A 71-year-old woman with a history of longstanding hypertension, diabetes mellitus, and obesity presents for evaluation of dyspnea on exertion. Her symptoms started about 3 years ago and gradually worsened over the past 2 years. She reports shortness of breath with mild physical activities, such as climbing less than 1 flight of stairs, and even with walking only few steps carrying her laundry basket. She sleeps on 2 pillows and reports occasional bilateral ankle edema. She denies resting or exertional chest pain. A 12-lead electrocardiogram demonstrated normal sinus rhythm, mild left ventricular hypertrophy, and nonspecific ST changes. A transthoracic echocardiogram showed mild left ventricular concentric hypotrophy with preserved ejection fraction (65%). Mitral inflow Doppler parameters were normal, with septal annual E′ velocity of 8 cm/s. Her right ventricular function was normal, with a resting right ventricular systolic pressure of 37 mm Hg. Pulmonary function test demonstrated mild restrictive pattern.
She was referred by her internist for an exercise stress echocardiogram. She exercised 3 minutes on the Bruce protocol and stopped due to severe dyspnea. She demonstrated a hypertensive response to exercise and did not reach her target heart rate. At rest, her blood pressure was 141/85 mm Hg and her heart rate was 70 bpm. At peak exercise, her blood pressure was 188/110 mm Hg and her heart rate was 96 bpm. Peak stress echocardiographic images demonstrated normal wall motion augmentation in all segments. Due to persistent symptoms, coronary angiography was performed and showed mild nonobstructive coronary artery disease and an left ventricular end-diastolic pressure of 14 mm Hg.
Her medications included hydrochlorothiazide 25 mg/d orally and amlodipine besylate (Norvasc) 10 mg/d orally. Her cardiovascular exam was unremarkable with the exception of mild ankle edema bilaterally. Laboratories values demonstrated that complete blood count, serum electrolytes, and kidney function were within normal limits, and notably, her brain natriuretic peptide (BNP) was 87 pg/mL (normal range, <100 pg/mL).
She underwent a right heart catheterization that showed the following: right atrium: 8 mm Hg; pulmonary artery (PA): 34/13 mm Hg; and pulmonary capillary wedge pressure (PCWP): 15 mm Hg. She was subsequently referred to a heart failure program at a tertiary care center where an exercise hemodynamic study was performed and demonstrated significant exercise-induced elevation in pulmonary and wedge pressures (peak exercise PA: 47/27 mm Hg; PCWP: 28 mm Hg). She was diagnosed with heart failure with preserved ejection fraction (HFpEF) and enrolled in an investigational trial evaluating the safety and clinical benefits of an interatrial shunt device (IASD) aimed at relieving excessive left atrial pressure, which is a common physiopathologic pathway in HFpEF symptomatology. Pre- and post-IASD® hemodynamics are summarized in Figures 6-1 and 6-2.
Figure 6-1
Hemodynamic tracing demonstrates normal resting Right Atrial (RA) pressure, Pulmonary Artery pressures (PA) and Pulmonary Capillary Wedge Pressure (PCWP). With recombinant bike exercise, there was marked increase in PCWP from 15 mm Hg to 28 mm Hg and parallel increase in PA pressure from 34/13 mm Hg to 47/27 mm Hg.

Figure 6-2
The patient is now one-month post placement if IASD device. At rest, RA, PA, PCWP were all within normal limits. At peak exercise on a recombinant bike, there was less increase in PCWP (9 → 19 mm Hg) with no significant change in baseline or exercise PA pressures compared to matching pressures in the pre-IASD study.

HFpEF is an increasingly common clinical entity resulting in high rates of mortality, morbidity, and hospitalizations. Acute diastolic heart failure represents approximately 40% to 50% of acute heart failure admissions as well as approximately half of patients with clinical heart failure,1 and the American Heart Association has projected the economic cost of HFpEF to increase to $47 billion by 2030.2 The number of acute diastolic heart failure admissions as a proportion of total heart failure admissions is expected to rise to 60% by 2020.3 Annual mortality ranges from 10% to 30%,4 and the 5-year survival rates for HFpEF after hospitalization for heart failure are estimated to be as high as 50% to 60%,5 which is in line with the survival estimates for heart failure with reduced ejection fraction (HFrEF). Symptomatically, patients with HFpEF have as much functional limitation and symptomatology as patients with HFrEF.6 The mortality rates are similar for both types of heart failure, with HFpEF nearly matching the in-hospital mortality rate of HFrEF; in fact, there is some evidence that although survival rates for HFrEF are improving, survival rates for HFpEF are not.7
Diastolic dysfunction is the key risk factor in the development of HFpEF. HFpEF is associated with multiple other risk factors, some innate and some acquired. Because diastolic dysfunction is considered to be a normal part of the aging process, it is unsurprising that the average age at time of diagnosis of HFpEF is 80 years.8 Other common comorbidities seen in HFpEF include hypertension, coronary artery disease, chronic kidney disease, diabetes mellitus, and depression.9 There is a mild female predominance among patients with HFpEF. The roles of metabolic syndrome, obesity, and sleep apnea/sleep-disordered breathing should not be understated, and the contributions of such disease to the previously listed comorbidities is significant.5,10
Diastolic dysfunction constitutes defects in diastolic relaxation, left ventricular filling, and/or left ventricular compliance, and often coexists with systolic dysfunction. When symptoms of heart failure occur in a patient with diastolic dysfunction and no systolic dysfunction, the term diastolic heart failure or heart failure with preserved ejection fraction may be used.11
Diastole is an active phase of the cardiac cycle involving a complex interaction of signaling pathways, structural changes, and energy usage. During diastole, these processes produce a combination of myocardial unwinding and elastic recoil of the left ventricle, which results in a decrease in the pressure in the left ventricle. Combined with the extent of shortening in the previous beat and the left atrial pressure, this active relaxation generates forward flow across the mitral valve, pulling blood into the left ventricle. The left atrial kick provides a finishing touch on left ventricular filling, supplying about a quarter of the total left ventricular filling volume. During exertion, as the heart rate increases, diastole shortens, and left ventricular filling becomes more reliant on the myocardium increasing its active relaxation to pull blood in from the left atrium even more quickly during the shortened diastole.
As could be surmised from the plethora of risk factors previously discussed, diastolic dysfunction and HFpEF are not simply a result of the elevation of filling pressures. Rather, the process is far more complicated. Various mechanisms have been uncovered or suggested by studies and have revealed that a variety of biochemical changes are at play.12 Neurohormonal activation and the effects of the sympathetic nervous system and renin-angiotensin-aldosterone pathways on cardiac function have been heavily studied. Newer evidence suggests that alterations in signaling pathways such as nitric oxide–cyclic guanosine monophosphate,13 endothelial dysfunction and microvascular inflammation,14 and myocardial energetics and calcium handling12 play large roles. Changes in myocyte structural proteins have also been found to occur in diastolic dysfunction: alterations in matrix metalloproteinases, desmin, microtubules, actin, myofilament and myosin function, and troponin binding and phosphorylation have been found. Pulmonary venous hypertension and peripheral arterial stiffness are often seen, although it is unclear whether these processes have more to do with the underlying risk factors or the process of diastolic dysfunction itself.
A complex interplay between diastolic dysfunction and the patient’s altered systemic milieu may lead to further changes, including abnormal exertional vasodilatation, chronotropic incompetence, pulmonary hypertension, and volume overload. In combination, this can lead to the clinical presentation of heart failure. Notably, the plethora of underlying changes that lead to diastolic dysfunction help to explain its heterogeneity in both clinical presentation and response to therapy.
Patients who develop diastolic dysfunction and HFpEF exhibit symptoms in large part because of impairment of these pathways. In HFpEF, the critical role of augmentation of cardiac unwinding forces, or pulling force, is impaired, and the time it takes to fully complete relaxation is prolonged.15 During periods of rest, this may not result in symptoms. During exertion, when heart rate increases and diastole shortens, relaxation may not be complete before the beginning of the next cardiac cycle. This results in compensation via elevated left atrial pressure, which produces worsened venous hypertension and pulmonary symptoms. With more advanced HFpEF, such symptoms can occur even with very mild exertion. In some cases of HFpEF, the chronically increased filling pressures will increase wall stress. The left ventricle remodels to combat this by thickening the left ventricular wall, resulting in concentric remodeling or concentric hypertrophy in many cases. Finally, the contribution of the atrial kick to left ventricular diastolic filling also may explain why some patients with HFpEF tolerate atrial fibrillation poorly.
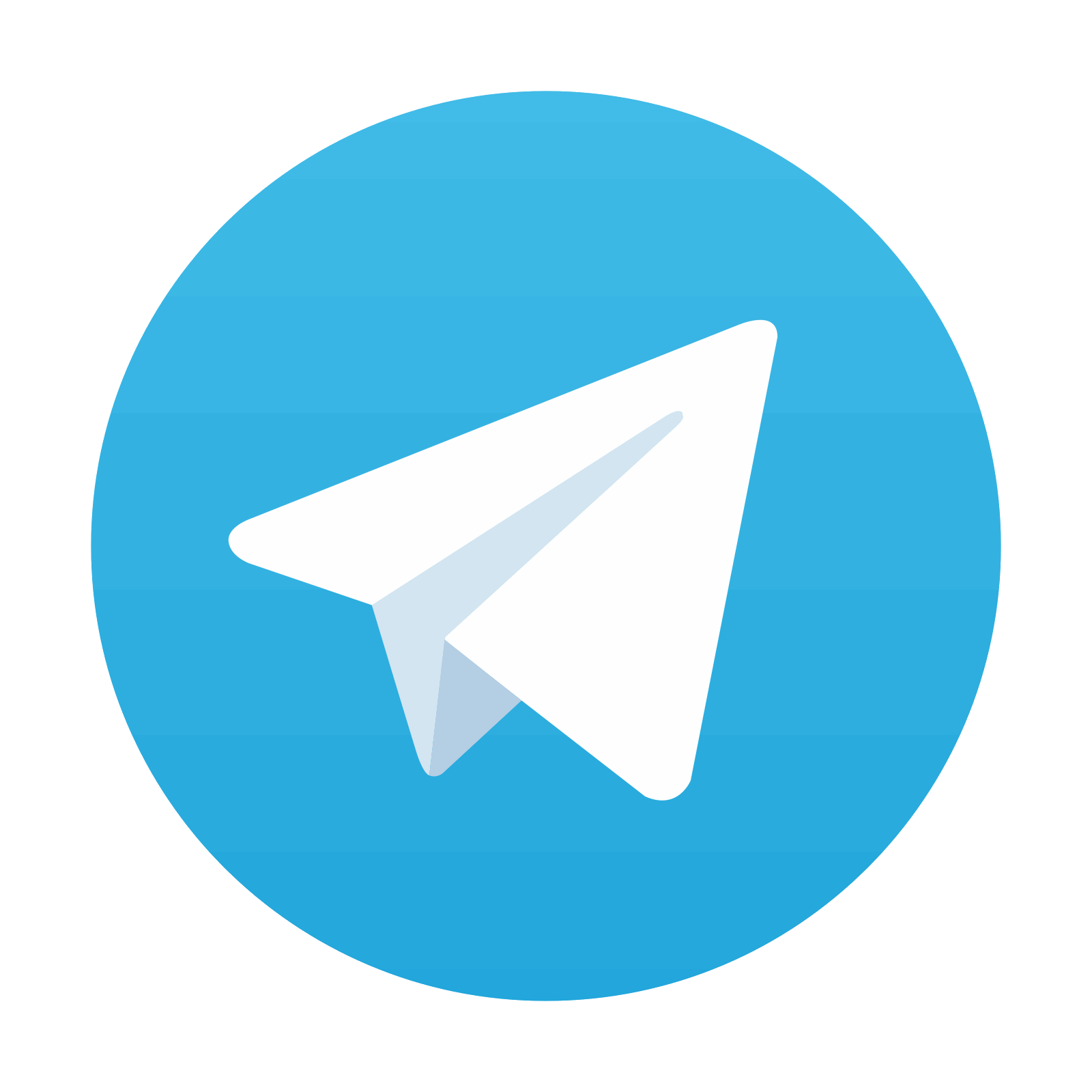
Stay updated, free articles. Join our Telegram channel

Full access? Get Clinical Tree
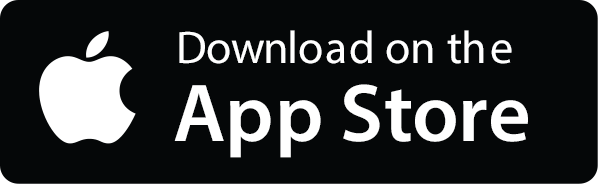
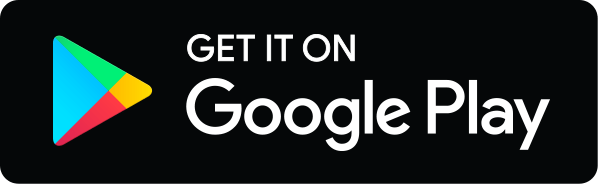