A 67-year-old man with past medical history of chronic obstructive pulmonary disease (COPD) presents with complains of cough, shortness of breath, and weight loss for 2 months. He has smoked 1 pack of cigarettes a day for the past 40 years. His primary care doctor obtains a chest radiograph, which shows a mass in the patient’s left upper lobe. How will you work up this patient? Learning Objectives: 1. What are the different symptoms and signs associated with lung cancer? 2. What are the different paraneoplastic syndromes associated with lung cancer? 3. What investigational methods are needed to diagnose lung cancer? Lung cancer is the leading cause of cancer-related death worldwide.1 The majority of lung cancer (~80%-85%) includes non–small cell lung cancer (NSCLC) histology, and the remainder is small cell lung cancer (SCLC). It is important to differentiate between NSCLC and SCLC as diagnostic evaluation and management differ. Most patients with lung cancer are symptomatic at presentation. Some present with a suspicious finding detected on chest imaging done for lung cancer screening, and some can present with an incidental finding on imaging done for other reasons. Lung cancer screening is recommended for early diagnosis in individuals at high risk2 but has had slow uptake.3 Of note, the cumulative risk tends to increase with age and with lifetime exposure to cigarette smoke (Figure 11-1). Figure 11-1. Smoking cessation and lung cancer. The goal of initial evaluation in a patient suspected of having lung cancer is timely diagnosis and staging so appropriate treatment can be administered. In this chapter, we review initial evaluation (history and physical examination) and diagnosis (imaging and pathology) for a patient with suspected lung cancer. The general approach in the diagnosis of lung cancer includes history and physical examination, imaging, biopsy, pathology, staging, and molecular testing. The majority of patients with lung cancer have advanced disease at clinical presentation. Symptoms may result from local effects of the tumor, from regional or distant spread, or from distant effects not related to metastases (paraneoplastic syndromes). Many symptoms are associated with lung cancer and are mostly non-specific. • Cough: It is the most common symptom and is usually present in 50%-75% of lung cancer patients.4,5 It is more common in squamous cell and SCLC due to its tendency to involve central airways. • Dyspnea: The incidence of dyspnea is 25%-50%.4,5 It can be caused by multiple factors, such as intrinsic or extrinsic compression, pulmonary embolism, pleural effusion, pneumonia, or lymphangitic spread. • Chest pain: The incidence of chest pain is 30%-50%,4 usually on the same side of the chest as the primary tumor. • Hemoptysis: The incidence of hemoptysis is about 20%-50%.4,5 Bronchitis is the most common cause of hemoptysis. • Hoarseness: The incidence of hoarseness is about 10%. This is due to malignancy involving the recurrent laryngeal nerve along its course under the arch of the aorta. • Weight loss: The incidence for weight loss is 36%. • Community-acquired pneumonia (CAP)6,7: Lung cancer can mimic CAP as a differential diagnosis or co-occur with CAP and present with pulmonary infiltrate and cough in about 1%-2% of cases. Lung cancer can metastasize to any part of the body and present with symptoms related to metastatic disease like bone pain or neurological symptoms (headache, vomiting, seizures, visual disturbances). In a metanalysis, hemoptysis was found to have the greatest diagnostic value for lung cancer, with a diagnostic odds ratio (DOR) of 6.39 (3.32-12.28), followed by dyspnea 2.73 (1.54-4.85), then cough 2.64 (1.24-5.64), and lastly chest pain 2.02 (0.88-4.60).8 Different signs associated with lung cancer include palpable lymphadenopathy (cervical, supraclavicular area), clubbing, superior vena cava syndrome, and Pancoast syndrome. Clubbing has an incidence of about 20% and a 3.9 likelihood ratio.9 Superior vena cava (SVC) syndrome has an incidence of 5%-10%. It is seen more with SCLC compared to NSCLC. Symptoms commonly include a sensation of fullness in the face/head and dyspnea. Physical findings include dilated neck veins, a prominent venous pattern on the chest, facial edema, and a plethoric appearance (Figure 11-2). Figure 11-2. Superior vena cava syndrome due to lung cancer causing SVC syndrome. (Adapted from Wilson LD, Detterbeck FC, Yahalom J. Clinical practice. Superior vena cava syndrome with malignant causes. N Engl J Med. 356(18):1862-1869.) • SVC syndrome grading system10: Lung cancers arising in the superior sulcus cause Pancoast syndrome, manifested by pain (usually in the shoulder and less commonly in the forearm, scapula, and fingers); Horner syndrome (ptosis, miosis, and anhidrosis); bony destruction; and atrophy of hand muscles. It is more commonly seen in NSCLC (Figure 11-3). Figure 11-3. Pancoast tumor in the superior sulcus. (Reproduced with permission from Sugarbaker DJ, Bueno R, Krasna MJ, Mentzer SJ, Zellos L. Sugarbaker’s Adult Chest Surgery. 3rd ed. New York, NY: McGraw Hill; 2020: https://accesssurgery.mhmedical.com/content.aspx?bookid=2781§ionid=239711663#239711671.) Paraneoplastic effects of tumor are remote effects that are not related to direct invasion, obstruction, or metastasis. While paraneoplastic syndromes are most often diagnosed in the setting of a known malignancy, it is common for a paraneoplastic disorder to develop before a cancer is identified. Therefore, search for underlying malignancy is important. In most instances, the tumor is revealed by computed tomography (CT) of the chest, abdomen, and pelvis. Hypercalcemia: Most patients with hypercalcemia have advanced disease, which is associated with poor survival. It may arise from bony metastasis and less commonly due to tumor secretion of parathyroid hormone–related protein. It is more commonly associated with squamous cell lung cancer followed by adenocarcinoma and then SCLC. Syndrome of inappropriate antidiuretic hormone secretion (SIADH) is frequently caused by SCLC.11 Incidence is about 10%. Symptoms are related to severity of hyponatremia. Treatment focuses on treating the malignancy. Hypertrophic pulmonary osteoarthropathy is defined by the presence of clubbing and periosteal proliferation of the tubular bones associated with lung cancer or lung disease causing symmetrical painful arthropathy. It is commonly caused by NSCLC. Incidence in lung cancer12 varies between 4% and 17%. Neurologic paraneoplastic syndromes are most commonly seen in lung cancer, especially SCLC. Incidence in SCLC is about 10%, most frequently Lambert-Eaton myasthenic syndrome (LEMS) (3.8%), sensory neuronopathy (1.9%), and limbic encephalitis (1.5%).13 The following well-categorized paraneoplastic antibodies and associated paraneoplastic syndromes seen in lung cancer14: • Anti-Hu (ANNA-1): Encephalomyelitis including cortical, limbic, and brainstem encephalitis; cerebellar degeneration; myelitis; sensory neuronopathy; and/or autonomic dysfunction • Anti-Ri (ANNA-2): Cerebellar degeneration, brainstem encephalitis, opsoclonus-myoclonus • Anti-CV2/CRMP5: Encephalomyelitis, cerebellar degeneration • Anti-PCA-2 (MAP1B): Peripheral neuropathy, cerebellar ataxia, encephalopathy • Anti-VGCC: cerebellar degeneration, LEMS Lambert-Eaton myasthenic syndrome may be seen in about 3% of SCLC patients, but almost 60% of patients with LEMS have SCLC. It serves as a marker for early disease and precedes the diagnosis of SCLC in most cases. It usually presents as complaints of slowly progressive proximal muscle weakness. There is no significant muscle atrophy, and deep tendon reflexes are almost depressed or absent. The aggressive search for a primary underlying malignancy is central to the management of patients with LEMS. Cushing syndrome secondary to ectopic production of corticotropin (ACTH) presents with muscle weakness, weight loss, hypertension, hirsutism, and osteoporosis. Also, it involves hypokalemic alkalosis and hyperglycemia. Incidence in SCLC is about 1%-5%, and patients with Cushing syndrome and SCLC together tend to have a worse prognosis.15 Every patient suspected of lung cancer should undergo thorough history and physical examination. Symptoms typically indicate advanced disease. Evaluation should be symptom directed, with particular attention to symptoms that might suggest metastasis. • Medical history: Pay attention to other medical conditions like COPD, renal disease. • Family history. • Social history with attention to family/social support and smoking history. • Performance status (Eastern Cooperative Oncology Group [ECOG] or Karnofsky performance scale). • Elicit history about bone pain, back pain, neurologic symptoms (headache, focal weakness, blurry vision, confusion, slurred speech, etc.). • Physical examination: • Symptom-directed evaluation prompts appropriate laboratory testing and imaging. Consider performing the following laboratory studies if the history/physical examination or chest imaging are suspicious for lung cancer • Complete blood count • Creatinine • Calcium • Electrolytes • Liver function test: alkaline phosphatase, alanine aminotransferase, aspartate aminotransferase, total bilirubin • Albumin No serum tumor markers have shown clinical utility in the diagnosis of lung cancer. If lung cancer is suspected based on signs/symptoms, the initial step is a CT of the chest and upper abdomen (including adrenal glands) with contrast.16,17 A patient suspected of having lung cancer due to lung cancer screening or an incidental finding will already have initial chest imaging. Magnetic resonance imaging (MRI) of the brain with contrast should be performed for all patients with SCLC16 and for stage IB, II, III, and IV cancer in patients with NSCLC.17 An 18F-fludeoxyglucose (FDG) positron emission tomographic (PET)/CT scan from the skull base to the midthigh should be considered in patients with suspected limited-stage SCLC16 and in NSCLC patients during the initial evaluation.17 Patients with symptoms suspicious of metastasis should receive dedicated imaging. • Back pain: MRI spine • Headache, confusion, blurry vision: Brain MRI (preferred); if unable to obtain an MRI, then CT head with contrast • Radicular back pain with focal weakness: MRI spine • Abdominal pain: CT abdomen/pelvis with contrast • Abnormal liver function test: CT abdomen/pelvis with contrast if liver not fully evaluated in CT chest For patients who presented with CAP, a chest radiograph should be performed at 6 weeks following treatment of high-risk patients for malignancy6,7: • Smokers • >50 years of age Tissue diagnosis is needed to diagnose lung cancer and differentiate between NSCLC (adenocarcinoma, squamous cell carcinoma) and SCLC. A biopsy that is the least invasive with the highest yield is preferred. Biopsy the site that would confer the highest stage, such as a biopsy of a suspected metastasis or mediastinal lymph node rather than the pulmonary lesion. Immunohistochemical (IHC) staining is used to classify NSCLC (adenocarcinoma, squamous cell carcinoma). Patients with suspected nodal disease should receive a biopsy by endobronchial ultrasound (EBUS), endoscopic ultrasound (EUS), navigational bronchoscopy, or mediastinoscopy.17 • EBUS provides access to nodal stations 2R/2L, 4R/4L, 7, 10R/10L, and other hilar nodal stations if necessary. • An EBUS transbronchial needle aspiration negative for malignancy in a clinically positive mediastinum should undergo subsequent mediastinoscopy prior to surgical resection. Lung cancer patients with an associated pleural effusion should undergo thoracentesis and cytology. A negative cytology result on initial thoracentesis does not exclude pleural involvement, and an additional thoracentesis should be considered before starting therapy with a curative intent. Multiple genetic alterations have been identified in NSCLC with approved targeted therapies. Molecular testing17 is recommended in all metastatic lung cancer. The National Comprehensive Cancer Network (NCCN) guidelines recommend testing for the following gene mutations as a part of broader molecular profiling with the goal of identifying rare driver mutations: • EGFR (epidermal growth factor receptor) • ALK (anaplastic lymphoma kinase) • ROS1 (ROS proto-oncogene 1) • BRAF gene • PD-L1 (programmed death ligand 1) Plasma cell-free/circulating tumor DNA (liquid biopsy) can be used in certain clinical situations for molecular testing: • If a patient is clinically unfit to receive invasive tissue biopsy • If a patient has no safe accessible site for invasive tissue biopsy • In the setting of insufficient tissue after the initial diagnostic setting Patients with suspected lung cancer, when possible, should be discussed in a multidisciplinary conference to move forward with the best approach for imaging and biopsy. A 68–year-old white male with a past medical history of malignant pleural mesothelioma is presented for discussion at a multidisciplinary lung tumor board meeting. The patient’s CT chest scan with intravenous contrast only showed nodular lobular thickening in the left lower lobe, and no thoracic lymphadenopathy was seen. CT of the abdomen and pelvis with intravenous contrast showed no suspicious lesions. The cardiothoracic surgeon would like to consider the patient for surgery. What is the best imaging test to order next? Learning Objectives: 1. What are the most common imaging modalities used in clinical practice? 2. What are the radiographic findings to suggest lung cancer in pulmonary nodules? 3. What are the characteristic radiographic features of SCLC? 4. For which lung cancer is a PET gallium scan the best to evaluate for metastatic cancer? 5. What is the lymphatic drainage pattern of left lower lobe lung cancer? Radiographic imaging is essential in the evaluation of patients with suspected lung cancer. Imaging tests are very useful in distinguishing benign pulmonary nodules from metastatic lung lesions. Also, it is very useful in clinical practice to follow up patients with thoracic malignancy. Oncologists often use imaging tests such as CT of the chest and PET/CT tests to follow cancer patients after they complete treatments. The main imaging modalities used in the initial diagnosis of lung cancers are chest x-ray and CT of the chest. A chest x-ray may show distinctive findings to suggest lung cancer. Chest CT is used to further characterize the chest x-ray findings. Once cancer is diagnosed by tissue biopsy, FDG PET/CT is often used for staging lung cancers. A bone scan with CT of the chest, abdomen, and pelvis may also be used for staging lung cancer. MRI is also used for patients who cannot tolerate iodinated contrast. Last, MRI is also used by cardiothoracic surgeons to evaluate patients for the possibility of surgical resection of localized malignant pleural mesothelioma.18 Patients who present with lung symptoms such as coughing and dyspnea are typically worked up starting with a chest x-ray (Figure 11-4). Chest x-rays allow an initial inspection of all lung structures, which often allows identification of radiographic abnormalities related to lung cancer. These include direct and indirect signs of cancer. Imaging findings of cancer include unexplained hilar lymphadenopathy, pleural effusions, atelectasis, infiltrates, and bone lesions.19,20 Figure 11-4. Chest x-ray. Chest CT is the most common imaging modality for the evaluation of lung cancers (Figure 11-5). CT images allow high spatial resolution for evaluation of pulmonary nodules, thoracic lymphadenopathy, pleural abnormalities, and locally invasive cancers. Metastatic bone lesions are also easily identified with this imaging modality. Figure 11-5. Chest CT with contrast visible in large vessels. In addition to diagnostic imaging, CT is valuable as a screening modality (Figure 11-6). Specifically, adult patients aged 55-80 with a 30 pack-year or more history of cigarette smoking who either actively smoke or have quit within 15 years are eligible for a low-dose CT (LDCT) of the chest for lung cancer screening. The difference between screening and standard non-contrast CT is in the radiation dose. The National Lung Screening Trial in 2011, for example, involved a LDCT dose of 2 millisievert (mSv), as compared to 8 mSv in standard CT scanning of the full chest.21 The concept in utilizing LDCT is to provide a high-quality screening test for patients at risk for lung cancer while exposing them to as minimal a dose of radiation as possible. Identifying lung nodules long before symptoms arise will then, ideally, lead to earlier diagnosis and subsequent management. Figure 11-6. Screening chest CT without contrast. Positron emission tomography with computed tomography is the current gold standard for staging of cancer (Figure 11-7). It is also used to evaluate undetermined pulmonary nodules for lung cancer. It is very important to remember that PET/CT does have limitations. Infectious and inflammatory diseases may lead to false-positive findings of high SUV. Also, slow-growing cancers with indolent features such as low-grade adenocarcinomas, bronchoalveolar carcinomas, and carcinoid cancers may exhibit a false-negative finding of low SUV. Figure 11-7. Chest PET/CT. Currently, PET/CT is routine in preoperative staging in order to avoid unnecessary surgery. To highlight an example of its importance, a 2011 study published in the New England Journal of Medicine found that, of 189 total patients (98 randomized to the PET/CT group and 91 to conventional staging), 60 underwent thoracotomy in the PET/CT group (61%), compared to 73 (80%) in the conventional group. Approximately 41% of thoracotomies were then found to be futile in the conventional group, compared to only 21% in the PET/CT group. The authors concluded that PET/CT in preoperative NSCLC staging reduced both total and futile thoracotomies.22 This study was followed by a study by Zelidat et al., who reported in 2014 on a cohort of 2,977 patients with NSCLC; there was a focus on 976 patient who underwent resection over an approximate 12-year period. Of patients who had surgery, 30.3% were later found to have distant metastases; however, the use of PET increased diagnosis of distant metastatic disease from 9% to 91% over this same period. By instrumental variable analyses, PET was associated with a reduction in unnecessary surgery (odds ratio 0.53, p = .004).23 Also, PET/CT may also be used in treatment response assessment, specifically when there is evidence or suspicion for either recurrence or metastatic spread of disease. The difficulty, however, is that PET/CT is often overused and may be misleading in some cases. Moreover, the efficacy of PET/CT in the context of formally assessing treatment response has not been established by clinical practice recommendations, making it difficult for physicians to justify its routine use.24 The role of PET/CT in lung cancer surveillance is also limited. A 2015 study published in the Journal of Nuclear Medicine, however, argued for its use when Antoniou et al. conducted a retrospective analysis of 261 patients from a single center with lung cancer, confirmed by biopsy. Within this population, 488 PET/CT scans were performed 6 months or longer from initial therapy completion; lung cancer recurrence was seen in 281 scans, while the other 207 demonstrated absence of disease. In a subpopulation of 245 patients in whom providers had no clinical suspicion of disease, PET/CT identified recurrence in 107 patients (43.7%). It was also found that overall survival was negatively correlated with a PET/CT study that demonstrated disease recurrence, as expected. Authors concluded that PET/CT could serve as a prognostic tool in following patients with lung cancer after primary treatment and could even assist in clinical judgment.25 And while the latter of these conclusions is interesting, larger scale studies are needed to validate the authors’ findings before PET/CT becomes a standardized component of lung cancer surveillance. Magnetic resonance imaging is a very valuable imaging modality for oncologists and surgeons (Figure 11-8). For example, it is very useful to evaluate thymic lesions. MRI can be used to help distinguish benign thymic lesions from cancerous lesions. Also, MRI is the best modality for identification of soft tissue invasion. Therefore, when a surgeon is considering a resection of a malignant mesothelioma, the surgeon can order an MRI to see if the cancer has penetrated through the diaphragm, which would preclude surgery.26 Figure 11-8. Chest MRI. Brain imaging is generally reserved for patients who demonstrate new neurologic symptoms, rather than as a routine screening modality (Figure 11-9). Given the propensity for intracranial extension of lung cancer, however, MRI of the brain can be a valuable tool for physicians. Most societal guidelines, including those put forth by the NCCN, European Society for Medical Oncology, and British Thoracic Society, recommend brain imaging in patients with stage III or greater NSCLC, but debate exists on how to screen in stages I-II. One group took a unique approach in a 2017 retrospective study that examined 585 patients who had undergone resection of diagnosed lung cancer, 471 of which had accessible radiographic records. Of the patients, 5.3% (25/471) had brain metastases on imaging, and 18/471 (3.8%) presented with metastases to the brain after surgical resection of lung cancer, 12 of which had adenocarcinoma. The authors concluded that preoperative brain MRI in patients with NSCLC, specifically those with adenocarcinoma histology, is indicated prior to surgery with curative intent. The idea here is that patients may be spared an invasive surgical procedure in favor of systemic therapy should intracranial metastases be discovered. Schoenmaekers et al. recently lauded the findings of this study in their own 2018 article published in the Journal of Thoracic Disease; the study recommended mandatory brain MRI in stage IIIA or higher NSLC or in those with stage II adenocarcinoma at a younger age.27,28 Figure 11-9. Brain with metastasis. Magnetic resonance has other utilities in the context of both primary and metastatic lung cancer. It is useful, for example, in visualizing Pancoast tumors, as it provides detailed imagery of the regional extent of the tumor and involved surrounding soft tissue. Magnetic resonance angiography (MRA) is particularly useful in highlighting nervous and vascular structures of the superior sulcus. MRI is also critical in imaging the spinal cord and surrounding structures, specifically in the setting of suspected cord compression secondary to spinal metastases. Detecting such compression in a timely manner allows physicians to enact timely medical management and/or surgical intervention, if indicated. Last, MRI has utility in the radiographic diagnosis of leptomeningeal disease, which occurs most commonly when solid cancer, such as that of lung, breast, or skin, disseminates into the cerebrospinal fluid (CSF). The ideal image in suspected cases is a T1-weighted MRI with gadolinium contrast, which in the brain will demonstrate pial nodularity and enhancement overlying areas that include the basal cisterns, cerebral convexities, or ependymal surfaces of ventricles. In the spine, MRI with contrast will reveal patchy nerve root involvement and extramedullary nodules within the dura, specifically at the level of the cauda equina. And, as useful as these high-resolution images are, obtaining CSF to observe for malignant cytology remains the gold standard for diagnosis in leptomeningeal carcinomatosis.29 This concept is best understood by recognizing that solid tumor, such as of the lung, may appear grossly on brain imaging, but hematogenous cancer (eg, acute lymphoblastic leukemia) will likely be radiographically negative but still very much involved in the CSF. A bone scan is also a very common radiographic test ordered by oncologists. It is a very sensitive nuclear medicine test to detect bone metastases. Metastatic bone cancers have increased radiotracer uptake. Lung cancers can be sclerotic or lytic. Squamous cell lung cancers can have mixed osteolytic and osteoblastic features. Lung adenocarcinomas typically are osteolytic. In this section, we discuss radiographic clues of lung cancer. We focus on chest CT because this is the most common radiographic imaging test used to characterize lung cancers. On a chest CT examination, there are many radiographic findings that are identified by reviewing the images ourselves or by reading the radiology report interpreted by a radiologist. These radiographic findings of lung cancer include pulmonary nodules, pulmonary masses, cavitation, ground glass opacity (GGO), encasement, and lymphadenopathy. A pulmonary nodule is defined as a round lesion less than 3 cm in maximum diameter (Figure 11-10). Common pulmonary nodule characteristics help distinguish benign versus malignant lesions. For example, a spherical shape is more likely benign. On the other hand, spiculated margins imply cancer. There are also internal traits of pulmonary nodules, including solidity, GGO, fat inclusion, and calcifications. Fat-containing pulmonary nodules are commonly seen in benign hamartomas. Calcification of pulmonary nodules may be benign or cancerous. Pulmonary nodules with stippled or eccentric calcifications are suspicious for cancer. Central and laminar calcifications on pulmonary nodules are likely benign. Figure 11-10. Chest CT with pulmonary nodule. A pulmonary mass is defined as a lesion greater than 3 cm in maximum diameter. Differential diagnosis of pulmonary masses includes primary lung cancer, metastatic lung cancer, lymphoma, carcinoid tumor, infection, inflammation, and vasculitis. Image findings of cancer include borders that are spiculated, cavitations with thick walls (described in material that follows), and invasive lesions. Other clues to suggest malignancy include a mass with associated hilar or mediastinal lymphadenopathy and ipsilateral pleural effusion.30,31 Cavitation is defined as a lucency within a pulmonary nodule, mass, or consolidation (Figure 11-11). The gas-containing space is due to underlying lung necrosis. Differential diagnosis of cavitations includes necrotizing pneumonia, abscess, squamous cell lung cancer, metastatic cancer, and vasculitis. The wall thickness of the cavitation can be a clue to whether the underlying cause is an infection or cancer. Cavity wall thickness of less than 7 mm is most likely benign. On the other hand, cavity thickness more than 2 cm is most likely neoplastic.30,31 Figure 11-11. Chest CT with cavitation. Ground glass opacity is defined as an increased density in an area in the lung that does not completely cover up the underlying structures (Figure 11-12). This is due to the fact that lung alveolus is filled with fluid, blood, or cancer cells. An alveolus can fill due to increased interstitial fluid, increased blood flow, or cancer cell invasion. Atypical infections, such as interstitial pneumonia caused by mycoplasma pneumonia, viral pneumonia, and pneumocystis jirovecii can also cause GGO. Interestingly, immunotherapies used in lung cancer can cause immunotherapy-associated pneumonitis that can be seen radiographically as GGO on CTs. Last, lung cancers can present as GGO. Lung adenocarcinomas that are lepedic or minimally invasive appear as GGO. Therefore, clinical history is very important to fully analyze GGOs.30,31 Figure 11-12. Chest CT with ground glass lesion. Encasement is due to surrounding a lung structure due to an underlying tissue (Figure 11-13). For example, aggressive cancers may encase and completely obstruct the SVC, pulmonary artery, or main stem bronchus. If cancers encase the SVC, this is known as SVC syndrome. Lung cancers that are commonly centrally located in the thorax such as SCLC and squamous cell lung cancer can also encase and obliterate the main bronchus or nearby large great vessels.32 Figure 11-13. Chest CT with lymphadenopathy encasing pulmonary vessels. Lymphadenopathy is defined as abnormal enlargement of a lymph node. CT findings include intrathoracic lymph nodes that measure more than 1 cm on CT. There is an associated FDG uptake within the lymph node on PET/CT. A good rule of thumb for abnormal lymph node size is location. For instance, a chest CT with a suspicious lung mass with an internal mammary lymph node, retrocrural lymph node, or extrapleural lymph node should always be considered abnormal and should be closely followed or biopsied. On the other hand, the other lymph nodes have location-specific upper limit normal size criteria. For instance, lower paratracheal and subcarinal lymph nodes greater than 1.1 cm, high paratracheal and superior mediastinal lymph nodes greater than 7 mm, right hilar and paraesophageal lymph nodes greater than 1.0 cm, left hilar and paraesophageal lymph nodes greater than 7 mm, and peridiaphragmatic lymph nodes greater than 5 mm should be closely monitored. Morphology of the lymph node may also be a clue to a cancerous lymph node. For example, abnormal lymph node features include rounded morphology, loss of fatty hilum, irregular borders, necrotic centers, and internal calcifications.30,31 Lung adenocarcinomas are the most common histologic type of lung cancer (Figure 11-14). On imaging, there are many types of lung adenocarcinomas. Adenocarcinoma in situ (CIS) and minimally invasive adenocarcinomas have a GGO or part-solid nodule less than 3 cm. Lepidic-predominant adenocarcinomas are part solid or purely GGO. Acinar or papillary with mucin production nodules are usually solid and may include GGO. Last, invasive mucinous adenocarcinoma may be multilobar or bilateral lung cancers. Figure 11-14. Chest CT and adenocarcinoma lung cancer. The most common radiographic findings of lung adenocarcinomas include peripheral or central mass, band-like features that look like fibrosis, postobstructive atelectasis or pneumonia, local invasion of mediastinum or chest wall, hilar or mediastinal lymphadenopathy, and ipsilateral pleural effusion. Also, interseptal thickening that is smooth or nodular can be present due to lymphangitic carcinomatosis. There are also radiographic prognostic indictors for lung adenocarcinomas. For example, if a lung adenocarcinoma has an extensive ground glass component, that is considered a favorable prognostic indicator. On the other hand, if the lung adenocarcinoma is predominantly solid, that is a poor prognostic indicator. For mixed masses, if the mass is composed of mostly GGO, that is a favorable prognostic sign. Other predictors of poor outcomes include spiculated lesions, thick cavitations, and concave pull into the cancer. Last, the larger the adenocarcinoma is, the likelihood of central nervous systemic metastatic disease is increased. Therefore, any patient with a lung adenocarcinoma that is greater than 4 cm should receive a brain MRI for further evaluation.33 Squamous cell lung cancer is thought to have developed from squamous metaplasia (Figure 11-15). Greater than 60% of these cancers arise in the main stem bronchus or lobar or main segmental branches. Apical lesions can also present as a Pancoast tumor. On radiographic x-rays, squamous cell lung cancer borders are commonly spiculated. Also, squamous cell lung cancers commonly exhibit internal cavitation or necrosis. Other common findings include a central airway mass with bronchial obstruction. This may manifest as postobstructive pneumonia in patients. On the other hand, the postobstructive pneumonia may also obscure an underlying cancer. Associated findings include wide mediastinum due to mediastinal lymphadenopathy. Figure 11-15. Chest CT with central squamous cell lung cancer and atelectasis. Computed tomographic findings for squamous cell lung cancer include central necrosis and cavitations. Of squamous cell lung cancers, 15% have cavitation. Squamous cell lung cancers typically have an internal cavity wall thickness of greater than 1.5 cm. Other clues of squamous cell lung cancers on CT include assessment of local invasion. Squamous cell lung cancers can invade mediastinal structures such as great vessels, esophagus, pericardium, and myocardium. In terms of chest wall invasion, if the lung cancer and pleura are more than 3 cm in contact, there is a high likelihood of tumor invasion. Lymphadenopathy is often a radiographic imaging clue to squamous cell lung cancer. Squamous cell lung cancers tend to have ipsilateral hilar lymph nodes greater than 1 cm on the short axis. Subcarinal lymph nodes greater than 1.2 cm, retrocrural lymph nodes greater than 0.8 cm, para-aortic lymph nodes greater than 0.8 cm, and pericardial lymph nodes greater than 0.8 cm are considered abnormal.33 Small cell lung cancer is the most commonly diagnosed as a central pulmonary nodule or mass (Figure 11-16). Mediastinal and hilar encasement are also very common. This includes invasion of pericardium, myocardium, pulmonary arteries, SVC, and aorta. Unlike other lung cancers, SCLC can present with only mediastinal or hilar lymphadenopathy without a lung mass. A peripheral pulmonary nodule or mass is not as common in SCLC. Most common finding of SCLC is a central pulmonary mass, which may produce atelectasis and volume loss. Also malignant pleural effusion may occur. In terms of extrathoracic metastases, the most common areas of metastases are liver, bone, adrenal glands, and brain. On initial diagnosis, 15% of SCLC patients that are asymptomatic have metastatic brain lesions. Thus, all SCLC patients should receive an initial brain MRI.33 Figure 11-16. Chest CT with central small cell lung cancer. Large cell lung cancers are often considered a diagnosis of exclusion. Therefore, there are very few features of SCLC, adenocarcinoma, or squamous cell lung cancers on histological examination. This cancer occurs most commonly in the lung periphery. On CT, large cell lung cancers are not that different from lung adenocarcinomas. Spiculated pulmonary nodules or masses are seen. Also, the pulmonary nodules often have eccentric or punctate calcifications. On PET/CT, large cell carcinomas have increased FDG uptake. Large cell lung carcinomas are rarely centrally located on imaging.33 Carcinoid tumors are low-grade malignant neuroendocrine cancers that typically do not metastasize to extrathoracic structures. These neuroendocrine cells arise from the bronchial epithelium. Most commonly, these cancers are found on imaging at the main stem, lobar, or segmental bronchi. They appear as a central hilar or perihilar well-defined mass. Metastases to liver or bone are very uncommon. Classic radiographic finding is seen with an expiratory air trapping that has a mosaic appearance on CT. Unlike other cancers, carcinoid tumors are not typically spiculated. These cancers typically have smooth pulmonary nodule borders. However, similar to other cancers, carcinoid tumors do spread to regional lymph nodes. The best imaging modality for atypical carcinoid is PET with gallium 68–labeled somatostatin. This is because neuroendocrine tumors overexpress somatostatin receptors. PET with gallium 68–labeled somatostatin analogue binds to the somatostatin analogue. This is the best imaging modality to identify metastatic atypical carcinoid cancer.33 Malignant pleural mesothelioma has a circumferential nodular pleural thickening. There is also loss of volume on the affected hemithorax. Calcified plaques occur in 25% of the cases. MRI is most sensitive for local invasion. In terms of pleural thickening, it is more than 1 cm thick. Also, pleural effusion is typically unilateral. On PET/CT, there is avid pleural thickening the pleura.34 Thymic carcinomas are malignant thymic epithelial cancers. It is difficult to distinguish thymic carcinoma from thymoma on CT imaging. On imaging, both cancers present as prevascular, circular soft tissue masses. These masses have smooth borders. There may be internal decreased density due to necrotic change. Invasive thymomas also commonly have pleural nodules. MRI is the best way to distinguish a benign thymic lesion from thymic carcinoma if tissue diagnosis cannot be obtained. Thymic carcinoma has a very characteristic MRI signal sequence that is not appreciated on benign thymic tissue.26 Teratomas are primary germ cell cancers that contain tissues derived from more than 1 germinal layer. Teratomas present as a well-defined circular anterior mediastinal mass with smooth borders. There is also a characteristic rim-like calcification in about 20% of the cases. The most characteristic finding is a fluid cyst that is thin walled (less than 7 mm thick) with septations. There may also be a fat-fluid level that is considered very diagnostic. Of note, teratomas rarely have lymphadenopathy. The best radiographic characteristics of a teratoma is a cystic mass that contains fat with a rim-like calcification. Lung cancers commonly spread to intrathoracic and extrathoracic structures. On CT, there are usually multiple circular or ovoid pulmonary nodules in both lungs. Interestingly, EGFR-positive adenocarcinomas can present with a bilateral pulmonary miliary pattern metastatic cancer (Figure 11-17). Other findings include endoluminal lesions, pleural metastases, cardiac metastases, tumor emboli, chest wall metastases, and lymphangitic carcinomatosis. In terms of metastatic pleural effusion, there is typically a nodular thickening of the pleural effusion. Also, malignant pericardial effusion presents as a nodular thickening.35 Figure 11-17. Chest CT with metastases to both lungs. Pancoast tumor presents as a lung mass in the pulmonary apex (Figure 11-18). Commonly there are rib destructions in the upper ribs. There are three main compartments in the lung apex: anterior, middle, and posterior. Figure 11-18. Chest CT with Pancoast tumor. The anterior compartment includes the subclavian vein. Therefore, if there is compression of the anterior compartment by a mass, this leads to SVC obstruction. Associated findings of this condition commonly include dilated SVC, mediastinal widening, and intraluminal thrombus. Enlarged mediastinal widening is due to increased size of the mediastinal vessels, including the azygos vein, superior intercostal vein, and brachiocephalic vein. Compression of the middle compartment leads to obstruction of the subclavian artery and portions of the brachial plexus. This leads to symptoms that include Horner syndrome. The posterior compartment has the brachial plexus roots. Compression of this compartment also leads to neurologic symptoms of the side of the body involved, which leads to smaller pupil, drooping eyelid, and decreased sweat production on the ipsilateral side.36 A lymph node map helps us evaluate lymph node metastases. There are 7 lymph node zones and 14 lymph node stations that can be assessed on CT. The 7 lymph node zones are the supraclavicular zone, superior mediastinal zone, aortopulmonary zone, subcarinal zone, inferior mediastinal zone, hilar and interlobar zone, and peripheral zone.37 • The supraclavicular zone includes station 1R and station 1L. This zone includes right and left cervical, supraclavicular, and sternal notch lymph nodes. • The superior mediastinal lymph zone (stations 2, 3, and 4) includes right and left upper paratracheal lymph nodes, prevascular lymph nodes, retrotracheal lymph nodes, right and left lower paratracheal lymph nodes. • The aortopulmonary zone (stations 5 and 6) includes the subaortic and para-aortic lymph nodes. • The subcarinal zone (station 7) includes the subcarinal lymph nodes. • The inferior mediastinal zone (stations 8 and 9) includes paraesophageal lymph nodes and pulmonary ligament lymph nodes. • The hilar and interlobar zone (station 10 and 11) includes hilar lymph nodes and interlobar lymph nodes. • The peripheral zone (stations 12, 13, and 14) includes the lobar lymph nodes, segmental lymph nodes, and subsegmental lymph nodes. Lung cancer generally spreads from the intrapulmonary lymph nodes to the regional hilar lymph nodes then to mediastinal lymph nodes. Specific patterns also vary by the lobe involved, as discussed in the following material. And, while these patterns are useful for radiation and/or surgical planning, many variations in the lymphatic extension of lung cancer exist, and an individualized approach should be taken in guiding management.37 • Right upper lobe primary cancer cells drain to the right hilar lymph nodes, then into the paratracheal and anterior mediastinal lymph nodes. • Left upper lobe primary cancer cells drain to the left hilar lymph nodes, then to the aorticopulmonary and para-aortic lymph nodes. • Right middle lobe primary cancer cells drain from the right middle lobe pulmonary node to the right hilar lymph nodes, then to the subcarinal lymph nodes and afterward to the right paratracheal and anterior mediastinal lymph nodes. • Right lower lobe primary cancer cells drain from right lower lobe pulmonary lymph node to right hilar lymph nodes, then to the subcarinal lymph nodes and afterward to the right paratracheal and anterior mediastinal lymph nodes. • Left lower lobe primary cancer cells drain from the left lower lobe pulmonary lymph node to the left hilar lymph node, then to subcarinal and aorticopulmonary lymph nodes.38 A 67-year-old ex-smoker with a 2-cm nodule in the right middle lobe is worked up for lung cancer. His CT shows hilar lymphadenopathy and a contralateral mediastinal adenopathy. The adrenal gland has a 3-cm lesion that lights up on PET as well as the lesions in the chest. What is the best way to obtain sufficient tissue to diagnose the nodule and help with treatment planning. Learning Objectives: 1. What are the different types of bronchoscopy? 2. Can bronchoscopy treat lung cancer? 3. What are the complications of bronchoscopy? Bronchoscopy is a minimally invasive procedure that allows access to the airways, mediastinum, and lung parenchyma. Bronchoscopy can be performed for both diagnostic and therapeutic purposes. While most bronchoscopy today is performed using a flexible fiber-optic bronchoscope (FOB), rigid bronchoscopy still plays an important role in the management of lung cancer–related complex airway problems. FOB can be performed with moderate sedation or general anesthesia and has a very good safety profile. In the last few decades, bronchoscopy has seen tremendous advances in both the therapeutic and the diagnostic arenas. The rigid bronchoscope was invented by Dr. Gustav Killian in 1898. Dr. Ikeda invented the flexible bronchoscope in 1967. In today’s day and age, this minimally invasive tool has become an integral part of thoracic oncology, aiding in diagnosis and staging of lung cancer and minimizing the need for invasive procedures. In this chapter, we discuss the diagnostic and therapeutic applications of bronchoscopy in lung cancer. Fiber-optic bronchoscopy is a very common procedure that involves intubation of the trachea with a flexible fiber-optic instrument. The FOB has a working channel that allows for instillation of topical anesthesia and suctioning of secretions and blood and facilitates the introduction of tissue sampling tools such as biopsy forceps, cytology brushes, needles for fine-needle aspirates or tissue cores, or therapeutic tools as mentioned further in the chapter. Complications of bronchoscopy include hypoxemia, pneumonia, bronchitis, bleeding and trauma to vocal cords or the tracheobronchial tree, and pneumothorax. The overall complication and mortality rates are extremely low, reported between less than 0.1% to 11% and 0% to 0.1%, respectively.39 The tracheobronchial tree is a 23-generation branching structure with the trachea being generation zero. A standard 5.9-mm bronchoscope with a 2-mm working channel only penetrates up to the fourth- to fifth-generation airways and allows visualization of the next 1-2 generations of airways.40 Thus, the majority of the tracheobronchial tree is beyond the visual range of standard bronchoscopy. Patients with known or suspected lung cancer with persistent atelectasis, unresolving pneumonia, or large pleural effusions should undergo bronchoscopy and airway inspection to evaluate for an obstructing endobronchial mass or stenosis/stricture of the central airways from radiation therapy. Similarly, hemoptysis in a patient with known or suspected lung cancer should be evaluated bronchoscopically to evaluate for endobronchial malignancy, radiation-induced tracheobronchitis, or tracheobronchial-vascular fistulas that may develop from the malignancy itself or radiation effects. Most standard bronchoscopy is performed using white light. White light bronchoscopy (WLB) can miss subtle endobronchial airway lesions, such as CIS. Narrow-band imaging (NBI) is a bronchoscopic modality that can improve detection of such lesions. The NBI technique involves decreasing the red wavelength and using the blue and green wavelengths of the light spectrum to enhance the superficial mucosal and deeper submucosal blood vessels, respectively. This depicts the angiogenesis associated with a malignant lesion. This technique has been used effectively in gastroenterology and now is being employed in bronchoscopy as well. In one study, NBI detected dysplasia or malignancy in 23% of the patients with normal WLB.41 In another study, NBI performed after WLB led to a change in therapy in 10% of the patients.42 The pattern of vascularization seen on NBI has been shown to correlate with the pathologic features of angiogenic squamous dysplasia.43,44 In bronchology, the term endobronchial generally refers to a lesion that can be visualized during bronchoscopy. Endobronchial tissue sampling can be performed using biopsy forceps and various gauge needles for needle aspirates or tissue cores. Cytology brushes can be used for endobronchial tissue sampling but are only able to capture the superficial parts of such lesions, which are often necrotic, increasing the likelihood of a non-diagnostic sample. The sensitivity and specificity of bronchoscopically visible lesions is 88% to 100%.45 Among different endobronchial tissue-sampling modalities, endobronchial biopsies have the highest sensitivity (74%), while cytology brushings and bronchial washings show poorer sensitivity at 59% and 48%, respectively.46 The combination of all three modalities increases the sensitivity to 88%.46 Thus, whenever feasible multiple sampling techniques should be utilized to obtain an adequate amount of diagnostic material, an issue that is even more important in the era of precision medicine. Fluoroscopy-guided transbronchial tissue sampling is used for lesions in the lung parenchyma and thus involves accessing pathologies that are “beyond visual range” of the bronchoscopist. These lesions can be pulmonary nodules, masses, or pulmonary infiltrates. The underlying etiology of these lesions is infectious, inflammatory, or neoplastic depending on the particular clinical context. The particular lobe of the lung and corresponding segment are identified during preprocedure review of chest imaging. During the procedure, the bronchoscope is advanced to the particular lobe and corresponding segment of concern. The tissue-sampling tool (biopsy forceps, cytology brushes, or aspiration needles) are then passed through the working channel of the bronchoscope and advanced under fluoroscopic guidance to access the parenchymal lesion. To maximize diagnostic yield and obtain abundant tissue for molecular analysis, a combination of sampling modalities is used, and multiple samples are usually obtained whenever feasible and safe. Transbronchial biopsies are generally safe, with a mortality rate of less than 0.04% and an overall complication rate of up to 6%.47,48 Pneumothorax is the most common complication seen with transbronchial biopsies, with a rate of 5.8% in one study.48 Transbronchial tissue sampling involves operating beyond the visual range of the bronchoscopist using a 2-dimensional fluoroscopic view for guidance. As a result, the ability of a bronchoscopist to guide the sampling tool through the airways and the certainty of the relation of the tool to the parenchymal lesion as seen on the fluoroscopy view is decreased. While this factor is of limited concern in diffuse parenchymal processes such as pneumonia and pneumonitis, it can significantly compromise diagnostic yield in more focal lung lesions, such as pulmonary nodules and masses. Hence, the diagnostic yield of transbronchial sampling ranges widely, from 36% to 88%.45 Various factors have been shown to affect the diagnostic yield, including the sampling method (forceps biopsy, cytology brushing, bronchoalveolar lavage [BAL]); number of samples taken; lesion size; and presence or absence of an airway leading to the lesion on chest CT scan.45 Of these, the lesion size has the greatest impact on yield (63% for lesions greater than 2 cm and 34% with lesions less than 2 cm). The diagnostic yield of CT-guided transthoracic needle aspirate (CT-TTNA) is higher at 90% compared to fluoroscopy-guided bronchoscopic biopsy.45 However, CT-TTNA comes with a much higher complication rate of 15% for pneumothorax and 1% for hemorrhage (of these, 18% need a blood transfusion) based on cross-sectional analysis of 15,865 adults who had undergone CT-TTNA.49 Over the last 2 decades, guided bronchoscopic techniques such as EBUS, electromagnetic navigation bronchoscopy, virtual bronchoscopy, and others have evolved to help bronchoscopists overcome the limitations of fluoroscopy-guided peripheral pulmonary tissue sampling and improve diagnostic yield. These techniques are discussed further in the chapter. Bronchial washings and BAL are performed by instilling fluid (usually normal saline) through the working channel of the bronchoscope and then aspirating to collect a specimen. The fluid can be instilled either on an endobronchial lesion (bronchial washing) or into the distal airways and alveolar tissue (BAL) after wedging the scope in a segment of the lung. Bronchial washing/BAL alone has a poor sensitivity for diagnosis of endobronchial lung cancer (48%) and even lower sensitivity (29%) for peripheral lung cancer.45,50 In 1 study, only 1% of the lung cancer diagnoses would have been missed in the absence of BAL, and ground glass lesions were not associated with increased BAL yield.50 The main role of BAL in lung cancer is in the evaluation of patients who present with respiratory distress and pulmonary infiltrates on chest imaging in the setting of chemoimmunotherapy. In this situation, BAL can help diagnose an underlying infection or, by demonstrating negative cultures, favor a diagnosis of pneumonitis, whereby treatment with corticosteroids may be indicated.51 Curvilinear EBUS (or convex probe EBUS) is a modification of the standard bronchoscope that includes an ultrasound transducer at the distal end of the bronchoscope (Figure 11-19). The ultrasound allows the visualization and sampling of structures outside the central airways. Malignant lymph nodes classically appear on ultrasound as round, heterogeneous structures with well-enhanced margins and loss of normal intranodal hilar structures (Figure 11-20). An aspiration needle is inserted through the working channel of the scope and exits at the distal end of the scope, making real-time sampling (called EBUS transbronchial needle aspirate or EBUS-TBNA) of central mediastinal masses and mediastinal and hilar lymph nodes possible (Figure 11-21). Since its introduction about 2 decades ago, curvilinear EBUS has radically changed the approach to mediastinal staging for lung cancer. Pooled analysis of 26 studies including 2,756 patients showed a sensitivity of 89%, specificity of 100%, positive predictive value of 100%, and negative predictive value of 91% for lung cancer.52 These were significantly better than conventional unguided transbronchial needle aspiration. Figure 11-19. Distal end of a curvilinear endobronchial ultrasound scope showing the ultrasound transducer. Figure 11-20. Endobronchial ultrasound view of a typical malignant lymph node with rounded shape, heterogeneous internal echotexture, loss of normal central hilar structure, and a distinct hyperechoic margin. Figure 11-21. Real-time endobronchial ultrasound-guided transbronchial lymph needle aspiration of a malignant lymph node. The aspiration needle is seen entering diagonally into the lymph node. Endobronchial ultrasound can provide access to the bilateral upper and lower paratracheal, subcarinal, and bilateral hilar lymph nodes. Combined EUS and fine-needle aspiration (EUS-FNA) and EBUS-TBNA (“medical mediastinoscopy”) can provide access to inferior pulmonary ligament and esophageal lymph nodes, thus expanding the horizon of minimally invasive lung cancer staging. This medical mediastinoscopy can be performed in a single setting with a single scope using either moderate sedation or general anesthesia on an outpatient basis, thus saving time and cost.53,54 Pooled analysis of 7 studies (811 patients) showed a sensitivity of 91%, specificity of 100%, positive predictive value of 100%, and negative predictive value of 96% for the combined approach, better than either approach alone.52 A multicenter randomized controlled trial of 241 patients compared mediastinoscopy alone for lung cancer staging to combined EBUS/EUS.53 The patients underwent mediastinoscopy if the EUS/EBUS approach was negative. The sensitivities for mediastinoscopy, combined EBUS/EUS, and mediastinoscopy following a negative EBUS/EUS were 79%, 85%, and 94% respectively. The non-curative resection rate was reduced from 18% in the mediastinoscopy group to 7% (p < .02). The conclusions from this study were that patients should undergo EBUS/EUS staging first and if negative move on to mediastinoscopy. Many studies have demonstrated cost savings with EBUS by reducing unnecessary mediastinocopies and other surgical procedures.55–57 These savings persist even if EBUS is performed with general anesthesia.55 Recent studies have shown that the EBUS scope can be used to sample the left adrenal gland and thus possibly allow evaluation of adrenal metastasis from lung cancer.58,59 The EBUS-TBNA samples have been found to be adequate for molecular analysis of non–small cell carcinoma. A systematic review and meta-analysis of 28 studies found a pooled probability of 94% for obtaining a sufficient sample for EGFR and ALK mutations.60 EBUS samples have been found to be adequate for PD-L1 testing as well with an adequacy rate of 86%-90%.61,62 EBUS needles are available as 21G, 22G, 25G cytology needles as well as a 19G histopathology needle. Multiple studies have been published to evaluate and compare the diagnostic yield and sample adequacy of these needles.63 The studies showed good diagnostic yield with all available needles but were not conclusive enough to recommend one or another. Moreover, an EBUS microforceps needle has been introduced that can allow tissue biopsies. Multiple studies have shown feasibility and promising results with the miniforceps.63 Finally, EBUS has an excellent safety profile. Herth et al. studied the performance characteristics of EBUS in 124 patients with tissue-proven stage IIIA-N2 disease undergoing restaging after neoadjuvant chemotherapy.64 The sensitivity, specificity, positive predictive value, negative predictive value, and diagnostic accuracy of EBUS in this patient population were 76%, 100%, 100%, 20%, and 77%, respectively. Of the 35 patients with no nodal metastases on EBUS-TBNA, 28 were found to have residual disease at thoracotomy. Because of a low negative predictive value in patients undergoing restaging after neoadjuvant chemotherapy, a negative result with EBUS should be confirmed surgically. In the ACCP (American College of Chest Physicians) Quality Improvement Registry, Evaluation, and Education (ACQuIRE) registry that includes 1,317 patients undergoing EBUS in 6 different hospitals, the overall complication rate was less than 1%, and the pneumothorax rate and major bleeding rate were 0.2% respectively.65 Guided bronchoscopic techniques for peripheral pulmonary lesions (PPLs) are technologies that allow the bronchoscopist to visualize and navigate the airways beyond the visual range of the standard bronchoscope, potentially increasing access to peripheral lung lesions and improving diagnostic yield. These technologies include radial probe endobronchial ultrasound (RP-EBUS), electromagnetic navigation bronchoscopy (ENB), and virtual bronchoscopy with ultrathin bronchoscopy (VB/UB). Radial probe EBUS uses a thin, flexible ultrasound probe (Figure 11-22) that can be passed through the working channel of the bronchoscope and advanced to the peripheral lung tissue to provide a 360° ultrasonic view of the lung tissue. Normal lung tissue has a characteristic “snowstorm” appearance on lung ultrasound. PPLs appear heterogeneous with a bright hyperechoic margin if the RP-EBUS probe is within or near a lesion (Figure 11-23). RP-EBUS probe is usually used within a guide sheath as a single unit. Once the PPLs have been located, the RP-EBUS probe is removed, leaving the guide sheath in place. The guide sheath acts as an extended working channel (EWC) to allow sampling of PPLs with forceps, brushes, and needles. The biggest disadvantage of RP-EBUS is the inability of the bronchoscopist to maneuver the probe in the peripheral smaller and tortuous airways. This is why this technique is often combined with ENB to provide better navigation and maneuverability. Figure 11-22. Radial EBUS probe. Figure 11-23. An RP-EBUS view of a malignant lung nodule. The dark central circle represents the RP-EBUS probe in a central position relative to the nodule. Notice the heterogeneous lesion and bright margin of the lesion around the probe. While studies have shown a diagnostic yield of around 70% with RP-EBUS alone, real-world experience has been less impressive, with yields around 57% in the ACCP ACQuIRE bronchoscopy registry.66,67 The biggest predictor of yield is the location of the RP-EBUS probe in relation to the lesion, with a more central location (as shown in Figure 11-23) associated with higher yields.40 RP-EBUS probe can also be used to study tumor invasion of the tracheobronchial wall as in centrally located lung malignancies. This can help evaluate whether these central tumors are actually invading the trachea or simply abutting the central airways without actual tissue invasion. Herth et al. studied 131 consecutive such patients and found RP-EBUS had a sensitivity of 89%, specificity of 100%, and accuracy of 94% for assessing tumor invasion.68 RP-EBUS performed better than CT in this study. Electromagnetic navigation bronchoscopy involves uploading the patient’s CT to planning software. The computer uses the information from the axial, coronal, and sagittal views of the CT to generate a virtual bronchoscopy view and a 3-dimensional tracheobronchial tree (Figure 11-24). The bronchoscopist selects the target of interest and locates any airway that may lead to the target on the CT. The planning software then generates a pathway from the central airways to the lesion. During bronchoscopy, an EWC that houses a steerable locatable guide (LG) is used to travel through the airways while the computer tracks the position of the LG in an electromagnetic field that surrounds the patient and provides instructions on where to make the turns. Once the LG is within a centimeter of the lesion, the LG can be removed, leaving the EWC in place. When combined technologies are utilized, an RP-EBUS probe can then be passed through the EWC at this point to obtain a real-time image of the lesion. The position of the probe is correlated with the patient’s chest imaging and fluoroscopic view. RP-EBUS probe is then removed and samples obtained through the EWC using needles, brushes and biopsy forceps. The diagnostic yield of ENB with or without RP-EBUS has been very variable, ranging from 38.5% to 74%.66,67,69 This wide variation in diagnostic yield can be due to a wide range of motion of pulmonary nodules during the respiration cycle, as shown in study by Chen et al.70 In this study, the average motion of all nodules was 17.6 mm, with lower lobe nodules having greater motion than upper lobe nodules. Ongoing technologic advances in navigation bronchoscopy promise more accurate and real-time sampling of PPLs in the near future. Figure 11-24. Electromagnetic bronchoscopy screen as seen during a procedure. The “green ball” represents the center of the lesion. Virtual view and triplanar CT views are depicted simultaneously. Virtual bronchoscopy with ultrathin bronchoscopy involves using planning software to reconstruct a virtual tracheobronchial tree. This can be combined with UB with a scope with an outer diameter of 2.8 mm that can be advanced under virtual guidance to visualize up to the ninth-generation airway. Diagnostic yields around 70% have been reported in the literature.67 The small size of the tissue samples obtained with UB would be concerning when considering molecular analysis for lung cancer.40 Guided bronchoscopy has a good safety profile, with a pneumothorax rate of 1.5% in a meta-analysis of 39 studies including 3,052 patients.67 The therapeutic role of bronchoscopy in lung cancer includes management of complications caused by the tumor or therapies. These problems can include hemoptysis, trachea-broncho-esophageal fistulas and airway stenosis from tumors or postradiation strictures (Figures 11-25 through 11-31). Bronchoscopy can also be used to assist in radiation therapy for lung cancer by placing fiducial markers and endobronchial brachytherapy catheters. Bronchoscopic intratumoral use of various antitumor agents has been tried in the past.71 Bronchoscopic radio-frequency ablation (RFA) of lung cancer is also on the horizon.71 A detailed discussion of the different bronchoscopic therapeutic modalities is beyond the scope of this book. Table 11-1 highlights some of the common indications and available therapeutic interventions. Figure 11-25. Postradiation scarring of left upper lobe anterior segment bronchus. Figure 11-26. Close-up view of left upper lobe showing web-like postradiation scar. Figure 11-27. Left upper lobe bronchus after bronchoscopic electrocautery and balloon dilation showing normal-appearing bronchial orifice. Figure 11-28. Large endobronchial malignant tumor in distal trachea causing near-complete obstruction of trachea and left main stem bronchus. Figure 11-29. Distal trachea and bilateral main stem bronchi completely patent after tumor debulking with electrocautery. Figure 11-30. Large defect seen in medial wall of left main stem bronchus caused by tumor infiltration and radiation therapy. Figure 11-31. The same area after hybrid covered airway stent placement. TABLE 11-1 Therapeutic Bronchoscopy and Indications in Lung Cancer
11
DIAGNOSTICS OF LUNG CANCER
CLINICAL TESTING
CLINICAL MANIFESTATIONS
Symptoms
Signs
Grade 0: Asymptomatic—Radiographic SVC obstruction in the absence of symptoms
Grade 1: Mild—Edema in head or neck (vascular distention), cyanosis, plethora
Grade 2: Moderate—Edema in head or neck with functional impairment (mild dysphagia; cough; mild or moderate impairment of head, jaw, or eyelid movements; visual disturbances caused by ocular edema)
Grade 3: Severe—Mild or moderate cerebral edema (headache, dizziness); mild/moderate laryngeal edema; or diminished cardiac reserve (syncope after bending); incidence 10%
Grade 4: Life threatening—Significant cerebral edema (confusion, obtundation), significant laryngeal edema (stridor), or significant hemodynamic compromise (syncope without precipitating factors, hypotension, renal insufficiency); incidence 5%
Pancoast Syndrome
Paraneoplastic Syndromes
HISTORY AND PHYSICAL EXAMINATION
Evaluate for hypoxia.
Look for lymphadenopathy, finger clubbing.
Auscultate lung fields (evaluate for pleural effusion).
Every patient should undergo a brief neurologic examination; if neurologic symptoms are present, then perform a focused detailed neurologic examination to evaluate for brain metastasis and spinal cord compression.
TESTING FOR LUNG CANCER
Laboratory
Imaging
Biopsy
Molecular Testing
Exon 19 deletions or exon 21 L858R mutations are the most common mutations and are associated with responsiveness to EGFR tyrosine kinase inhibitor.
EGFR exon 20 insertions and p.T790M are less common and associated with lack of responsiveness to EGFR tyrosine kinase inhibitor.
IMAGING OF LUNG CANCER
IMAGING MODALITIES
Chest Radiography
CT Chest
Screening CT
Positron Emission Tomography/Computed Tomography
Preoperative PET, Response Assessment, Surveillance
Magnetic Resonance Imaging
Use of MRI for Brain Lesions, Pancoast Tumors, Spinal Metastases to Rule Out Cord Compression and/or Leptomeningeal Disease
Bone Scan
RADIOGRAPHIC CLUES OF LUNG CANCER
Pulmonary Nodule
Cavitation
Ground-Glass Opacity
Encasement
LUNG CANCERS
Lung Adenocarcinoma
Squamous Cell Lung Cancer
Small Cell Lung Cancer
Large Cell Carcinoma
Carcinoid Tumors
Malignant Pleural Mesothelioma
Thymoma and Thymic Carcinomas
Teratoma
Metastatic Cancer
Pancoast Tumor
LYMPH NODE METASTATIC PATTERN IN LUNG CANCER
Lymph Node Map
LUNG CANCER LYMPHATIC DRAINAGE PATTERN
BRONCHOSCOPY IN LUNG CANCER
FIBER-OPTIC BRONCHOSCOPY
Airway Inspection
Endobronchial Tissue Sampling
Transbronchial Tissue Sampling Under Fluoroscopic Guidance
Bronchial Washings and Bronchoalveolar Lavage
Curvilinear Endobronchial Ultrasound
Guided Bronchoscopic Techniques for Peripheral Pulmonary Lesions
THERAPEUTIC BRONCHOSCOPY IN LUNG CANCER
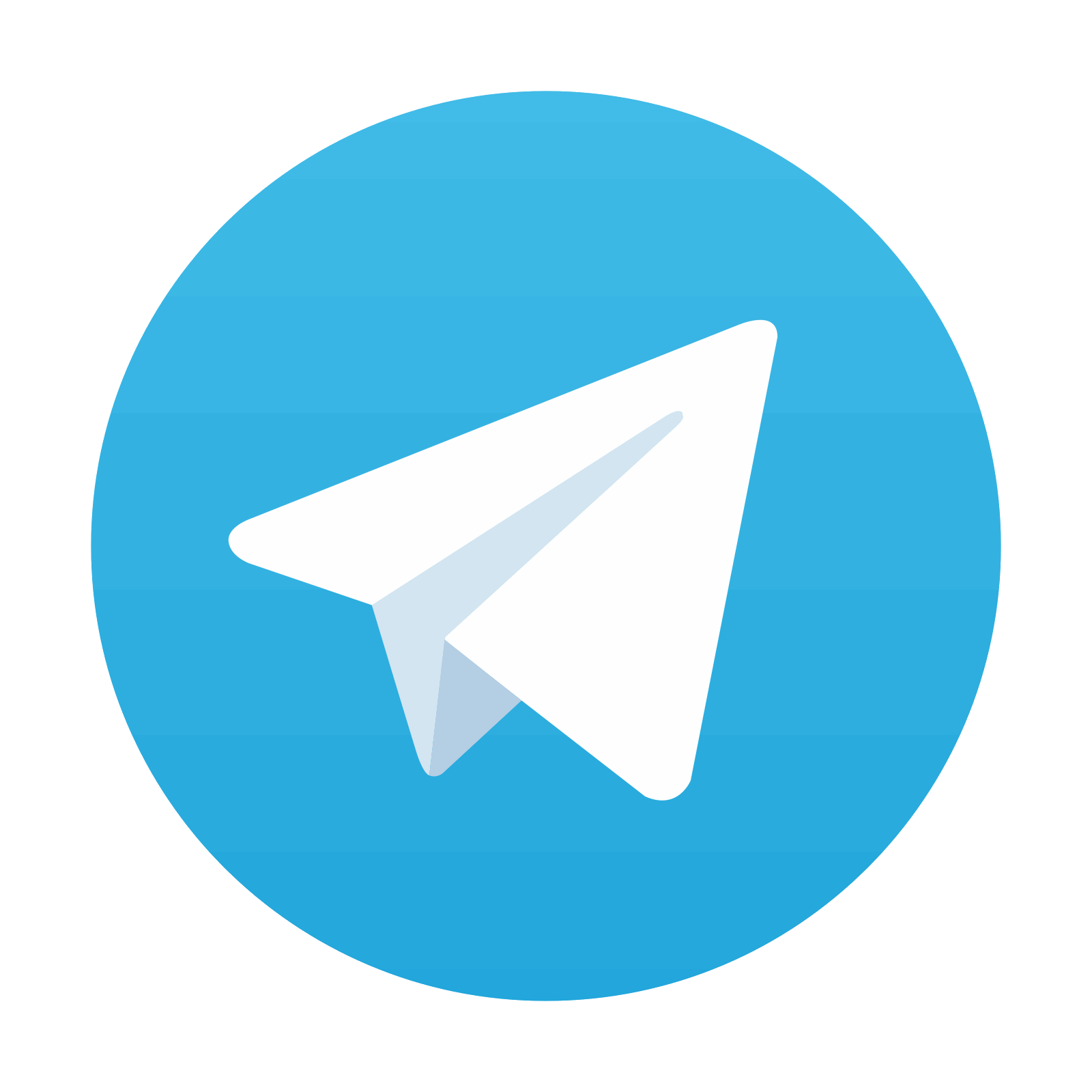
Stay updated, free articles. Join our Telegram channel

Full access? Get Clinical Tree
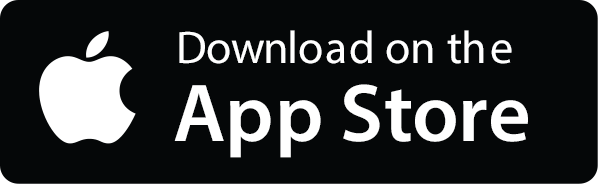
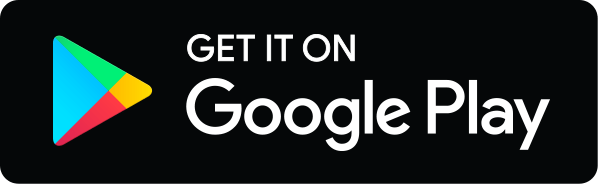