(1)
Department of Surgery, Northwestern University Feinberg School of Medicine, 676 N. St. Clair Street, Suite #650, Chicago, IL 60611, USA
(2)
Department of Surgery, University of North Corolina, Chapel Hill, NC, USA
Keywords
Ankle-brachial indexToe pressureContinuous wave DopplerSegmental limb pressurePulse volume recordingArterial duplex ultrasoundComputed tomography angiographyMagnetic resonance angiographyDigital subtraction angiographyIntroduction
Critical limb ischemia (CLI), if left untreated, is associated with a high risk of limb loss [1–3]. Before revascularization can be performed, a thorough but efficient diagnostic approach is warranted. The diagnostic process begins with an initial clinical evaluation to assess for the presence of peripheral artery disease (PAD ). Any underlying comorbidities the patient has must be identified as they will influence decisions regarding the diagnostic evaluation. CLI is manifested by rest pain and/or tissue loss of the lower extremity but is also an indicator of atherosclerotic disease in other vascular beds that increases the patient’s risk of cardiovascular events [4–8]; this along with other comorbidities will determine the patient’s risk of revascularization. The process then proceeds to diagnostic studies to confirm the presence of PAD , localize the lesions that need treatment, and finally plan a revascularization procedure if indicated [1]. With the recent explosion of treatment modalities for PAD, there has been an equal development of imaging modalities available to delineate the patient’s vascular anatomy prior to revascularization [9, 10]. Noninvasive vascular lab studies are used to determine the hemodynamic significance of the patient’s vascular lesions [3]. Anatomic imaging by arterial duplex ultrasound, computed tomography angiography (CTA ), magnetic resonance angiography (MRA ), or catheter-based digital subtraction angiography (DSA ) can then be used to plan a revascularization procedure [3, 10–13]. The best imaging study to obtain depends on the patient’s underlying comorbidities, distribution of disease, and institution-specific imaging capabilities (Fig. 15.1).


Fig. 15.1
Diagnostic decision tree for patients with critical limb ischemia
Clinical Diagnosis
Each CLI patient should undergo a thorough history and physical examination to establish a clinical diagnosis of CLI, determine the etiology of CLI, and assess suitability for revascularization prior to proceeding with further diagnostic imaging [1, 3, 14, 15]. The clinical evaluation should focus on the patient’s symptoms , cardiovascular risk factors, and comorbidities [1]. Patients with CLI will complain of rest pain or have tissue loss of their lower extremities [14]. The duration of symptoms and status of tissue loss are important indicators when determining the urgency of further workup and need for revascularization [2]. A time course of peripheral vascular symptoms can also be helpful in establishing a diagnosis of PAD or other etiologies for CLI such as embolic disease [1]. Patients with PAD will often (but not always) have pre-existing symptoms of claudication. These patients are at significant risk of having atherosclerotic disease in other vascular beds that contributes to cardiovascular morbidity and mortality. Furthermore, they often have other comorbidities that may require diagnostic consideration in tandem with the CLI workup [4–8]. Diabetes mellitus, renal insufficiency, decreased cardiac output, and a smoking history are commonly found in patients with CLI and also contribute to poor microvascular blood supply [1, 16]. This information is critical to determine the patient’s ability to tolerate a revascularization procedure [1, 14]. These comorbidities will also influence which imaging modality is best suited to each patient individually. Lastly, the functional status of the patient should be assessed along with their living situation and ambulatory status to determine the risk/benefit ratio of open revascularization, endovascular revascularization, primary amputation, or best medical management [1, 3, 14].
On physical examination , CLI patients require an assessment of the cardiovascular system with a systematic examination of pulses and evaluation of tissue perfusion to establish the level of obstructive lesions. All pulses should be palpated and recorded, including the common femoral, popliteal, dorsalis pedis , and posterior tibial arteries. An ankle-brachial index (ABI) should be measured at the bedside even if a pulse is palpated as it is a much more objective measure that quantifies blood flow to the limb [14, 17–19]. Signs of severe chronic ischemia include dependent rubor, pallor of the extremity with elevation, reduced capillary refill, and a paucity of hair. The skin should be evaluated for signs of atheroembolization; the characteristic livedo reticularis is suggestive of an embolic source from the heart, proximal arterial aneurysm, atherosclerotic plaque, or hypercoagulable state that may require further diagnostic consideration [1]. The feet and legs should be meticulously inspected for wounds. When tissue loss is encountered, it is important to determine if the underlying etiology is due to arterial disease, venous disease, or pressure-related problems or if it is multifactorial [1]. Careful attention must be paid to signs of infection, and the clinician must have a low threshold for administration of antibiotics and early debridement [14]. The extent of tissue loss and involvement of deep structures are an important factor in determining if the limb is salvageable [2]. If deemed a candidate for revascularization, patients with CLI should undergo an expedited evaluation for revascularization as this condition is associated with high risk of major limb amputation [1, 3]. Further diagnostic evaluation depends on the patient’s comorbidities, characteristics of each imaging modality, and the technical expertise of the given institution [10].
Diagnostic Testing
The objective of diagnostic testing in patients with CLI is to confirm the presence of PAD, identify the distribution and hemodynamic significance of disease, and provide anatomic information to plan a revascularization procedure [1, 3, 14]. Diagnostic tests can be grouped into three broad categories: (1) hemodynamic/physiologic measurements, (2) tissue perfusion, and (3) anatomic imaging. Most patients with CLI should undergo a noninvasive physiologic vascular laboratory study and an anatomic imaging study prior to revascularization. Anatomic imaging of the vascular tree is required to plan a revascularization procedure. Catheter-based DSA has long been accepted as the gold standard for anatomic imaging in patients with PAD, but arterial duplex ultrasound, CTA, and MRA have emerged as excellent noninvasive alternatives [1, 3, 10, 12, 14]. With the increased utilization of endovascular interventions and broad spectrum of device options, preoperative noninvasive imaging is becoming utilized more frequently for device selection along with planning open bypass and hybrid operations [10]. Noninvasive imaging modalities can potentially reduce the operative risk to the patient by minimizing the contrast volume during the intervention and selecting the safest site for vascular access [20].
Noninvasive Physiologic Tests
Continuous Wave Doppler
The handheld continuous wave Doppler (CWD) is the most basic noninvasive diagnostic tool used in the assessment of arterial flow. The probe emits continuous ultrasound waves and is continuously listening for the reflection of these ultrasound waves to measure the velocity of blood flow. It can be used in a variety of clinical settings from the office, to the emergency department, to the operating room. The audible Doppler signal provides a qualitative assessment of the arterial flow in a vascular bed. In a high-resistance vascular bed, like the lower extremity, a normal Doppler signal is triphasic with forward flow in systole, reversal of flow in early diastole, and forward flow in late diastole (Fig. 15.2). As a vascular bed develops occlusive disease, the Doppler signal becomes biphasic losing the reversal of flow during early diastole and then becomes monophasic as the disease progresses further (Fig. 15.2) [21]. The diagnostic accuracy of CWD can be improved with waveform analysis; this is most commonly used in combination with other noninvasive diagnostic tools that will be discussed later in the chapter [22, 23]. The main limitation to the CWD is its qualitative nature, but its ease of use makes it a very helpful tool in the assessment of patients with CLI.


Fig. 15.2
Continuous Doppler waveforms and segmental limb pressures in a patient with bilateral superficial femoral artery occlusion. The femoral waveform is triphasic. The popliteal waveform is biphasic. The dorsalis pedis (DP) and posterior tibial (PT) waveforms are monophasic
Ankle-Brachial Index
The ankle-brachial index (ABI ) is a simple, noninvasive test that can be used in most clinical settings to establish a diagnosis of PAD [1, 3]. The ABI is a ratio of the ankle systolic blood pressure to the brachial blood pressure. Systolic blood pressure measurements are taken using a CWD ultrasound probe and a manual blood pressure cuff. To measure the ankle pressure, an appropriately sized blood pressure cuff is placed just proximal to the ankle; measurements are recorded for both the posterior tibial (PT) and dorsalis pedis (DP) arteries in each leg. The brachial artery pressure is measured in each arm, and the highest recorded systolic brachial pressure is used to calculate the ABI for both legs. The ratio of highest ankle systolic pressure in each leg to the highest brachial pressure in either arm is then recorded to two decimal points (Fig. 15.3). Normal systolic blood pressures at the ankle are 10–15 mmHg higher than the systolic blood pressure in the brachial artery. A normal range for the ABI is 0.91–1.29 [18, 24]. An ABI of 1.3 and greater is abnormal and considered to be falsely elevated or noncompressible, generally due to heavily calcified tibial vessels. In these patients, the ABI cannot be used as a diagnostic index. An ABI of 0.41–0.9 is consistent with mild to moderate PAD , and an ABI of less than 0.4 is consistent with severe PAD. Furthermore, patients with an ABI of less than 0.4 are more likely to have CLI with either rest pain or tissue loss [3, 18]. Several investigations have been conducted to evaluate the interobserver variability of the ABI. These studies have demonstrated a measurement difference of between 0.05 and 0.08 [25, 26]. These results have been interpreted to indicate a change in ABI of greater than 0.15 to be clinically significant [14, 27]. The ABI is a useful diagnostic tool and can also be used to monitor a patient over time and evaluate the quality of a therapeutic intervention [1].


Fig. 15.3
Ankle-brachial index. From Hiatt WR, Medical Treatment of Peripheral Arterial Disease and Claudication, NEJM, 344: p 21 [18]. Copyright (2001) Massachusetts Medical Society. Reprinted with permission from Massachusetts Medical Society
In addition to being used as a diagnostic tool in PAD, a reduced ABI is also an indicator of the patient’s overall health status [1, 14, 27]. Both a reduced ABI and a noncompressible ABI are predictors of cardiovascular events and premature mortality [6–8]. In a meta-analysis of almost 50,000 patients, an ABI of less than 0.9 was associated with a twofold increase of all-cause mortality , cardiovascular mortality, and major coronary events [6]. This demonstrates the systemic effects of atherosclerotic disease and the need to risk stratify these patients prior to proceeding to revascularization.
The ABI is a useful, quick, and simple test to determine the presence of PAD, but it is not without its limitations . Several studies have demonstrated a significant variability in the method in which the ABI is measured by clinicians [25]. The ABI may also be insensitive to detect disease progression over time [27]. The information obtained from the ABI can only establish the presence of an occlusive lesion but cannot localize the lesion. With slight differences in technique and insensitivity to early disease progression, the test may be less reliable when conducted over long periods of time and between institutions.
The ABI is of limited reliability in patient populations that have a falsely elevated ankle pressure. The result is the illusion of a normal ABI when there is actually significant ischemia. Patients with diabetes, who have a high prevalence of medial arterial calcification , may have a falsely elevated ABI because of severely calcified tibial vessels [28–30]. Elderly patients, patients with very distal tibial lesions, and those with minor stenosis may also have a falsely elevated ABI (Fig. 15.4) [30]. Clinicians must have a high index of suspicion for PAD in these high-risk patient populations. CWD waveform analyses, toe-brachial index, or tissue perfusion examinations can be used in these patients with a noncompressible ABI to evaluate and monitor over time [28, 31, 32]. Despite these limitations , the ABI is a simple noninvasive test that can be used to diagnose PAD, follow patients over time, and evaluate the quality of therapeutic interventions in the right patient population.


Fig. 15.4
Continuous Doppler waveforms in a patient with diabetes. Note the noncompressible ankle pressures with pressure measured >250 mmHg. The common femoral artery waveform is triphasic and the popliteal artery waveform is biphasic. The right leg has monophasic dorsalis pedis (DP) and posterior tibial (PT) waveforms and a toe pressure of 0. The left leg has preserved DP and PT waveforms with a toe pressure of 62. These findings are consistent with severe right tibial occlusive disease, often observed in patients with diabetes
Segmental Limb Pressure
Similar to the ABI, measurement of segmental limb pressures is a noninvasive test that uses blood pressure cuffs and a CWD probe to assess the perfusion of the lower extremities. The arrangement of cuffs can vary by institution by using either two, three, or four cuffs. When using four cuffs, in addition to the brachial artery cuff, the lower extremity cuffs are typically placed on the high thigh, low thigh, calf, and ankle (Fig. 15.5) [33, 34]. Figure 15.2 shows the segmental pressures using a two-cuff method. This study shows a characteristic pressure gradient between the brachial artery pressure and the low thigh pressure along with changes in the CWD waveform from triphasic in the common femoral artery to biphasic in the popliteal artery. The cuff width should be 40 % greater than the diameter of the limb, as inappropriately small cuffs are associated with a falsely elevated pressure [35]. A gradient of over 20 mmHg between cuffs within the same limb is indicative of a hemodynamically significant lesion in the intervening segment (Fig. 15.6) [1]. In contradistinction to the ABI that is not able to localize an occlusive lesion , having segmental cuffs distributed along the length of the leg gives the clinician a good idea of the level of disease especially when using the four-cuff system [34, 36–38]. Though there is some ability to localize the level of disease, this is still an indirect assessment of the vascular tree as there is no direct visualization of the diseased segment. Furthermore, the pressure gradient may be the result of a short occlusion or a long stenosis. As with the ABI, severely calcified arteries can falsely elevate the recorded pressures, making the study invalid [28, 29]. Despite these limitations, this remains a useful tool to establish a diagnosis of PAD and plan further imaging studies or as a means to survey patient before and after an intervention.



Fig. 15.5
Cuff position for segmental limb pressures . (a) Four-cuff technique with two thigh cuffs, a calf cuff, and an ankle cuff. (b) Three-cuff technique with a single wide thigh cuff, a calf cuff, and an ankle cuff. The two-cuff technique (not shown) uses a single thigh cuff and an ankle cuff. (c) Transmetatarsal cuff and great toe cuff

Fig. 15.6
Continuous Doppler waveforms and segmental limb pressures in a patient with bilateral aortoiliac disease as well as infrainguinal occlusive disease. The four-cuff technique is able to identify a pressure gradient across the left thigh suggesting a mid-superficial femoral artery (SFA) stenosis
Pulse Volume Recording
Segmental air plethysmography or pulse volume recording (PVR ) is another noninvasive technique to evaluate patients with PAD. This technique measures the subtle changes in limb volume with each arterial pulsation to obtain qualitative information regarding the nature of arterial flow to the extremity. Similar to segmental limb pressures, cuffs are placed on the thigh, calf, ankle, and forefoot to record the PVR. A brachial cuff is also used to record a PVR in the upper extremity and is used as a comparison for the lower extremity waveforms. The cuffs are inflated to ~60–65 mmHg to record the waveform but not occlude the vessel being measured. The waveforms collected are similar but not identical to those recorded using the CWD for segmental limb pressure measurements. The normal PVR waveform has a quick and sharp upstroke with a rapid decline (Fig. 15.7) [39]. As arterial obstruction progresses, the PVR waveform flattens and widens [39, 40]. The PVR is more reliable in patients who have noncompressible vessels than the ABI [41]. Foot PVR waveforms have been shown to be an indicator of heeling potential of tissue loss and forefoot amputations. The major limitation of the PVR is its qualitative nature. PVR is also abnormal in patients with a diminished cardiac stroke volume, making the test less reliable in this patient population. In this situation, the brachial artery waveform is a helpful comparison to the lower extremity waveforms to determine if the abnormality is the result of PAD or poor cardiac function. Segmental limb pressures have better interobserver reliability than PVR [36, 38]. Currently, PVR is not widely used due to its qualitative nature and the development of arterial duplex ultrasound.


Fig. 15.7
Pulse volume recordings (PVR) from normal limbs with various combinations of peripheral vascular disease. AI aortoiliac, SF superficial femoral, PT popliteal-tibial. From Rutherford RB, Lowenstein DH, Klein MF: Combining segmental systolic pressures and plethysmography to diagnose arterial occlusive disease of the legs. Am J Surg 1979;138:p 216 [39]. Reprinted with permission from Elsevier Limited
Toe Pressure
As mentioned previously, the ABI is not a valid diagnostic test to assess the degree of PAD in certain patient populations [28–30]. In patients that have noncompressible tibial vessels, the digital vessels are typically spared from significant calcification, making the toe systolic blood pressure a more reliable indicator of lower extremity perfusion [42]. Similar to the ABI, the toe-brachial index (TBI) is the ratio of the toe systolic blood pressure to the highest brachial systolic blood pressure. A normal toe systolic blood pressure is 30 mmHg less than that of the systolic ankle pressure making a normal TBI 0.7 or greater. A TBI of less than 0.25 is considered to be severe CLI. The TBI has been shown to be more reliable than the ABI when assessing lower extremity perfusion in diabetic patients who have noncompressible arteries [28]. The absolute toe systolic blood pressure is also a useful clinical indicator. Diabetic patients with an absolute toe pressure of 55 mmHg or higher have been shown to have a better chance of healing their tissue loss [14]. One of the main limitations of measuring the toe pressure is that it cannot be used in patients that have painful tissue loss or inflammation of their digits. Measuring a toe pressure also requires specialized equipment that prevents it from being used in the variety of clinical settings that the ABI can be conducted (Fig. 15.4c). Lastly, the toe pressure will vary corresponding to the systolic pressure. For this reason, the TBI is often a better indicator. Despite these limitations, the TBI is a useful clinical index in patients that have a noncompressible ABI.
Evaluation of Tissue Profusion
Inadequate tissue perfusion is the underlying etiology of CLI and the goal of revascularization is to restore tissue perfusion to the meet the demands of the affected tissue. Several noninvasive techniques, such as transcutaneous oxygen pressure, skin perfusion pressure, and hyperspectral imaging, have been developed to assess the quality of tissue perfusion in patients with CLI [31, 43, 44]. Unfortunately, due to limitations in their techniques, none of these diagnostic studies are widely used; though there are emerging technologies that may prove to be valuable in the evaluation of tissue perfusion.
Transcutaneous Oxygen Pressure
Transcutaneous partial pressure of oxygen (TcPO2) is a noninvasive test that measures the partial pressure of oxygen at the skin surface. Small probes are placed on the foot or leg. A probe placed on the chest is used as a reference to standardize the test for each patient. The probes are constructed of a platinum cathode with a surrounding silver-silver chloride anode ring. Oxygen diffusing to the surface of the skin reacts with the cathode producing an electric current that is then used to measure the partial pressure of oxygen in the target tissue [45]. A normal TcPO2 is above 60 mmHg. A TcPO2 above 30 mmHg has been associated with wound healing in diabetic patients [32, 45]. Some have argued that the TcPO2 may be a better indicator for wound healing and resolution of rest pain compared to a palpable pulse because it is a direct measurement of tissue perfusion at the affected site. A TcPO2 less than 30 mmHg indicates severely reduced perfusion to the target tissue and that healing is less likely to occur [32]. TcPO2 has been shown to be a better indicator of wound healing with hyperbaric oxygen therapy than the TBI or ABI in diabetic patients [46]. Another benefit of the TcPO2 is that heavily calcified vessels do not influence the reliability of the test, and as such it is useful in diabetic patients with a noncompressible ABI [31, 32].
The measured TcPO2 is affected by the amount of arterial flow to the tissue, but it is also impacted by several other factors that may limit its clinical reliability. The measurements are affected by variables such as skin temperature, sympathetic tone, cellulitis, hyperkeratosis, obesity, edema, metabolic activity, age, and probe position [47–49]. Additionally, an abnormal TcPO2 does not necessarily indicate poor perfusion to the tissue; a low TcPO2 can be found when oxygen consumption is increased in the target tissue [45]. The use of TcPO2 has not been shown to be superior to toe pressure measurement in the management of patients with CLI [49]. Because of the number of variables that impact the measurement of TcPO2 , it is not routinely used by most vascular labs.
Skin Perfusion Pressure
Skin perfusion pressure is another noninvasive method of measuring tissue profusion that uses laser Doppler. The laser Doppler probe is placed on the target tissue and detects the motion of cutaneous red blood cells. The waveform generated by the laser Doppler corresponds to arterial flow, but due to the irregular geometry of the cutaneous capillary network, the actual arterial flow cannot be calculated. As laser Doppler detects flow within the skin, it is used in conjunction with a pressure cuff to measure the skin perfusion pressure [45]. A normal skin perfusion pressure is 50–70 mmHg, with skin perfusion pressures of less than 30 mmHg associated with CLI [50, 51]. The variables that impact the reliability of TcPO2 also impact the reliability of laser Doppler. This technique is not widely used among vascular labs because of the inability of laser Doppler to directly measure arterial flow and limitations on calibrating the instrumentation.
Hyperspectral Imaging
Hyperspectral imaging is an emerging noninvasive technique being used in patients with PAD to assess tissue perfusion. The test uses scanning spectroscopy to evaluate the relative cutaneous concentrations of oxyhemoglobin and deoxyhemoglobin. An image is captured with wavelengths of visual light between 500–660 nm; this includes the absorption peaks for oxyhemoglobin and deoxyhemoglobin. These wavelengths only penetrate 1–2 mm below the skin, so only the cutaneous concentrations of these molecules are being measured. A two-dimensional (2D) surface map is then constructed, giving a visual representation of differential oxygenation of the tissue being imaged [43, 52, 53]. Studies evaluating concentrations of deoxyhemoglobin concentrations have shown statistically different concentrations between patients with and without PAD [43]. Additionally there are statistically different concentrations of deoxyhemoglobin between angiosomes with monophasic, biphasic, and triphasic CWD waveforms [43]. This technique has been used to evaluate healing potential of diabetic foot ulcers at 6 months [54]. Although there have been several promising studies in patients that have tissue loss, there have been other studies that show no correlation between the ABI and hyperspectral imaging [52, 53].
Unlike the other noninvasive tests that require placement of probes on the skin or inflation of pressure cuffs to measure pressures, this technique does not require any physical contact with the patient. There is a clear benefit to using this technique for patients that have painful tissue loss in which the other tests may be poorly optimized. Additionally the other tests of tissue profusion can only take measurements at a single point, whereas hyperspectral imaging generates a 2D image of the perfusion to a larger surface area [53]. At this time there have been no large-scale studies involving patients with CLI. As this is an emerging diagnostic tool, more investigation is required before it can be widely implemented.
Noninvasive Anatomic Imaging
Arterial Duplex Ultrasound
Duplex ultrasonography is a noninvasive, inexpensive imaging modality that can provide both physiologic data and anatomic imaging in a variety of clinical settings. Duplex ultrasound equipment is now widely available and inexpensive. With the portability of modern equipment, this imaging modality can be utilized in a variety of clinical settings ranging from the office, to the emergency department, to the operating room. When B-mode imaging is combined with pulsed wave Doppler (PWD), this is commonly referred to as duplex ultrasonography. B-mode or gray-scale imaging produces 2D cross-sectional images of an arterial wall, the arterial lumen, and surrounding tissue. This provides anatomic information about the artery, characteristics of any atherosclerotic plaque within the wall of the artery, or other anatomic pathologies such as aneurysmal disease. As opposed to DSA and MRA, duplex ultrasound can provide useful information about the size and degree of calcification to help identify suitable targets for a distal bypass [12]. PWD gives the ultrasonographer the ability to obtain physiologic measurements within the arterial system [37]. The velocity of blood through an area of stenosis increases relative to the velocity of blood in the artery immediately proximal to the stenosis. Using this principle, the ultrasonographer can identify areas of stenosis in the arterial system by identifying segments with increased velocities. The velocity prior to the stenosis and highest velocity within the stenosis are recorded. A peak systolic velocity (PSV) within a lesion of greater than 200 cm/sec or a ratio of PSV prior to the lesion to the PSV within the lesion of greater than 2.5 is generally consistent with a 50 % or greater stenosis and is considered hemodynamically significant [55]. The combination of these imaging characteristics can give anatomic information about the location, length, and degree of stenosis [23, 56]. Figure 15.8 shows an example of a stenotic lesion in the native superficial femoral artery.


Fig. 15.8
Arterial duplex ultrasonography . (a) A normal triphasic waveform in the common femoral artery and (b) a superficial femoral artery stenosis with an increased peak systolic velocity and biphasic waveform with spectral broadening
Though there are many benefits to duplex ultrasound in the assessment of patients with CLI, there are also some limitations. As with any ultrasound study, the accuracy and reliability of the results are operator dependent and require an experienced technician [23]. The entire leg must be scanned in a continuous systematic manor as to not miss a focal lesion. Ultrasound becomes less reliable the further away the target is from the probe, which can limit the utility in obese patients. This does not pose as much of a problem in the lower leg but can severely limit studies conducted on the aortoiliac segment. Ultrasound does not travel through air, making overlying bowel gas an additional challenge when imaging vessels in the abdomen and pelvis. Sound waves are also reflected off high-density surfaces, making heavily calcified lesion difficult to image and obtain reliable physiologic data. The patient must be positioned in a way for the technologist to evaluate the entire vascular tree. The patient’s pain or wounds may make this challenging and limit the quality of the study. Imaging of pedal vessels may be conducted, but they are easily compressed with the probe and may invalidate the measured values.
The anatomic information obtained from duplex arterial ultrasound can be used to plan revascularization procedures in patients with CLI. This imaging modality has been used to select tibial targets for a distal bypass in place of DSA [11, 20]. Interestingly, one study showed no difference in patency or limb salvage of distal bypasses constructed using either duplex ultrasound versus other angiographic methods to determine the distal target [57]. An arterial duplex ultrasound done prior to an endovascular procedure allows for selective angiography, thereby minimizing the contrast dose during the procedure [20, 58].
Though there has been tremendous advancement in the resolution of modern ultrasound equipment, further advancements are on the horizon to further aid in the diagnostic evaluation of patients with CLI. The addition of intravenous contrast agents can be used to improve the assessment of the lower extremity vasculature [59, 60]. Contrast-enhanced ultrasound of the tibial vessels has been shown to improve the accuracy of arterial duplex ultrasound when compared to DSA [59]. The use of contrast agents may be able to assess the adequacy of muscle perfusion before and after revascularization, which may prove to be a superior means to assess the quality of revascularization compared to other noninvasive tests [61, 62]. The advancement of 3D ultrasound may aid in characterizing the anatomy of short segments of the arterial tree [63]. Robotic ultrasound systems are also being developed in the hopes of automating the study to eliminate some of the variability of the current ultrasound examinations [63, 64]. With these advances, duplex ultrasound will become an even more powerful noninvasive tool in the evaluation of patients with CLI.
Computed Tomography Angiography
Computed tomography angiography (CTA) is a noninvasive imaging modality that has become widely accepted as a primary imaging technique in patients with CLI [9]. The CTA images are produced using an X-ray source and a series of detectors surrounding the patient. The data obtained by this technique is then processed by a computer to produce cross-sectional images of the patient for analysis. Modern multi-detector scanners allow for rapid image acquisition with high anatomic detail of the vascular tree and surrounding soft tissue [65–68]. CTA has a high accuracy to identify, characterize, and measure peripheral arterial occlusive disease with a sensitivity of 91–100 % and specificity of 93–96 % when compared to DSA [65, 69, 70]. The sensitivity of CTA to occlusive lesions is higher than that for stenotic lesions [69]. With a high negative predictive value, a CTA without significant stenosis is clinically important to rule out occlusive arterial disease as the cause of CLI [12]. As with duplex ultrasound, but in contrast to DSA and MRA, CTA gives morphologic characteristics of the arterial wall and surrounding structures that can be used to determine the quality of the artery as a target for distal bypass [12, 69]. This information can also be used to identify other nonatherosclerotic causes of limb ischemia such as peripheral aneurysmal disease [69]. This imaging modality is also faster and more comfortable to patients when compared to MRA that requires a long acquisition time in a confined space and the invasive catheter-based angiography [12]. CTA allows for imaging through previously stented arteries that appear as flow voids when imaged by MRA [71]. Using CTA as a primary imaging modality , though associated with the increased need for additional imaging, is more cost effective than primary DSA and MRA [65, 72].
Modern multi-detector scanners obtain axial images that can then be reformatted into other views that can aid in preoperative planning [69]. These reformats can be 2D multiplanar reformats, curved planar reformats perpendicular to the arterial centerline, or 3D renderings (Fig. 15.9) [68, 70]. Maximum intensity projection is a reformatting method that displays the highest attenuation value, preferentially displaying high-density structures such as arteries with intravenous contrast [65, 70]. Advances in software development have been helpful in increasing the speed at which these reconstructions are produced and may improve the interpretation of the luminal characteristics within heavily calcified arteries. The quality of reformatted images depends on the type of scanner used to generate the axial images, the protocol used to obtain the axial images, and the software used to make the reconstructed images [66, 67]. Reformatted images give the clinician more information about the anatomic characteristics of the vascular tree that can be used to plan revascularization procedures [73].


Fig. 15.9
Computed tomography angiography . (a) Axial image showing a left iliac occlusion (green arrow) and (b) 3D reformat showing left iliac occlusion (green arrow), right superficial femoral artery (SFA) occlusion (red arrow), and left SFA stenosis (yellow arrow). Calcifications are also depicted (white arrows)
CTA has some limitations when imaging the vasculature. Heavily calcified vessels, as seen in diabetic patients, with median calcinosis can make it difficult to determine if small vessels are patent [12]. The way the scanners are designed, the contrast bolus must be followed down the leg as it moves through the arterial tree. Poor timing or high-grade proximal obstruction may impact the quality of the contrast bolus within the distal vessels, limiting the diagnostic accuracy of the study [66]. Additional scans with or without additional contrast may be required to obtain diagnostic images of the tibial vessels. This comes with added radiation exposure and the risk associated with additional intravenous contrast administration. Modern scanners have reduced some of the issues encountered with previous-generation scanners but have not eliminated the problem [66, 67].
Though there are some significant benefits of using CTA as a primary anatomic imaging modality in patients with CLI, there are also several drawbacks . CTA requires intravenous contrast to obtain clinically relevant information when planning a revascularization procedure. Iodinated contrast agents used for CTA are nephrotoxic and must therefore be used cautiously in patients with renal insufficiency [74–77]. Contrast-induced nephropathy (CIN ) is defined as an increase in serum creatinine greater than 25 % or greater than 0.5 mg/dl above baseline within 3 days of contrast administration without another identifiable cause [74, 75]. All patients exposed to iodinated contrast are at risk of developing CIN , but patients with renal insufficiency are at an increased risk [78]. Diabetes is an independent risk factor for CIN, and diabetic patients with renal insufficiency are at the highest risk of developing CIN [76, 79]. The incidence of CIN ranges from 2.9 to 12.1 % and is largely dependent on the degree of renal insufficiency prior to the contrast exposure [76, 78, 79]. Fortunately, only about 1 % of patients will have a persistent change in renal function and only a small fraction of these patients will go on to require hemodialysis [76, 79]. However, the development of CIN is associated with an increased all-cause hospital mortality [75]. To prevent CIN, high-risk patients should be hydrated prior to and after exposure to intravenous contrast [80–83]. The administration of Mucomyst and/or sodium bicarbonate before and after exposure to iodinated contrast has been shown to reduce the risk of CIN in some studies [84, 85]. When used intravenously, as with CTA, there is no difference in the incidence of CIN between iso-osmolar and low-osmolar contrast agents; however, there is a decreased incidence of CIN when iso-osmolar contrast agents are used intra-arterially [86]. Nephrotoxic medications should be held around the time of contrast exposure to also reduce the risk of CIN [74, 75].
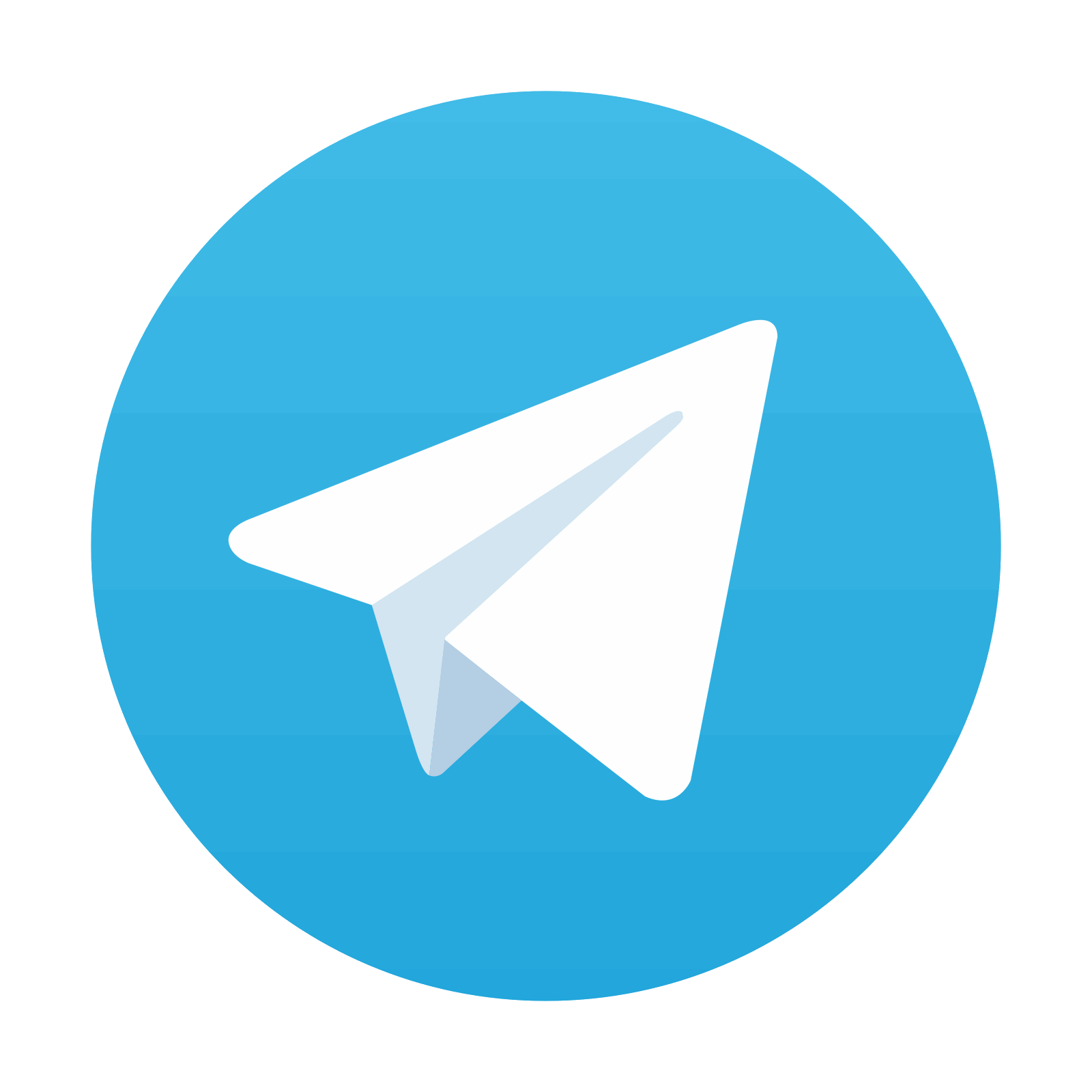
Stay updated, free articles. Join our Telegram channel

Full access? Get Clinical Tree
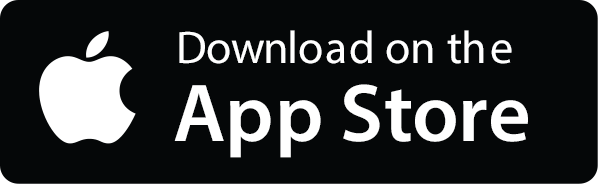
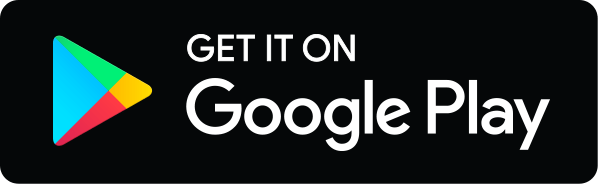