxysteroid dehydrogenase |
3. Genes of the sympathetic nervous system |
Amiloride-sensitive epithelial sodium channel |
β-3 Subunit of G-protein (GNB3) |
Renal chloride channels |
4. Genes expressing proteins that normally do not decrease renal sodium transport |
α-Adducin |
5. Genes expressing proteins that normally decrease renal sodium transport |
Adrenomedullin |
Dopamine, dopamine receptors, and GRK4 |
Endothelial nitric oxide synthase |
Eicosanoids |
Kallikrein-kinin system |
Natriuretic peptide precursor A/B |
6. Genes of the vasculature |
Endothelin |
Endothelial nitric oxide synthase (eNOS) |
Transforming growth factor-β (TGF-β) |
5.4 Saturated Fatty Acids (SFA)
Saturated fatty acids (SFA) are long-chain carboxylic acids that usually have between 12 and 24 carbon atoms and have no double bonds. The most prevailing SFA in the diet are lauric acid (12:0), myristic acid (14:0), palmitic acid (16:0), and stearic acid (18:0). SFA are present in a type of single-bond animal or vegetable fat, as that found in butter, meat, egg yolks, and coconut or palm oil.
Ecological data from the Seven Countries Study showed a strong positive association (r = 0.73) between SFA intake and coronary heart disease (CHD) incidence (Keys and Aravanis 1980). Dietary saturated fat intake has been shown to increase low-density lipoprotein (LDL) cholesterol and therefore to be associated with increased risk of CVD. SFA inhibit the activity of the LDL receptor in liver. Thus, LDL cholesterol and total cholesterol in the blood are increased by SFA. In addition, high intake of dietary SFA is positively associated with the development of insulin resistance. Dietary SFA upregulated the mRNA levels of resistin, downregulated adiponectin and glucose transporter 4 (GLUT4), and upregulated lipoprotein lipase (LPL) (Saravanan et al. 2005). These findings suggest that dietary SFA alter the expression of different genes associated with insulin sensitivity in adipose tissue.
5.5 Monounsaturated Fatty Acids (MUFA)
MUFA are distinguished from the other fatty acid classes on the basis of having only one double bond. MUFA are not nutritionally essential as they can be synthesized from other (saturated) fatty acids and from carbohydrates. Common sources of MUFA are olive oil (contains about 79 % MUFA), canola oil (contains about 54 % MUFA), avocadoes, and nuts.
The typical diet of populations living in Mediterranean countries is high in olive oil, which provides 14–40 % of calories, and consequently is high in MUFA (16–29 % of calories) (Kris-Etherton 1999). Evidence suggesting the beneficial health effects of the “Mediterranean diet” has emerged from the classic studies of Keys and Aravanis (1980), which indicate that the consumption of diets enriched in MUFA reduced incidence of CVD (Keys and Aravanis 1980; Martinez-Gonzalez et al. 2002; Knoops et al. 2004). These cardioprotective effects can be explained by several mechanisms. Foods containing high MUFA reduce total and LDL cholesterol while did not alter high-density lipoprotein (HDL) cholesterol and triglycerides (TG) (Kris-Etherton 1999). High-MUFA diet significantly improved fasting glucose and insulin sensitivity and increased plasma glucagon-like peptide-1 (GLP-1) levels but has no effect on insulin secretion (Vessby et al. 2001). Several data suggest that MUFA may decrease platelet aggregation (Sirtori et al. 1986), increase bleeding time (McDonald et al. 1989), and increase fibrinolysis (Lopez-Segura et al. 1996), thereby protecting against thrombogenesis.
Two recent studies have reported interactions between genetic variants and MUFA for obesity (Warodomwichit et al. 2009; Lai et al. 2009). Variants of the adipocyte-derived protein adiponectin have been previously linked to obesity and insulin resistance, but association studies among different ethnic groups have not been consistent. In a population of 1,083 White Americans, the A allele of the adiponectin 11,391 G > A variant was protective against obesity independently of dietary intake and also under conditions of high MUFA intake, but this relationship was reversed in subjects with low intake of MUFA (Warodomwichit et al. 2009). MUFA can affect gene expression patterns of various proteins and accordingly may regulate pathways related to CVD pathophysiology.
5.6 N-3 Polyunsaturated Fatty Acids (n-3 PUFA)
Animals and humans cannot synthesize PUFA of the n-3 series, which contain double bonds at C-3, from the methyl end of the molecule. N-3 PUFA include the plant-derived α-linolenic acid (ALA, 18:3n-3) and the fish-oil-derived eicosapentaenoic acid (EPA, 20:5n-3) and docosahexaenoic acid (DHA, 22:6n-3). PUFA represent the fundamental components of phospholipids in cellular membranes where they maintain homeostasis for correct membrane protein function and influence membrane fluidity, thus regulating cell signaling processes, cellular functions, and gene expression (Das 2006).
In the 1970s, Dyerberg et al. (1978) reported that the rate of ischemic heart disease was markedly lower in Greenland Inuits, a population consuming a high-fat diet but rich in n-3 PUFA, than in age-matched population of Denmark. Inuits have high levels of EPA and low levels of AA, and they also have a tendency to bleed. It is possible that dietary enrichment with EPA will protect against thrombosis. The following prospective observational studies and adequately powered RCTs from many countries have shown that consumption of fish oil or n-3 PUFA decreases the risk of major cardiovascular events, such as myocardial infarction, sudden cardiac death, coronary heart disease (CHD), atrial fibrillation, and death in patients with heart failure (Lee et al. 2008 b; Kromhout et al. 1985; Albert et al. 1998; Jialal et al. 1999; Yokoyama et al. 2007; Tavazzi et al. 2008).
N-3 PUFA regulate a wide variety of biological functions, depending on the location of the last double bond, which range from blood pressure and blood clotting to the correct development and functioning of the brain and nervous system. These include prevention of arrhythmias as well as lowering resting heart rate and blood pressure, decreasing platelet aggregation, and lowering triglyceride levels and might also include ameliorating myocardial efficiency and improving vascular function (Reiffel and McDonald 2006; O’Keefe et al. 2006; Harris et al. 2008).
N-3 PUFA affect a myriad of molecular pathways, including alteration of physical and chemical properties of cellular membranes, direct interaction with and modulation of membrane channels and proteins, regulation of gene expression via nuclear receptors and transcription factors, changes in eicosanoid profiles, and conversion of n-3 PUFA to bioactive metabolites. In addition, lipid mediators generated from long-chain PUFA (AA) in the n-6 series and EPA and DHA in the n-3 series have important roles in immune regulation and inflammation (Calder 2009a). Classically, eicosanoids derived from n-6 PUFA (AA) are pro-inflammatory, while eicosanoids derived from n-3 PUFA (EPA and DHA) are anti-inflammatory. Thus, high intake of n-6 PUFA, along with low intakes of n-3 PUFA, shifts the physiological state to one that is pro-inflammatory and prothrombotic.
Latest research has shown that by increasing the ratio of n-3 to n-6 fatty acids in the diet, and consequently favoring the production of EPA in the body, or by increasing the dietary intake of EPA and DHA through consumption of fatty fish or fish-oil supplements, reductions may be achieved in the incidence of many chronic diseases that involve inflammatory processes including CVD (Wall et al. 2010).
Recent years have seen the discovery of a new class of inflammation-dampening and resolution-promoting n-3 PUFA-derived lipid mediators called resolvins and protectins that are generated within resolving exudates during resolution of acute inflammation. These compounds are hydroxylated derivatives of the parent n-3 PUFA, EPA for the E-resolvins, and DHA for the D-resolvins and protectin D1 (Serhan 2007). Resolvin E1 and protectin D1 promote phagocyte removal during acute inflammation, via regulating leukocyte infiltration, increasing macrophage ingestion of apoptotic polymorphonuclear leukocyte in vivo and in vitro, and enhancing the appearance of phagocytes carrying engulfed zymosan in lymph nodes and spleen.
The role of n-3 PUFA in the prevention of CVD and their biological actions are summarized in Table 5.2.
Table 5.2
Possible mechanisms of favorable effects of n-3 PUFA on cardiovascular health
1. Retard growth of atherosclerotic plaque |
Reduce adhesion molecule expression (such as VCAM-1, E-selection, and ICAM-1) |
Reduce platelet-derived growth factor |
Anti-inflammatory |
Suppress production of pro-inflammatory cytokines (such as TNF-alpha and interleukin-1beta and IL-6) |
Produce endogenous chemical mediators (such as resolvins and protectins) |
Suppress AA cascade |
2. Improve vascular function |
Promote nitric oxide-induced endothelial relaxation |
Increase arterial compliance |
3. Mildly hypotensive |
4. Lower plasma triglyceride levels |
Reduce hepatic synthesis of triglycerides |
Increase hepatic fatty acid beta-oxidation |
5. Antiarrhythmic |
Reduce susceptibility of the heart to arrhythmia |
Inhibit fast, voltage-dependent sodium channels |
Inhibit L-type calcium channels |
Delay rectifier potassium channel |
Reduce resting heart rate |
6. Prevent heart failure |
Increase left ventricular filling capacity |
Activate peroxisome proliferator-activator receptor (PPAR)-alpha and PPAR-gamma |
7. Antithrombogenic |
Decrease platelet aggregation |
May enhance fibrinolysis |
8. Upregulate adiponectin synthesis |
9. Reduce collagen deposition |
5.7 N-6 Polyunsaturated Fatty Acids (n-6 PUFA)
Polyunsaturated fatty acids (PUFA) have two or more double bonds. N-6 PUFA is a family of unsaturated fatty acids that have in common a final carbon-carbon double bond in the n-6 position that is the sixth bond from the methyl end. N-6 PUFA is taken in mainly as linoleic acid (LA, 18:2, n-6), the shortest-chained n-6 PUFA, cannot be synthesized by the body, and is therefore an essential fatty acid. The main dietary sources of LA include plant oils such as sunflower, safflower, and corn oils. LA can be desaturated and elongated to form other n-6 PUFA such as γ-linolenic and dihomo-γ-linolenic acids. The latter is converted by the body to the metabolically important n-6 PUFA, arachidonic acid (AA, 20:4). Cyclooxygenases (COX) and lipoxygenases (LOX) can convert AA to the 2-series of prostaglandins (PGE2, PGI2, PGD2, PGF2α), the 2-series of thromboxanes (TXA2, TXB2), and the 4-series of leukotrienes (LTA4, LTB4, LTC4, LTD4, LTE4). These are very important eicosanoids that are involved in various pathological processes mediating pro-inflammatory and prothrombotic conditions such as atherosclerosis (Das 2006). In general, as above, AA-derived eicosanoids are pro-inflammatory, but they have important homeostatic functions in regulating both the promotion and resolution of inflammation in the immune response (Ricciotti and Fitzgerald 2011). Latest studies identified AA-derived eicosanoids to have several beneficial effects including vasodilation, platelet aggregation inhibition, and anti-inflammatory effects (Calder 2009b; Oltman et al. 1998). In contrast, it is known that the n-3 PUFA and their LC derivatives mostly promote anti-inflammatory activities (Tai and Ding 2010). On the other hand, recently, classes of autacoids as lipoxins derived from n-6 PUFA have been identified as specialized mediators that elicit distinct anti-inflammatory and proresolution bioactions and have cytoprotective properties (McMahon and Godson 2004).
Observational studies generally suggest an overall modest benefit of n-6 PUFA intake on CHD risk. In a meta-analysis of 25 case-control studies evaluating blood/tissue n-6 PUFA content and CHD events, LA content was inversely associated with CHD risk, whereas AA was unrelated to CHD risk (Harris et al. 2007).
In the modern Western diet, the n-6:n-3 ratio has increased to be within the range of 10:1–20:1 (Olivier et al. 2011). This increase in the ratio of n-6:n-3 appears to be involved in chronic inflammatory diseases such as CVD, nonalcoholic fatty liver disease, obesity, inflammatory bowel disease, rheumatoid arthritis, and Alzheimer’s disease. Diets for appropriate n-6:n-3 ratios should be around 1–4:1.
The role of n-6 PUFA in CVD is much more complex than the role of n-3 PUFA, and the relationship between n-3 and n-6 PUFA is complex, and they are not always in opposition. Pro- and anti-inflammatory effects of n-6 and n-3 PUFA-derived eicosanoids are summarized in Fig. 5.2 (Patterson et al. 2012).
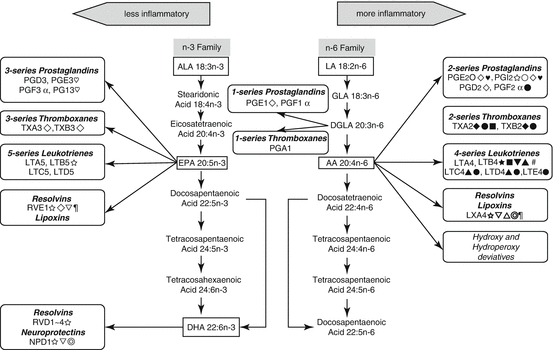
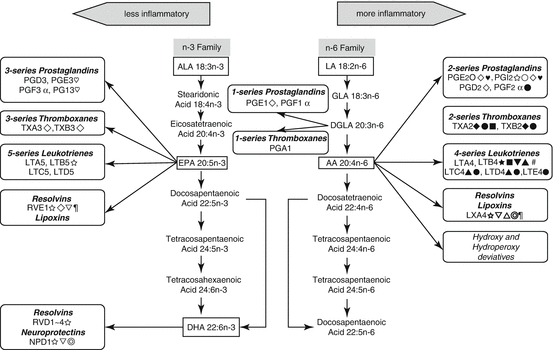
Fig. 5.2
Pro- and anti-inflammatory effects of n-6 and n-3 PUFA-derived eicosanoids. Abbreviations: LA linoleic acid, GLA γ-linolenic acid, DGLA dihomo-γ-linolenic acid, AA arachidonic acid, ALA α-linolenic acid, EPA eicosapentaenoic acid, DHA docosahexaenoic acid. Symbols:
pro-inflammatory;
anti-inflammatory; ♥ proarrythmic; ♡ antiarrhythmic;
accelerate platelet aggregation,
inhibit platelet aggregation; ● vasoconstriction; ○ vasodilation; ▲ enhance vascular permeability,
normalize vascular permeability; ▼ increase oxidative stress;
decrease oxidative stress;
release inflammatory cytokines;
decrease inflammatory cytokines; ■ enhance leucocyte chemotaxis;
antiapoptotic









5.8 Trans-Fatty Acids (TFA)
Trans-fatty acids (TFA) are unsaturated fatty acids with at least one carbon-carbon double bond in the trans, rather than the typical cis, configuration. Dietary TFA have two sources: they are formed during the natural bacterial hydrogenation of unsaturated fatty acids in small amounts in the fats of ruminants such as cows, sheep, and goats, or they come from the industrial hydrogenation of unsaturated vegetable oils such as margarines, margarine-based products, shortenings, and fats used for frying in much larger amounts.
Numerous epidemiologic studies and randomized trials have identified higher intake of TFA as an important modifiable risk factor in the development of CVD (Oomen et al. 2001; Lemaitre et al. 2006; Sun et al. 2007). This relation can be explained by several mechanisms. TFA have a wide range of physiologic effect, including both lipid and non-lipid effects. Metabolic studies showed lipid effects of TFA. TFA intakes above the population range of consumption raise LDL cholesterol, lipoprotein (a), and TG levels and reduce HDL cholesterol and LDL particle size (Mozaffarian and Clarke 2009; Mozaffarian et al. 2006). On the other hand, dietary TFA consumption leads to non-lipid risk factors, including systemic inflammation, endothelial dysfunction, and possibly insulin resistance, diabetes, and adiposity.
Recent evidence indicates that TFA intake could also affect biomarkers of inflammation and endothelial dysfunction including C-reactive protein (CRP), interleukin-6 (IL-6), soluble tumor necrosis factor receptor 2 (sTNFR-2), E-selectin, and soluble cell adhesion molecules (sICAM-1 and sVCAM-1) (Mozaffarian et al. 2004; Lopez-Garcia et al. 2005) . In another trial, consumption of TFA impaired endothelial function, as reflected by a reduction in brachial artery flow-mediated vasodilatation (de Roos et al. 2001). RCT in green monkeys indicated that consumption of TFA increased body weight, mainly due to increased deposition of intra-abdominal fat (Kavanagh et al. 2007). In adipocyte, TFA alter the expression of genes for PPAR-γ, resistin, and LPL (Saravanan et al. 2005).
Dietary Guidelines for Americans recommend limiting the amount of TFA to less than 1 % of total daily calories (less than 2 g/day) (Dietary Guidelines Advisory Committee 2005).
5.9 B Vitamins
The water-soluble vitamins of the B class include thiamine (vitamin B1), riboflavin (vitamin B2), niacin (vitamin B3), pantothenic acid (vitamin B5), pyridoxine (vitamin B6), biotin (vitamin B7), folic acid (vitamin B9), and the cobalamins (vitamin B12). B vitamins play a major role in the activities of enzymes, proteins that regulate biochemical reactions in the body, which are important in turning food into energy and other needed substances.
Drug treatment with high-dosed nicotinic acid (vitamin B3, niacin) improves the lipid profile and has been associated with reduction in morbidity and mortality from CVD. These may be due to several beneficial actions of this drug, such as antithrombotic, anti-inflammatory, and antioxidant effects. However, as a side effect, treatment with high-dose nicotinic acid also causes impaired glucose tolerance and elevations in uric acid and homocysteine levels in the blood, which is associated with an increased risk of CVD (Florentin et al. 2011).
The most recognized cardioprotective effects of B vitamins have been estimated with the lowering blood levels of the sulfur-containing amino acid, homocysteine. Several epidemiological studies demonstrated association between dietary intake or concentrations of B vitamins (folic acid, vitamin B6, and vitamin B12), homocysteine, and CVD (Rimm et al. 1998; Ishihara et al. 2008). Elevated homocysteine concentrations have been related to increased oxidant stress, impaired endothelial function, and increased thrombogenicity (Wierzbicki 2007). Each 5 μmol/l of homocysteine has been associated with an approximately 20 % increase in the risk of CHD events, independent of traditional CHD risk factors (Humphrey et al. 2008). Unfortunately, long-term RCTs have not documented benefits of folic acid with or without vitamin B6 and vitamin B12 on CVD outcomes (Bazzano et al. 2006). In some trials, supplemental folic acid was associated with increased risk of CVD (Miller et al. 2010). B vitamins have been shown to play a role in lowering blood levels of homocysteine, but it is not clear whether the lowering levels of homocysteine will reduce risk of heart disease. Further investigation is needed about this discrepancy in effects of B vitamins from epidemiologic studies and clinical trials.
5.10 Vitamin C
Vitamin C (ascorbic acid), a water-soluble vitamin, is widely distributed in fresh fruits and vegetables. Because humans different to rats do not have the ability to biosynthesize their own vitamin C, it must be obtained through their diet. Its deficiency has long been known to cause scurvy that is a lethal condition unless appropriately treated. Vitamin C is available in reduced form (l-ascorbic acid, biologically active form) and oxidized form (l-dehydroascorbic acid).
Results of previous studies on whether vitamin C is helpful for preventing CVD are mixed. But some evidence suggests that it may help protect against atherosclerosis by acting as an antioxidant (Losonczy et al. 1996; Knekt et al. 2004). Unfortunately, results from most clinical intervention trials have failed to show a beneficial effect of vitamin C supplementation on primary or secondary prevention of CVD (Cook et al. 2007b; Sesso et al. 2008).
Several mechanisms could explain a connection between vitamin C and heart disease. Vitamin C is known to be a powerful antioxidant in the body because, by donating its electrons, it prevents other compounds from being oxidized. As an antioxidant, vitamin C may protect lipids, particularly LDL, from peroxidation, and it keeps LDL ability to bind to LDL receptors (Sakuma et al. 2001). Oxidized LDL can play a key role in the pathogenesis of atherosclerosis, the underlying disorder leading to CVD. Vitamin C in vitro also can recycle vitamin E, which can donate electrons to prevent LDL oxidation in vitro. As the lipid-phase vitamin E is oxidized, it can be regenerated by aqueous vitamin C. Other possibilities are that vitamin C could improve vasodilatation and vascular reactivity, perhaps by decreasing the interactions of nitric oxide (NO) with oxidants (Vita et al. 1998). Pharmacological doses of vitamin C produce vasodilatation in the coronary arteries (Levine et al. 1996). In healthy subjects, vitamin C administration restored endothelium-dependent vasodilatation that was impaired by acute hyperglycemia (Beckman et al. 2001). Thus, vitamin C may have favorable effects on vascular dilatation, possibly through its antioxidant effects on NO (Jackson et al. 1998). Recent results have shown that endothelial cells recycle and accumulate (May and Qu 2005) and release (Davis et al. 2006) vitamin C by glutathione-dependent mechanisms. These regulation of vitamin C uptake, accumulation, and release by the endothelial cells may maintain the optimal antioxidant capacity of the plasma and the neighboring extracellular space to increase local NO bioavailability and prevent formation of the cytotoxic peroxynitrite (ONOO−).
5.11 Vitamin D
Vitamin D is a fat-soluble vitamin that plays a hormonelike action. Two major forms of vitamin D are vitamin D2 (ergocalciferol) and D3 (cholecalciferol). 1,25-dihydroxyvitamin D [1,25(OH)2D] is the most active vitamin D metabolite, and its receptor (VDR) generates biological responses in the cardiovascular system. Expression of the VDR and vitamin D-metabolizing enzymes in the cardiovascular system including vascular smooth muscle cells, endothelial cells, and cardiomyocytes suggests an important role for vitamin D in the cardiovascular system.
Low-level vitamin D status is a common problem in the general population. The Third National Health and Nutrition Examination Survey (NHANES III) reported the prevalence of vitamin D deficiency in US adults to be 25–57 % (Looker et al. 2002). Risk factors for vitamin D deficiency include advanced age, dark skin color, homebound status, increased distance from the equator, winter season, clothing, the use of sunscreen, air pollution, smoking, obesity, malabsorption, renal disease, liver disease, and different medications (Lee et al. 2008a). Among CVD patients, vitamin D deficiency is a common problem. Vitamin D suppresses the progression of heart failure, hypertension, and myocardial hypertrophy and decreases prevalence of cardiovascular risk factors and related morbidity and mortality.
Several prospective studies indicated that individuals with low level of serum 25-hydroxyvitamin D were at increased risk for CVD (Wang et al. 2008; Giovannucci et al. 2008). Recent meta-analysis of epidemiological studies summarized that high vitamin D concentrations were associated with lower prevalence of CVD (Parker et al. 2010). Although the observational studies were suggestive of increased CVD risks associated with low levels of serum 25-hydroxyvitamin D, recent systematic reviews show that the evidence was inconsistent and insufficient to prove a cause and effect relationship between vitamin D supplementation and prevention of cardiovascular events (Shapses and Manson 2011; Wang et al. 2010).
Vitamin D has a wide variety of the cardioprotective mechanisms including the inhibition of vascular smooth muscle proliferation, the suppression of vascular calcification, the downregulation of pro-inflammatory cytokines, the upregulation of anti-inflammatory cytokines, the suppressive effect on platelet aggregability, the improvement of insulin secretion and sensitivity, and the decreases of renin, angiotensin levels, atrial natriuretic peptide, brain natriuretic peptide, plasminogen activator inhibitor-1, and vascular endothelial growth factor (Zittermann et al. 2005; Darabian et al. 2012; Rammos et al. 2008; Deluca et al. 2001; Gysemans et al. 2005).
5.12 Vitamin E
Vitamin E, a fat-soluble vitamin, is an essential micronutrient. Of the eight naturally occurring forms of vitamin E, only alpha-tocopherol, and to a minor extent gamma-tocopherol, is carried in human blood and is considered to be the active form. Numerous foods provide vitamin E. Nuts, seeds, and vegetable oils are among the best sources of alpha-tocopherol, and significant amounts are available in green leafy vegetables and fortified cereals.
Several prospective cohort studies have associated lower rates of CHD with higher vitamin E consumption or supplementation (Rimm et al. 1993; Stampfer et al. 1993; Knekt et al. 1994). On the other hand, RCTs have failed to show reductions in CVD events with supplemental vitamin E, and two meta-analyses suggest that high-dose vitamin E supplements may increase total mortality (Bjelakovic et al. 2007; Miller et al. 2005). More evidence is needed to determine if there are benefits of vitamin E supplementation.
The cardioprotective effects of vitamin E are attributed to its antioxidant properties that stop the production of reactive oxygen species (ROS) formed when fat undergoes oxidation. Of the antioxidant vitamins, vitamin E may be the most potent inhibitor of lipid oxidation because it is fat-soluble and constitutes part of the LDL molecule (Pryor 2000). It is thought to exert its primary protective effects via the protection of LDL from oxidation. This effect has been demonstrated in laboratory animals in vivo (Keaney and Frei 1994), isolated tissues ex vivo, and in human populations (Pryor 2000).
Vitamin E may affect the pathogenesis of atherosclerotic vascular disease beyond its direct effects on lipids. Vitamin E could affect CVD morbidity and mortality by reducing platelet adhesion, inhibiting vitamin-K-dependent clotting factors, or stimulating nitric oxide formation by the endothelial cell (Pryor 2000).
5.13 Carotenoids
Carotenoids are a class of more than 600 compounds that are responsible for the red, yellow, and orange fat-soluble pigments found principally in plants. Fruits and vegetables are the main source of carotenoids in the human diet. The most common carotenoids in the human diet are α-carotene, β-carotene, β-cryptoxanthin, lycopene, lutein, zeaxanthin, and astaxanthin.
A number of retrospective and prospective longitudinal studies have identified an inverse association between carotenoid intake or serum concentrations of total or individual carotenoids and CVD risk (Osganian et al. 2003; Sesso et al. 2005; Evans et al. 1998; Buijsse et al. 2008), although the results of these studies are somewhat conflicting.
< div class='tao-gold-member'>
Only gold members can continue reading. Log In or Register a > to continue
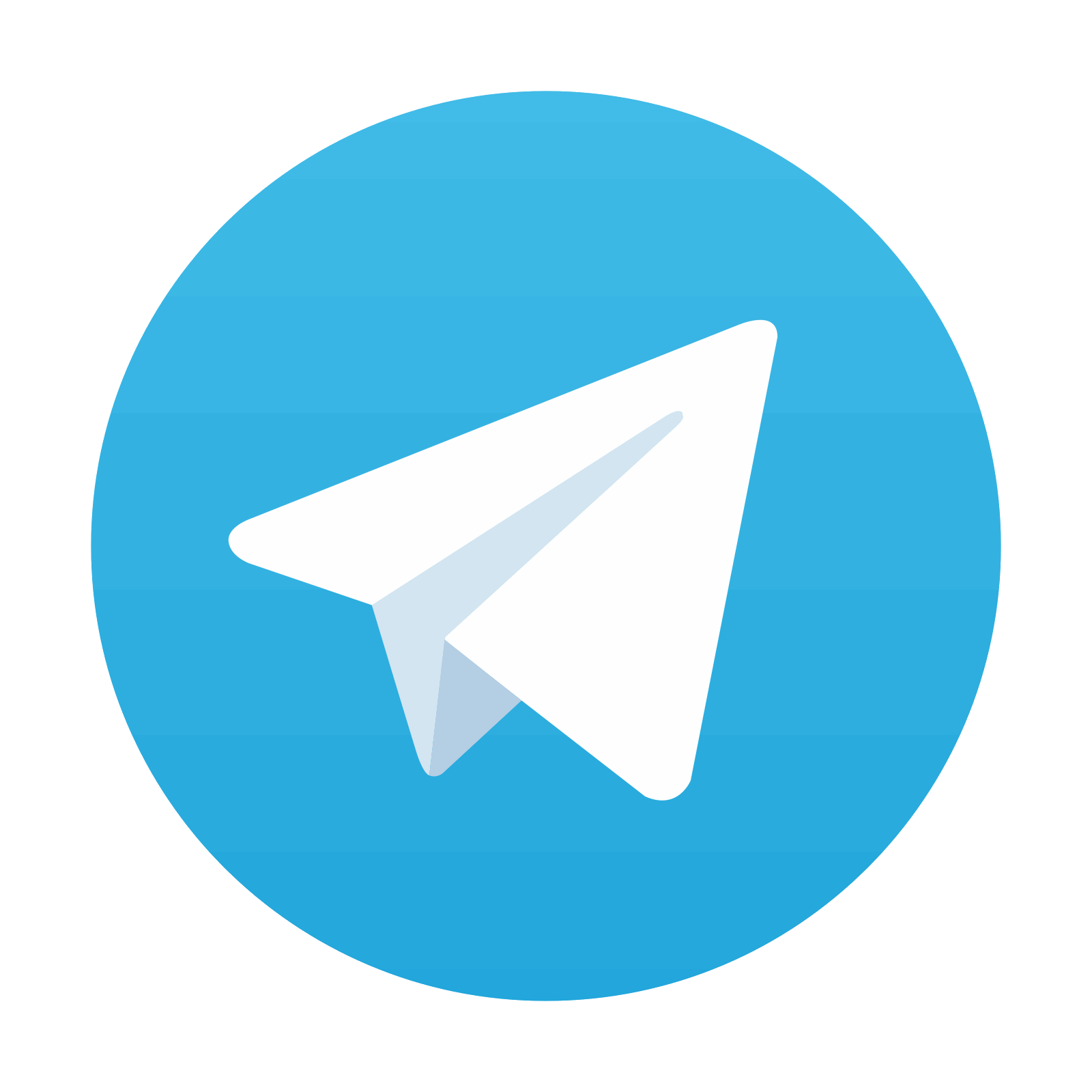
Stay updated, free articles. Join our Telegram channel

Full access? Get Clinical Tree
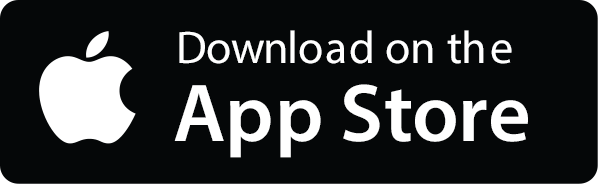
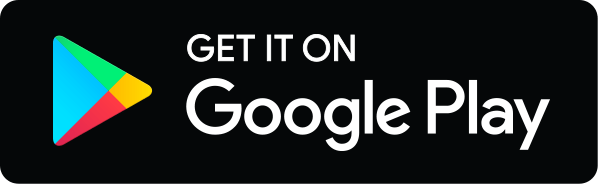