Background
The response of diastolic Doppler indices to exercise is not well defined for young subjects. The aims of this study were to evaluate this in nonathletic and endurance-trained probands and to correlate echocardiographic data with maximal oxygen consumption.
Methods
In this prospective study, Doppler echocardiography was performed at rest and after exercise in 40 nonathletes (NAs) and 20 endurance-trained athletes (ETs) aged < 40 years, matched for age and gender. Diastolic function was assessed by mitral inflow and early diastolic velocities of the septal (e′ septal) and lateral (e′ lateral) mitral annulus. Maximal oxygen consumption quantification was performed simultaneously.
Results
All cardiac chambers were larger in ETs than NAs. ETs had higher e′ lateral at rest (18.1 ± 2.7 vs 16.3 ± 3.3 cm/sec, P = .02) and higher mitral E (141 ± 15 vs 132 ± 15 cm/sec, P = .02) and e′ lateral (23.5 ± 2.5 vs 21.4 ± 3.0 cm/sec, P = .01) with exercise than NAs. There was a slight increase in E/e′ septal (overall, from 6.8 ± 1.3 to 7.2 ± 1.2; P = .02) and E/e′ lateral (overall, from 5.0 ± 0.8 to 6.2 ± 0.9; P < .0001) with exercise. Changes in diastolic parameters with exercise were similar in ETs and NAs. Percentage of predicted maximal oxygen consumption was correlated with exertional E ( r = 0.28, P = .03) and e′ lateral ( r = 0.32, P = .01), but the strongest predictor was indexed left ventricular end-diastolic volume ( r = 0.66, P < .0001).
Conclusions
During exercise, E/e′ increases but remains within normal ranges in healthy young subjects, and the response to exercise does not differ between ETs and NAs. These data help define the normal diastolic stress echocardiographic response in the young. Exercise capacity shows a correlation with enhanced exertional early diastolic velocities but is more closely related to cardiac structural adaption to endurance training.
Diastolic stress echocardiography is used for the noninvasive estimation of left ventricular (LV) filling pressure with exercise. The test is thought to be particularly helpful in patients with exertional dyspnea, in whom Doppler echocardiographic evaluation of diastolic function at rest is not diagnostic because it reveals only the presence of mild dysfunction or equivocal data.
In healthy subjects, filling rates can be substantially increased during exercise without increases in LV filling pressures, because of increased rates of myocardial relaxation and systolic emptying. In these subjects with preserved myocardial relaxation, parameters of early diastolic function, such as early diastolic transmitral velocity (E) and early diastolic mitral annular tissue velocity (e′), were shown to increase in parallel during exercise, resulting in an unchanged E/e′ ratio. An increase in E/e′ with exercise, on the other hand, is thought to reflect a pathologic response indicating an exertional rise in LV filling pressures. However, the data providing the base for these conclusions are derived from studies in populations with an average age of about 60 years.
With aging, the rates of myocardial relaxation and elastic recoil gradually decrease. Therefore, it is well established that the patients’ age must be considered when interpreting diastolic function by Doppler echocardiography under resting condition. To date, the influence of age on diastolic stress echocardiography has not been defined. In particular, it is not known whether young subjects exhibit a different response to exercise compared with their older counterparts.
The endurance-trained heart, which is known to become structurally adapted to intensive exercise, may be of particular interest in this context. Under resting conditions, diastolic function has been shown to be normal or even supernormal in endurance athletes compared with normal controls. Potentially, diastolic performance may be more enhanced during exercise in response to intensive endurance training, facilitating LV filling of athletes’ hearts at high heart rates. However, available data on diastolic stress echocardiography in endurance athletes are sparse, and a systematic comparison between young endurance-trained athletes (ETs) and nonathletes (NAs) is lacking.
If diastolic function during exercise were enhanced in endurance trained subjects, one would expect that diastolic stress echocardiographic parameters would be associated with exercise capacity. Previously published data in this field, however, are somewhat conflicting and thus remain inconclusive.
In a previous study by our group investigating young and healthy mountaineers, diastolic stress echocardiography performed under normoxic conditions unexpectedly showed a slight but significant increase in the E/e′ ratio in response to exercise. This finding differed from the normal response seen in older subjects. The reason for this observation was not clear. It could have potentially been attributed to the extraordinary training state or the young ages of the mountaineers.
The present prospective study was conducted to further investigate diastolic stress echocardiography in healthy young subjects. Therefore, diastolic function was assessed by Doppler and tissue Doppler echocardiography at rest and with exercise in healthy ETs and NAs. The two groups were chosen because we were particularly interested in the adaption of exertional diastolic function to endurance training. We hypothesized that diastolic stress echocardiographic parameters would be enhanced in young athletes as opposed to their more sedentary, age-matched counterparts. In addition to diastolic stress echocardiography, we performed simultaneous spiroergometry to correlate parameters of cardiac function and structure to exercise capacity as assessed by maximal oxygen consumption (V o 2max ).
Methods
Subjects
Sixty subjects aged 18 to 40 years were included in this prospectively designed and conducted study. Of these, 20 were amateur ETs who performed ≥5 hours of training per week. The other 40 subjects were healthy NAs leading normally active lifestyles. The study groups were matched for age and gender. Study participants had no cardiovascular risk factors and were free of cardiac symptoms. The study was approved by the local ethics committee. All patients provided written informed consent.
Two-Dimensional and Doppler Stress Echocardiography
Subjects underwent exercise testing on a cycle ergometer (Ergoline Ergometrics-900; Schiller AG, Baar, Switzerland) in the semisupine (45%) body position until exhaustion. Echocardiographic images were obtained at rest and immediately after exercise with the subject tilted to a left lateral position for image acquisition. A Vivid 9 ultrasound system with a 2.5-MHz transducer (GE Medical Systems, Milwaukee, WI) was used. Echocardiographic data were stored digitally, and data analysis was performed offline (EchoPAC version 7; GE Medical Systems).
LV ejection fraction and LV end-diastolic volumes were assessed by planimetry using Simpson’s method. LV myocardial mass was calculated as recommended. Right ventricular end-diastolic area was calculated by outlining the endocardial border of the right ventricle in end-diastole in the apical four-chamber view. Left atrial volume was calculated using the biplane area-length method. Right atrial area was calculated using the single-plane method of disks. Structural cardiac parameters were indexed to body surface area.
In the apical four-chamber view, transmitral inflow velocities were recorded in the pulsed-wave Doppler mode. Myocardial velocities were measured in the same view by Doppler tissue imaging using spectral Doppler mode, placing a 5-mm sample volume at both the septal and lateral mitral annulus. Gain was adjusted to optimize the signal-to-noise ratio of the tissue Doppler tracing. From the tissue Doppler recordings, early diastolic velocities (e′ septal and e′ lateral) and systolic peak velocities (s′ septal and s′ lateral) were measured.
For strain analysis, the two-dimensional speckle-tracking method was used as previously described. LV global longitudinal strain was calculated from loops acquired from the apical two-, three-, and four-chamber views.
Cycle Ergometer Cardiopulmonary Exercise Test
V o 2max was assessed as a measure of exercise capacity that is closely related to cardiac output. The cardiopulmonary exercise test was performed on the cycle ergometer at the same time as stress echocardiography. Subjects cycled in the straight semisupine body position until exhaustion. Spiroergometric data were collected before tilting the subject to the left lateral position for echocardiographic image acquisition. Gas exchange was assessed breath by breath using a spirometer (Cardiovit AT-104; Schiller AG). The system was calibrated against a standardized gas solution before each test. Oxygen was analyzed by a rapidly responding zirconia fuel cell and carbon dioxide by an infrared analyzer. Flow measurements were performed using a disposable pneumotachograph.
For the correlation between echocardiographic data and exercise capacity, V o 2max is expressed as a percentage of the predicted value. This approach was chosen because male subjects achieve larger V o 2max values than matched female subjects. When performing a correlation analysis in a mixed population, this gender-related difference must be considered. The percentage of the predicted V o 2max value accounts for the influence of gender on exercise capacity.
Statistical Analysis
Continuous data are presented as mean ± SD. Continuous data were compared by using Mann-Whitney tests and categorical variables by using χ 2 tests. Comparisons between rest and exercise data were performed by using repeated-measures analysis of variance. Linear regression was used for the correlation between percentage of predicted V o 2max and echocardiographic parameters. P values < .05 were considered statistically significant.
Reproducibility of diastolic stress echocardiographic measurements was assessed in 20 randomly selected subjects. Interobserver and intraobserver variability was expressed as mean percentage error, calculated as the difference between two repeated measurements, divided by the average of the two measurements and expressed as a percentage.
Statistical analyses were performed using JMP version 10 (SAS Institute, Cary, NC).
Results
Baseline Characteristics
The demographics and general physical characteristics are presented in Table 1 . ETs performed 9.2 ± 3.8 hours of endurance training per week, whereas NAs, on average, trained for 0.9 ± 1 hours weekly ( P < .0001). In ETs, training units comprised running, cycling, and swimming in eight subjects; running and cycling in three subjects; running in six subjects; and cycling in three subjects, respectively.
Variable | ETs | NAs | P |
---|---|---|---|
Age (y) | 29.3 ± 5.1 | 29.8 ± 6.0 | .61 |
Women | 7 (35%) | 14 (35%) | 1.00 |
Weight (kg) | 69 ± 10 | 73 ± 12 | .28 |
Height (cm) | 175 ± 9 | 176 ± 8 | .89 |
BSA (m 2 ) | 1.8 ± 0.2 | 1.9 ± 0.2 | .35 |
BMI (kg/m 2 ) | 22.4 ± 1.8 | 23.6 ± 3.2 | .19 |
Heart rate (beats/min) | 62 ± 18 | 76 ± 13 | .002 |
Systolic blood pressure (mm Hg) | 126 ± 12 | 134 ± 15 | .05 |
Diastolic blood pressure (mm Hg) | 79 ± 10 | 79 ± 9 | .56 |
Stress Echocardiographic Data
Cardiac structural parameters assessed under resting condition in ETs and NAs are summarized in Table 2 . LV size was larger and muscle mass higher in ETs than in NAs. Likewise, the other cardiac chambers were larger in ETs compared with NAs ( Table 2 ). Diastolic stress echocardiographic data are shown in Table 3 and illustrated in Figure 1 . The differences between rest and exercise values were similar in ETs and NAs for all diastolic stress echocardiographic parameters (ΔE, 56 ± 16 vs 51 ± 14 cm/sec, P = .22; Δe′ septal, 7.0 ± 2.6 vs 6.7 ± 3.2 cm/sec, P = .65; Δe′ lateral, 5.4 ± 4.1 vs 5.0 ± 2.7 cm/sec, P = .86; Δe′ average, 6.1 ± 3.0 vs 5.8 ± 2.8 cm/sec, P = .76; ΔE/e′ septal, 0.5 ± 1.3 vs 0.3 ± 1.3, P = .76; ΔE/e′ lateral, 1.4 ± 1.2 vs 1.2 ± 0.9, P = .46; ΔE/e′ average, 1.0 ± 1.2 vs 0.9 ± 0.9, P = .54). Resting and exertional E/e′ values were very similar between groups ( Figure 1 ). There was a slight increase in E/e′ septal from rest to exercise, which was statistically significant for the overall population (from 6.8 ± 1.3 to 7.2 ± 1.2, P = .02). Similar to E/e′ septal values, E/e′ lateral values increased but remained low with exercise (E/e′ lateral for the overall population, 5.0 ± 0.8 at rest vs 6.2 ± 0.9 after peak exercise; P < .0001 for the difference between rest and exercise). Representative mitral inflow and mitral annular velocity patterns of a subject with increases in E/e′ septal and E/e′ lateral with exercise are shown in Figure 2 .
Variable | ETs | NAs | P |
---|---|---|---|
LVEDV index (mL/m 2 ) | 76 ± 14 | 58 ± 12 | <.0001 |
LVESV index (mL/m 2 ) | 29 ± 7 | 22 ± 6 | <.001 |
LVMM index (g/m 2 ) | 90 ± 21 | 71 ± 13 | .0004 |
LV septal thickness (mm) | 9.8 ± 1.4 | 8.8 ± 1.5 | .02 |
LV posterior wall thickness (mm) | 8.8 ± 1.3 | 7.8 ± 0.9 | .004 |
LA volume index (mL/m 2 ) | 25 ± 8 | 16 ± 4 | <.0001 |
RVEDA index (cm 2 /m 2 ) | 10 ± 1.6 | 8.9 ± 1.6 | .02 |
RA area index (cm 2 /m 2 ) | 8.9 ± 1.5 | 7.2 ± 1.3 | .0001 |
Variable | Rest | Exercise | ||||
---|---|---|---|---|---|---|
ETs | NAs | P | ETs | NAs | P | |
E velocity (cm/sec) | 85 ± 14 | 81 ± 14 | .32 | 141 ± 15 | 132 ± 15 | .02 |
E′ septal (cm/sec) | 12.5 ± 2.0 | 12.4 ± 2.4 | .78 | 19.5 ± 2.8 | 19.1 ± 2.8 | .66 |
E′ lateral (cm/sec) | 18.1 ± 2.7 | 16.3 ± 3.3 | .02 | 23.5 ± 2.5 | 21.4 ± 3.0 | <.01 |
E′ average (cm/sec) | 15.3 ± 2.2 | 14.3 ± 2.5 | .14 | 21.5 ± 2.1 | 20.3 ± 2.7 | .06 |
E/e′ septal | 6.9 ± 1.1 | 6.7 ± 1.4 | .49 | 7.4 ± 1.3 | 7.1 ± 1.1 | .32 |
E/e′ lateral | 4.8 ± 0.8 | 5.1 ± 0.8 | .17 | 6.1 ± 1.0 | 6.2 ± 0.9 | .77 |
E/e′ average | 5.6 ± 0.9 | 5.7 ± 0.9 | .67 | 6.6 ± 1.0 | 6.6 ± 0.9 | .90 |
LVEF (%) | 62 ± 5 | 62 ± 4 | .88 | 78 ± 4 | 79 ± 3 | .39 |
LV GLS (%) | 21.8 ± 2.2 | 22.1 ± 2.4 | .84 | 26.2 ± 2.0 | 27.0 ± 2.6 | .29 |
S′ septal (cm/sec) | 8.9 ± 1.6 | 9.1 ± 1.6 | .90 | 16.9 ± 2.4 | 16.3 ± 2.4 | .43 |
S′ lateral (cm/sec) | 11.5 ± 1.8 | 11.4 ± 2.7 | .34 | 18.8 ± 3.6 | 18.2 ± 3.2 | .78 |


At rest, none of the subjects had fusion of the E and A waves. With exercise, fusion occurred in 27 subjects (45%). The proportion of subjects with fusion of the E and A waves after exercise was similar in ETs and NAs (nine [45%] vs. 18 [45%], P = 1.00). When subjects with fusion were excluded from the analysis, the behavior of the E/e′ ratio remained similar as in the overall population (E/e′ septal, 6.9 ± 1.3 to 7.5 ± 1.1, P = .007; E/e′ lateral, 5.1 ± 0.9 to 6.3 ± 0.9, P < .0001).
LV systolic parameters assessed during stress echocardiography are presented in Table 3 .
Cardiopulmonary Exercise Test
Data derived from cardiopulmonary exercise testing are presented in Table 4 . Heart rate and blood pressure values measured at peak exercise were similar in ETs and NAs. ETs achieved better exercise capacity and higher V o 2max than NAs ( Table 4 ).
Variable | ETs | NAs | P |
---|---|---|---|
Peak heart rate (beats/min) | 166 ± 9 | 165 ± 16 | .81 |
Peak systolic BP (mm Hg) | 194 ± 24 | 192 ± 27 | .73 |
Peak diastolic BP (mm Hg) | 85 ± 21 | 82 ± 17 | .70 |
Exercise duration (min) | 22.0 ± 2.4 | 20.2 ± 4.2 | .008 |
Achieved workload (W) | 249 ± 56 | 184 ± 43 | <.001 |
Percentage of predicted maximal workload | 136 ± 17 | 98 ± 17 | <.0001 |
V o 2max (mL · min −1 · kg −1 ) | 46 ± 9 | 33 ± 7 | <.0001 |
Percentage of predicted V o 2max | 121 ± 18 | 91 ± 14 | <.0001 |
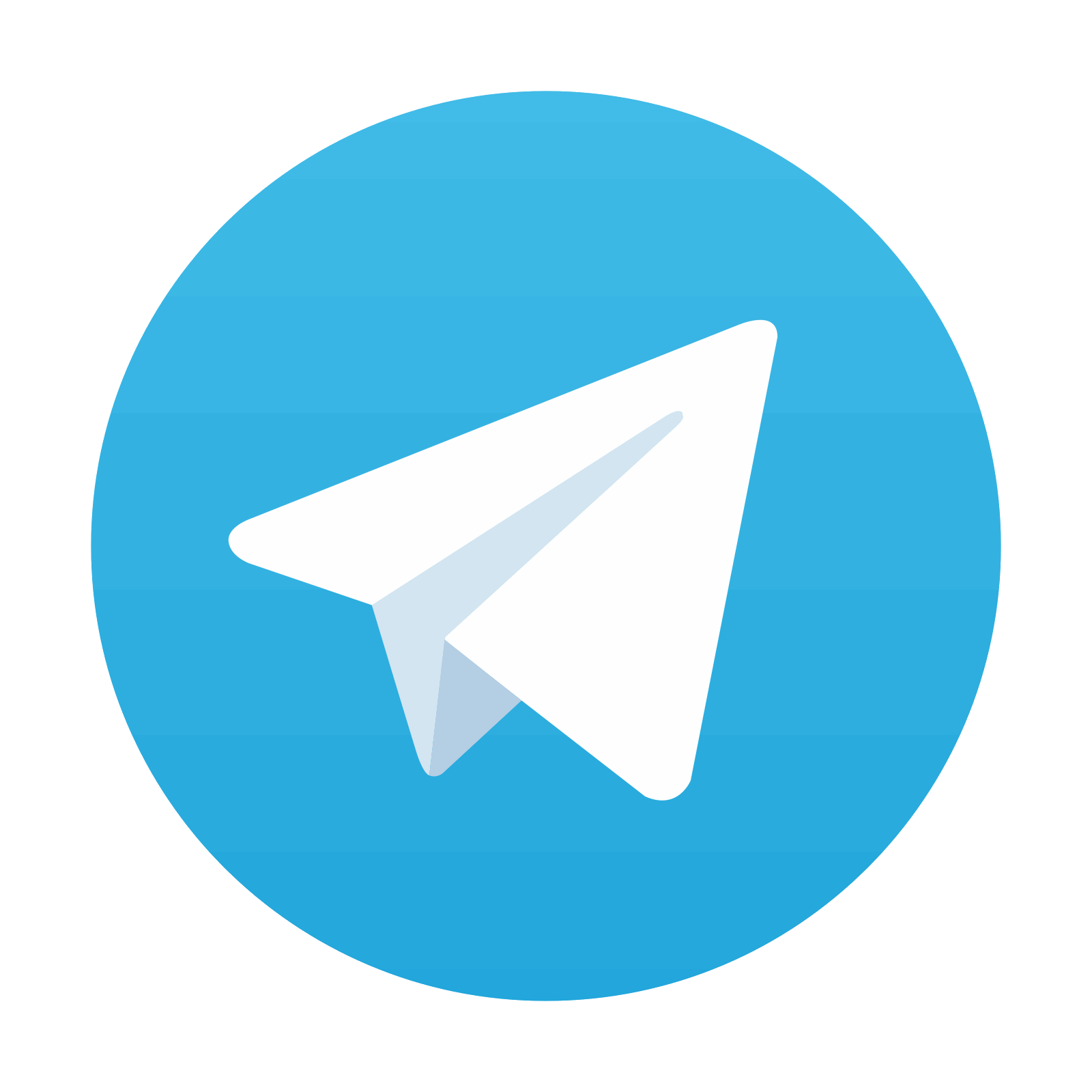
Stay updated, free articles. Join our Telegram channel

Full access? Get Clinical Tree
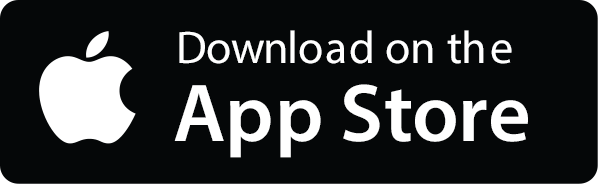
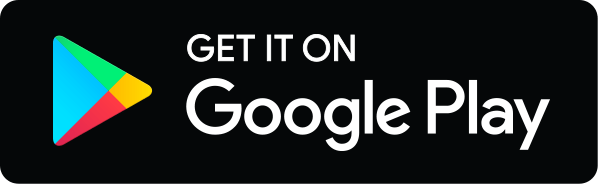
