Diagnostic and Interventional Radiology
Trauma imaging may be used to provide rapid and broad surveys when clinical evaluation is likely to be incomplete or unreliable or used to characterize recognized injuries as part of treatment planning. And it may be used to guide observational, operative, and minimally invasive decisions. Therefore, trauma imaging may inform clinical diagnosis and can guide, but not make, management decisions.
Imaging strategies are affected by the proximity of available imaging technology to the resuscitation area, the capabilities of the imaging equipment, the experience and availability of radiology technologists performing emergent imaging procedures, and timely access to expert interpretation and reporting.
The timing of diagnostic imaging should reflect the needs of individual patients and the local system. With some exceptions for image-guided endovascular hemostasis, hemodynamically unstable patients should be resuscitated prior to imaging according to accepted guidelines and recommendations. In order to enhance efficiency, triage priorities for imaging should be based on the acute needs for accurate information that can be used to direct treatment of the patient. Close cooperation and open communication between the emergency physicians, traumatologists, consultants, nurses, imaging technologists, and radiologists are always necessary to optimize any imaging assessment.
One chapter cannot reasonably teach interpretation of diagnostic images. Therefore, a general approach to the role of imaging in the evaluation of selected clinical scenarios is presented, while pointing out the advantages and disadvantages of a given imaging strategy.
INITIAL IMAGING FOR THE ASSESSMENT OF BLUNT TRAUMA
Trauma Series
As part of the secondary survey of victims of blunt trauma, an imaging survey of the chest (supine anteroposterior [AP] chest with 10° of caudal angulation of the central x-ray beam), pelvis (supine AP pelvis), and cervical spine (horizontal-beam, cross-table lateral cervical spine obtained with bilateral arm pull) may be performed, if clinical evaluation alone is deemed insufficient (Fig. 15-1). The goals of these initial imaging studies are to identify life-threatening, but clinically occult, injuries such as an unstable pelvic fracture, hemomediastinum, or instability of the cervical spine.1,2
FIGURE 15-1 Trauma series. This 27-year-old unrestrained left rear seat passenger sustained multiple injuries, superfluous in a high-speed side-impact crash. (A) Anteroposterior (AP) recumbent chest radiograph shows hyperexpanded and hyperlucent left hemithorax with “deep sulcus” sign (short white arrow) and rightward mediastinal shift (double-ended arrow) due to left tension pneumothorax. Short black arrows show multiple displaced rib fractures. Asterisk shows irregularity of left hemidiaphragm, which strongly suggests herniation of abdominal contents through left diaphragmatic laceration. (B) AP pelvis radiograph shows lateral-compression-type pelvic ring disruption consisting of bilateral iliopubic and ischiopubic ramus and left sacral fractures (long arrows) with sacroiliac joint disruptions (short arrow). (C) Cross-table lateral cervical spine radiograph is grossly normal to C5. Therefore, this constitutes a nondiagnostic study. Craniocervical alignment should be assessed and may be easily overlooked. Dens–basion distance (white double-ended arrow) is normally no greater than 12 mm. Posterior axial line represents cephalad extension of posterior cortex of C2 body (and is normally no more than 12 mm posterior or 4 mm anterior to basion) (black double-ended arrow). Anterior atlantodens interval is normally no greater than 3 mm in adults and 5 mm in children (8 years and younger). Laminar point of C2 (laminar points are most anterior extent of neural canal margin of lamina) should be within 1.5 mm of line connecting laminar points of C1 and C3. (D) Coronal image of right upper quadrant from focused abdominal sonography for trauma (FAST) shows free intraperitoneal fluid in anterior subhepatic (Morison’s) space (arrows), compatible with hemoperitoneum.
The trauma resuscitation “ABCD” strategy may be extended to this “trauma series.” Verification of the integrity of the airway (and other tubes and lines) should be specifically made on the x-rays of both the chest and lateral cervical spine. Radiographic pulmonary opacities associated with hypoxemia include pulmonary contusions, aspiration pneumonitis, and atelectasis (including collapse due to aspirated dental or foreign debris). Tension pneumothorax and hemothorax are typically detected on clinical examination, while clinically occult pneumothoraces or hemothoraces are commonly shown by chest x-rays as a “deep sulcus” sign and generalized hemithoracic opacity, respectively. Other injuries, such as rupture of the hemidiaphragm, flail chest, pneumopericardium, and pneumomediastinum and hemomediastinum, are often diagnosed or suggested by initial conventional x-ray findings. Hemodynamic instability may be due to extraperitoneal hemorrhage from disruption of the pelvic ring. Biomechanically unstable disruptions of the pelvic ring are almost always shown on AP radiographs and may be associated with injuries to the bladder and urethra. In addition, pelvic x-rays may show hip dislocations and fractures of the acetabulum and proximal femur.
A technically adequate (C1–T1) lateral x-ray of the cervical spine provides a reasonable “screening” study to identify most unstable cervical injuries. It may provide important information regarding the best technique for airway control and may confirm that spinal shock from a vertebral fracture is the cause of unexplained hypotension.
Focused Abdominal Sonography for Trauma (FAST)
FAST is performed as part of the secondary survey in victims of torso trauma (see Chapter 16). It is a temporally (2–5 minutes) and anatomically limited real-time sonographic examination whose core components include a direct sonographic search for free fluid in the pericardial sac, both upper abdominal quadrants, and the intraperitoneal recesses adjacent to the urinary bladder. Scanning is optimally performed in two orthogonal planes (e.g., longitudinal and transverse), and intraparenchymal and retroperitoneal injuries are generally not sought but are sometimes seen. FAST may be extended to detect a hemothorax and pneumothorax. Commercially available, portable handheld real-time imaging devices are technically adequate to perform FAST.
FAST is uniformly accurate for the detection of intraperitoneal fluid with moderately large volumes >400 cm3 (at smaller volumes, accuracy varies with user experience).3–5 Unfortunately, isolated hepatosplenic injuries with minimal or no hemoperitoneum represent as many as one third of solid organ injuries.6,7 Fortunately, small isolated intraparenchymal lesions with less than 250 mL of intraperitoneal blood rarely require endovascular or surgical intervention (liver <1%, spleen <5%).8 False-positive interpretation of FAST images can result from improper machine settings (gain), sonolucent perinephric fat (which is rarely sonolucent in both axial and coronal scanning planes), fluid-filled loops of bowel, bladder, various types of fluid-filled intra-abdominal cysts, and physiological or nontraumatic free fluid (ascites). FAST is widely available, inexpensive, and noninvasive, uses no ionizing radiation, and can be repeated serially. In the setting of patients admitted with severe hemodynamic compromise or obvious hemorrhagic shock, FAST can establish the abdomen as a source of hemorrhage within a few seconds.
The FAST does, however, require operator training and experience for reliable performance and interpretation, and these can limit its value. In addition, FAST is not very valuable in patients with pelvic fractures as a hemoperitoneum may be present in the absence of visceral injury. Another important limitation of FAST is that isolated injuries to the bowel and retroperitoneal injuries are not reliably detected. While a systematic review of the literature would not support the use of FAST as a replacement for DPL and computed tomography (CT) in blunt abdominal trauma, many trained surgeon-sonographers use it on a daily basis with great accuracy.9
Hemodynamically stable patients who have suffered blunt trauma with a clinical presentation suspicious for injury to the bowel (e.g., lap belt sign and associated Chance or flexion-distraction injury to the thoracolumbar spine) or hematuria (e.g., gross hematuria in all age groups, microscopic hematuria defined as >50 red blood cell count per high-power field in individuals 16 years old and younger, or in those older with at least one documented episode of hypotension) should undergo intravenous contrast-enhanced CT of the abdomen and pelvis.
COMPREHENSIVE IMAGING FOR BLUNT POLYTRAUMA
Victims of blunt multiple trauma often receive portable imaging in the trauma suite and are then triaged based on their hemodynamic status to the operating room, intensive care unit, or angiography suite for ongoing resuscitation or remain in the trauma center for completion of secondary and tertiary surveys, including initial imaging. Typically, this consists of CT for clinically appropriate imaging (e.g., head, neck, chest, abdomen, and pelvis) for the most severely injured, but hemodynamically stable patients. Subsequently, conventional x-rays of the extremities and spine may be obtained. Less severely injured individuals may take a slightly different route with conventional x-rays preceding CT and directed at abnormalities found by clinical examination or prior imaging.
There are some who advocate the “pan scan,” also known as the “head to toe” CT, as a method of detecting many injuries. This paradigm advocates utilization of high-resolution thin collimation image acquisition to include CT scanning of the head, face, soft tissue of the neck, the chest, the abdomen, and pelvis, including the acetabulum and the spine. While it does provide excellent coverage, it exposes patients to much radiation and is not recommended as a general rule. On the other hand, the “pan scan” has value in a high-volume facility with considerable imaging resources and the need for rapid screening.
Multidetector Computed Tomography (MDCT)
MDCT scanners have changed the way CT is used in imaging trauma.10,11 MDCT scanners, with 16 or more detectors, can provide nearly equivalent resolution in any imaging plane with progressively shorter scan times. This greatly improves the quality of both two-dimensional (multiplanar) and three-dimensional reformations. The rapidity of multidetector helical scanning and enormously improved designs of x-ray tubes allow for single-session scanning from cranial vertex to pelvic ischia in less than 90 seconds.12,13 This includes two- and three-dimensional reformations of the thoracic and abdominal aorta, maxillofacial skeleton, cervical and thoracolumbar spines, and pelvis and acetabulae.
With appropriate anticipation and proscription, scanning parameters allow raw axial data to be reconstructed using different thicknesses (e.g., 5-mm-thick slices for abdominal viscera and 2.5-mm-thick slices for CT aortography). Thus, individual thin-section imaging of parts of the body in addition to the CT survey is superfluous, increases ionizing radiation unnecessarily, and increases time-out of the critical care area. This previous standard of detailed specialized imaging of the face and spine should be abandoned.
Computed Tomography of the Head
Axial noncontrast CT scanning remains the reference standard in patients with acute craniocerebral trauma, guiding initial decisions regarding the clinical management.14,15 Indications for CT of the cranium include the following: (1) objective evidence of closed injury to the brain, including decreased level of consciousness; (2) cranial or facial deformity; (3) hemotympanum; or (4) evidence for leakage of cerebrospinal fluid (Figs. 15-2 to 15-4). Clinical criteria reliably predict significant intracranial injury (ICI) and help determine which patients will require CT scanning of the head. In children,17 significant ICI is extremely unlikely in any child who does not exhibit at least one of the following high-risk criteria: (1) evidence of significant skull fracture; (2) altered level of alertness; (3) neurological deficit; (4) persisting vomiting; (5) scalp hematoma; (6) abnormal behavior; or (7) coagulopathy.16 More generically, minor trauma to the head may lead to surgically important injuries to the brain, and liberal utilization of CT is appropriate among individuals who have sustained “high-risk” mechanisms. These clinical criteria are not as reliable in the elderly patient.17 Single-photon emission CT (SPECT) may be helpful in initial diagnostic evaluation of patients with mild traumatic brain injury (MTBI), particularly for those with normal CT findings and associated post-traumatic amnesia (PTA), postconcussion syndrome (PCS), and loss of consciousness.18
FIGURE 15-2 Epidural hematoma. Helmetless bicyclist in crash. (A) Lateral scout scanogram shows linear lucency compatible with fracture (arrows). (B) Axial computed tomography (CT) displayed at bone window shows minimally displaced right parietal skull fracture with overlying subgaleal hematoma. Fracture is marked by white arrow and barely visible hyperintensity of extra-axial blood collection by arrowheads. Bone windows are inadequate to identify intracranial hemorrhages. (C) Axial CT at brain windows shows epidural hematoma (arrowheads), associated midline shift (short arrow), and subgaleal hematoma (asterisk). (D) Axial CT at level of the suprasellar cistern, brain windows, shows epidural hematoma (black asterisk), asymmetric widening of perimesencephalic cistern (arrow), which suggests impending uncal herniation. (Reproduced with permission from D. K. Hallam.)
FIGURE 15-3 Blunt head injury: Diffuse axonal injury. This 48-year-old helmeted female motorcyclist sustained diffuse axonal injury. (A) Axial computed tomography (CT) at level of lateral ventricles demonstrates intraventricular hemorrhage (asterisk), “tear” hemorrhage in posterior limb of internal capsule (white arrow). Black arrow denotes splenium of corpus callosum, which appeared normal by CT. (B) Axial magnetic resonance imaging (MRI) using FLAIR shows clot in right lateral ventricle as extended region of low signal (asterisk), edema associated with tear hemorrhage (white arrow), and splenium of corpus callosum (black arrow) to better advantage. (C) MRI gradient recalled echo (GRE) sequence shows low signal intensity of magnetic susceptibility due to hemorrhage in region of posterior horn of internal capsule (white arrow) and in right lateral ventricle (asterisk). Splenium of corpus callosum has region of mildly increased signal intensity compatible with edema (black arrow). In general, the more central findings of diffuse axonal injury, the greater the degree of neurological disability. (Reproduced with permission from W. A. Cohen.)
FIGURE 15-4 Patterns of herniation. (A). Axial computed tomography (CT) at level of suprasellar cistern shows extensive subarachnoid hemorrhage extending from lateral aspect of suprasellar cistern (1), into the sylvian fissure (2), circumferentially about brainstem and perimesencephalic cistern (3), along tentorium (4), and interpedunculate cistern of mesencephalon (5). Entrapment of lateral ventricles is shown as dilatation of temporal horns (white arrows). Brainstem appears relatively lucent and heart shaped, with pointed inferior portion of heart due to “beaking” of mesencephalon due to upward herniation. (B) Subfalcine shift. Axial CT, at brain windows, at level of lateral ventricles shows marked rightward subfalcine shift (black arrow), quantified as distance from third ventricle to line connecting anterior and posterior portions of sagittal sinus (which tend not to shift due to their fixed relation to calvarium). Note extensive hemorrhage in left frontal parietal region extending into left ventricle. (Reproduced with permission from W. A. Cohen.) (C) Uncal herniation. Axial CT at level of middle cranial fossa shows large left temporal hematoma (black asterisk) associated with left uncal herniation (small white arrows). Enlargement of right temporal horn (large white arrow) is compatible with obstruction to cisternal system. (Reproduced with permission from A. B. Baxter.) (D) Combined upward and downward herniation. This is a 38-year-old with posterior leukoencephalopathy due to hypertension. Axial CT at level of suprasellar cistern shows enlargement of lateral ventricles and right temporal horn (long white arrows). Suprasellar cistern is poorly seen. Perimesencephalic cisterns are absent and posterior aspect is beaked, compatible with superior herniation from posterior fossa. Upward herniation is also shown by the cerebellar vermis filling the subtentorial cisternal space (multiple small arrows). (Reproduced with permission from W. A. Cohen.) (E) Same patient as in (D). Sagittal T1-weighted magnetic resonance imaging shows tonsillar herniation through the foramen magnum (arrowheads), as well as superior herniation of cerebellum and mesencephalon at level of tentorium (white arrow).
Images are reconstructed using both bone and soft tissue algorithms and viewed at bone windows and two different soft tissue windows (“brain” and “blood”).
CT scanning is highly sensitive for the detection of extra-and intra-axial hemorrhage and mass effect, as well as for soft tissue injuries to the globe and paranasal sinuses. In patients with diffuse axonal injury, however, CT may even be normal and discordant between the severity of the clinical brain injury and radiographic findings. On a cautionary note, skull fractures aligned in the plane of scanning may be subtle, and review of the scout views used to plan the CT head study may alert the clinician to such fractures. Except for medicolegal imaging of nonaccidental trauma (child abuse), conventional x-rays of the skull are usually not necessary.19,20
Computed Tomography of the Maxillofacial Skeleton
Indications for specific facial CT scans include the following: (1) deformity or instability of the maxillofacial structures found by physical examination; (2) deformity, opacification, or fracture of the periorbital or paranasal sinus shown on head CT; and (3) clinical evidence for leakage of cerebrospinal fluid (Fig. 15-5). The mnemonic “LIPS-N” (lip lacerations, intraoral lacerations, periorbital contusions, subconjunctival hemorrhage, or nasal lacerations) provides a helpful tool during clinical examination of trauma patients, also, given the high association between any of LIPS-N lesions and facial fractures.21 Absence of opacification of a paranasal or periorbital sinus on CT generally excludes surgically important injury to the maxillofacial skeleton.22
FIGURE 15-5 Facial fracture: zygomaticomaxillary complex (ZMC) fracture with associated nasoethmoidal orbital complex fracture. This 54-year-old male sustained a blow to the face in a motorcycle crash. (A) Anteroposterior (AP) scanogram shows loss of symmetry to orbital volumes, with elliptoid enlargement of right orbit. Associated indistinctness of orbital floor and lateral maxillary sinus walls is also present. Opacification of right maxillar sinus is shown. (B) Axial computed tomography (CT) image at the level of zygomatic arches shows depression and overriding apposition of impacted zygomatic arch fracture (lateral white arrow), posterolateral maxillary sinus wall disruption (posterior arrow), and segmental comminuted fracture of anterior maxillary wall (anterior arrow). Medial to this anterior arrow is the base of the nasofrontal process of the maxilla with a fractured nasolacrimal duct just posterior to it. Internal rotation of the nasofrontal process of the maxiilla and associated fracture of the nasolacrimal duct are not portions of ZMC fracture and represent an associated nasoethmoidal orbital complex fracture. (C) Coronal CT reformations shows separation of right frontozygomatic suture (lateral and superior arrow), disruption of orbital floor (white arrow projected over orbit), and lateral maxillary wall (inferior white arrow). (D) Sagittal CT reformation shows associated vertical fracture of right ascending ramus of mandible, with anterior subluxation at temporomandibular joint (white and black arrows, respectively). (E) Three-dimensional CT reformation gives an overview of complex fracture of zygomaticomaxillary region and right mandibular fracture. It is important to note that spatial resolution is lost with three-dimensional reformations, although spatial comprehension is often improved. (F) Three-dimensional CT reformation shows depression of right zygomatic arch and loss of projection of the right zygoma (flat cheek).
Images need be acquired in only one plane, usually the axial, with reformations in the orthogonal plane, or as reconstruction from a CT of the cranium obtained from an appropriately prescribed study (i.e., 1.00- to 1.25-mm slice thickness). Spiral CT, and especially MDCT, can be reformatted into 2D reformations in the orthogonal planes (e.g., coronal, sagittal, oblique sagittal) and 3D reformations, using 1.0- to 1.25-mm thick sections in the axial plane, without loss of image quality and accuracy, stress on the neck, and further radiation.
Primary axial images are obtained using 1.0- to 1.25-mm slice thickness from above the frontal sinus to the hard palate or maxillary alveolus. These are commonly extended to include the mandible to aid surgical diagnosis, especially in children, as fractures of the condyle of the mandible are the most commonly missed maxillofacial fractures in this age group.23–25 Reformations in the coronal and sagittal planes should be perfectly orthogonal to the axial images. Reformations in the sagittal plane may be performed either relative to the coronal plane or as sagittal oblique reformations parallel to the optic nerve, if evaluating for blowout fractures of the orbital floor.26
While CT is highly accurate at detecting and characterizing surgically important injuries, it does not show the magnitude of osseous fragmentation found at surgery in complex fractures. Orbital and maxillary fractures should heighten suspicion of more complex fractures. Although its incremental diagnostic value is small, 3D-CT reformation appears to be the best imaging method for the global portrayal of complex maxillofacial injury patterns such as Le Fort–type fractures. This technique provides valuable information on spatial relationships and is particularly useful in planning operative treatment.27–30
On the other hand, axial and 2D reformations best portray defects in soft tissue, interposition of osseous fragments, and herniations of soft tissue (e.g., orbital floor blowout fractures) and are best for quantifying size of fractures.26,31
Maxillofacial CT delivers a significant radiation dose to the orbits and to the soft tissues of the neck (e.g., thyroid). This is an important consideration when imaging children.32
Imaging for Soft Tissue Injuries of the Neck
Clinical findings for blunt injury to the aerodigestive tract include subcutaneous crepitus, hemoptysis, hoarseness, neck pain, and abrasions or hematomas (e.g., from the shoulder harness of a three-point restraint; Figs. 15-6 and 15-7). In the absence of a pneumothorax, x-ray findings that suggest injury to the aerodigestive tract include parapharyngeal or precervical emphysema, soft tissue swelling, or fracture of the larynx or hyoid bone on the lateral image of the cervical spine. X-rays of the neck for the soft tissues, however, are superfluous as the image resolution is inferior and the imaging findings are not substantive. CT is the standard of care for imaging this area.
FIGURE 15-6 Soft tissue neck injury. This is a 15-year-old male motorcyclist with “clothesline injury,” causing tracheal and esophageal transection. (A) Lateral view of cervical spine shows extensive subcutaneous emphysema (white arrows). Endotracheal cuff balloon is abnormally large (diameter >3 cm), compatible with soft tissue injury, abnormal airway, or tracheomalacia. In general, the presence of precervical or parapharyngeal emphysema in neck without pneumothorax should raise question of airway or digestive tract injury. (B) Axial computed tomography (CT), lung windows. Cervical tracheal disruption is shown between two short white arrows at posterior and left side of trachea. Extensive parapharyngeal and precervical emphysema is present (long arrows). (C) A different 27-year-old man sustained thyroid cartilage fracture in strangulation. Axial CT at level of thyroid cartilage, soft tissue windows. White arrow points to paramedian thyroid cartilage fracture. Thyroid cartilage fractures typically occur within 2 or 3 mm of anterior junction of the lamina of the thyroid cartilage and are thought to be due to wishbone-like spreading of thyroid cartilage as it is driven against the vertebrae. Overriding apposition of fracture fragments shortens the vocal cord on the affected side, causing hoarseness and lower voice.
FIGURE 15-7 Esophageal injuries. (A) Gunshot wound in Zone II of the neck of a teenager. Barium extravasation directly enters the airway from a high laryngoesophageal fistula (circled). (B) Gunshot wound traversing Zone I into the right chest. Barium swallow shows leak on both the left side of the cervical esophagus (curved arrows) and the right thoracic esophagus (straight arrow). (C) A 27-year-old male sustained a low transverse mediastinal gunshot wound entering left, exiting right. Gastrografin esophagram shows leak into the right chest (straight right arrow) and into the peritoneal cavity (curved black arrow).
Noncontrast MDCT of the neck to visualize the larynx requires thin-section axial imaging from the hyoid bone to the sternal notch. Images are reconstructed using both bone and standard (soft tissue) algorithms and portrayed at bone and soft tissue windows, respectively.
CT is often most helpful in the evaluation of laryngotracheal injuries when deformed anatomy or extensive hemorrhage makes direct or indirect endoscopy more difficult. A careful search for open injuries (e.g., air adjacent to cartilaginous fractures) guides the need for intervention for debridement and mucosal closure. CT can assist in the grading of laryngotracheal injuries, but tends to understage compared to endoscopy or open exploration. The search should begin in the region of the valleculae and extend anteriorly along the larynx and trachea. The most common injuries are those to the thyroid cartilage. Fractures of the thyroid cartilage typically occur within 2–3 mm of the anterior crest of the two lateral laminae. Comminuted fractures of the laminae generally result from higher energy and direct impact injuries to the larynx, and are more commonly associated with thyrocricoid dislocation. Also, CT can demonstrate subluxations and dislocations of the arytenoid cartilage. Most tracheal disruptions are in the membranous portion of the trachea and will heal with conservative therapy. Soft tissue emphysema immediately adjacent to the trachea suggests an injury in this location. A search for a fracture of a tracheal ring is often fruitless unless the fracture is displaced. Coronal reformations through the trachea may show separation (vertical diastasis between tracheal rings) of the trachea compatible with more serious grades of injury.
A blunt injury to the esophagus is uncommon. It generally occurs in the proximal third, especially in the cervical esophagus. On the other hand, a penetrating injury to the esophagus is more common and may involve any portion of the esophagus.
A definitive diagnosis requires contrast esophagography or endoscopy or both, because neither is sufficiently accurate to exclude injuries, especially in patients who are intubated or unconscious. Barium sulfate is the contrast agent of choice for suspected esophageal injuries at or above the carina where aspiration is a concern. Water-soluble contrast media are less accurate in identifying leakage and are inflammatory when aspirated into the airway. Gastrografin should be avoided because it is hypertonic and results in pulmonary edema if aspirated into the airway. When there is concern of an injury to the distal esophagus or stomach, barium should be avoided because it can result in granulomas and peritonitis. Therefore, water-soluble contrast media should be used first when peritoneal contamination is a concern.
Rapid filming is necessary. In all but the most cooperative patients, the author injects thin barium through a feeding tube or a nasogastric tube to obtain full distention of the esophagus. The patient is placed in an oblique projection with a feeding tube or nasogastric tube positioned at the region of penetration. To obtain full distention, 50 mL contrast agent is rapidly injected, during which time fluoroscopic cineradiographs are obtained at 2–3 frames/s.
IMAGING FOR VASCULAR INJURIES OF THE NECK
Blunt carotid and vertebral injury (BCVI) (Fig. 15-8A) is now known to be much more common than previously appreciated. Vascular imaging of the neck is warranted in injury patterns associated with a high risk of blunt injury to the carotid and vertebral arteries, including the following: fracture of the cervical spine (especially involving C1–C3, involving the transverse foramina or extension into foramen magnum), neurological deficits not explained by findings at brain imaging, new-onset Horner’s syndrome, high-energy facial fractures (Le Fort II or III), fracture of the skull base involving the foramen lacerum, or soft tissue injury in the neck (neck belt sign and “hangings” sufficient to cause central anoxia).33,34 Catheter angiography is indicated emergently in patients with an expanding cervical hematoma, active extravasation from nose, mouth, or ears, or a cervical bruit in individuals younger than 50 years old.35 Angiography is the primary diagnostic tool in symptomatic BCVI because it allows for rapid utilization of endovascular therapy for bleeding and identifies patients at risk for embolic stroke.33,36 The sensitivity of duplex Doppler US is inadequately low (38.5%) for directly depicting BCVI. Therefore, CT angiography using MDCT, with a sensitivity of 90–100%, is the imaging test of choice in patients with less obvious signs of vascular injury because it allows rapid acquisition of a vascular assessment without delaying a rapid trauma workup.37–39
FIGURE 15-8 Blunt carotid and vertebral injury. (A) This 40-year-old lawyer complained of severe chronic unrelenting headaches. Prior history included a whiplash injury as a driver. Vertebral arteriogram shows fusiform dilatation of the vertebral artery at the level of the skull base (circled in white). This likely represents long-term effect of BCVI. A 2.8 French microcatheter was negotiated through the tortuous vertebral artery. Then coils were placed proximal and distal to the fusiform aneurysm with relief of headaches. (B) A 22-year-old man sustained a gunshot wound of Zone III of the neck and face. He was exsanguinating. Internal carotid arteriogram showed transection of the internal carotid artery high near the skull base and well above the angle of the mandible (arrow) with active oral hemorrhage (circled). The internal carotid artery was embolized with coils and patient made full neurological recovery.
CT arteriography of the neck is obtained following a timing bolus performed at the C6 level using 20 mL of contrast at 3 mL and a slice thickness of 5 mm. Based on the timing bolus, the CT arteriogram of the neck is acquired. Reformations, including those that are three-dimensional, performed as multiplanar volume reformations (MPVR) with maximum-intensity projections, are obtained.
PENETRATING VASCULAR INJURIES OF THE NECK
The imaging algorithm used to evaluate penetrating neck trauma is very complex. It depends on the mechanism of injury, the locations of entry and exit wounds, the hemodynamic and neurological assessment, and the likelihood of injury. Gunshot wounds may cause a blast injury involving intima, and shards and fragments of bone and metal can cause artifacts in the area that make interpreting a CT difficult. Catheter-based arteriography is advised for such wounds. When the likelihood of asymptomatic injury is low, CT arteriography may play a role in management of such patients.
Location of penetration is a key driver of the imaging workup for a suspected vascular injury. Zone III, above the angle of the mandible, is difficult to assess clinically and to explore operatively. There are extensive vessels at risk, including the internal carotid artery, the external carotid artery and branches, the vertebral artery, and the accompanying veins. Thus, imaging plays a vital role in these patients. Selective internal and external carotid arteriography, vertebral arteriography, and four-vessel intracranial arteriography all play important roles in detecting and evaluating such injuries. Indeed, arteriography is so valuable for the evaluation and treatment of bleeding in this zone that aggressive steps to resuscitate the unstable patient and control bleeding by packing are warranted to allow angiography to proceed. Most vascular injuries in Zone III are best managed by the interventional radiology service (Fig. 15-8B).
Zone II, between the inferior margin of the mandible and the manubrium/clavicles, is evaluated easily by a physical examination. If the common carotid artery or the internal jugular vein require repair, operative exposure is relatively simple and unobstructed. Thus, little imaging is necessary after penetration when “hard” signs of a vascular injury (see Chapter 41) are present. Treatment of asymptomatic patients is more controversial.
In Zone I, the area inferior to the manubrium/clavicles, the brachiocephalic vessels, trachea, or esophagus may be injured, and rapid exsanguination may occur. As a result, symptomatic patients may undergo urgent exploration without imaging.
When patients are stable or asymptomatic, immediate angiography has great value in excluding injury, in detecting vascular injuries that can be treated nonoperatively by embolization or insertion of a stent or stent graft, and in facilitating surgical exploration. CT angiography can be considered as a screening test because it often determines trajectory and the presence of hemorrhage. Penetrating injuries to Zones I and III of the neck are best evaluated with catheter-based imaging in the hemodynamically stable patient. CT of the neck and chest may allow analysis to determine whether penetration is in proximity to the great vessels or even whether vessels are injured. Unfortunately, artifacts are often present when bullets are adjacent to the area or the contrast medium is administered from the arm. As intimal injuries to the great vessels may be life-threatening, these artifacts limit the usefulness of CTA in Zone I and, possibly, in Zone III.
Computed Tomography of the Cervical Spine
In adults and older children (≥10 years old), validated clinical prediction rules (i.e., National Emergency X-Radiography Utilization Study Group [NEXUS] and the Canadian Cervical Spine Rule) can reliably determine those trauma victims who need imaging of the cervical spine. Another clinical prediction rule helps determine which imaging modality is most cost-effective. Specifically, Blackmore et al.40 developed a clinical prediction rule to determine pre-imaging risk of fracture in select patients about to undergo a helical CT to survey the cervical spine for fracture and soft tissue injuries (Figs. 15-9 to 15-12). For the application of this clinical prediction rule, it is assumed that the patient will already be undergoing CT of the cranium. Any one of three mechanisms of injury or any one of three clinical findings puts the patient at a pretest risk of greater than 5% of harboring an injury in the cervical spine. High-risk mechanisms of injury include the following: a high-speed motor vehicle crash >35 mph or >50 km/h combined impact, motor vehicle crash with a death at the scene, or a fall from a height of >10 ft or >3 m. Clinical parameters suggesting an increased risk for injury to the cervical spine that are associated with a high-risk mechanism include the following: a significant closed injury to the brain (or intracranial hemorrhage shown by CT of the cranium), acute neurological deficits referable to the cervical spine (acute myelopathy or radiculopathy), or either pelvic fracture or multiple extremity fractures. Hanson et al.41 validated the clinical prediction rule prospectively and showed its application by separating victims of blunt trauma into a high-risk group (12% prevalence of acute injury to the cervical spine) and a low-risk group (0.2% prevalence of injury to the cervical spine). In infants (<1-year old) and younger children (<9 years old) no validated rule exists. In general, patients with greater severities of injury have an elevated risk of injury to the cervical spine. Conventional x-rays will depict essentially all clinically important fractures and dislocations in patients aged 9 years and younger. CT is not indicated in younger children and infants to screen the cervical spine, nor in search for other occult injuries causing neurological deficits.42,43 CT should be reserved as a staging/treatment planning procedure among patients with a known bony abnormality.
FIGURE 15-9 Upper cervical spine, coronal reformations from computed tomography (CT). (A) Coronal reformation of craniocervical junction CT shows pathological widening of right lateral atlantoaxial interval (double-ended arrow), widening of left occipital condyle–C1 lateral mass interval, and bony flake due to left type 1 dens fracture (long arrow). These findings are compatible with atlanto-occipital dissociation. (B) Coronal reformation of the craniocervical junction shows transverse type 2 dens fracture (white arrow). Black arrow marks tubercle on C1 for the insertion of transverse atlantal ligament. Asterisk marks osteophyte at superior margin of C1 portion of anterior atlantodens articulation, a common finding in older patients. (C) Coronal reformations in a patient who sustained high-energy trauma show type 3 dens fracture (white arrows), which is minimally distracted. (D) Coronal reformations from survey CT of cervical spine focused at craniocervical junction. Black arrow demonstrates displaced type 2 dens fracture. White arrows show a right lateral mass fracture of C1. Intra-articular (lateral mass) fractures of C1 less often have involvement of transverse atlantal ligament unlike Jefferson burst fractures, which are more commonly extra-articular or minimally intra-articular.
FIGURE 15-10 Upper cervical spine, sagittal reformations from computed tomography (CT). (A) Left parasagittal CT reformation. This 3-year-old was run over by a trailer. The upper, long, white arrow shows a vertically and coronally oriented fracture of occipital condyle. The two lower arrows show superior articular surface of C1 lateral mass, with anterior subluxation of head. (B) Midline sagittal reformation shows hyperextension teardrop fracture at C2 (white arrows) in a 20-year-old man. (C) Midline sagittal CT reformation shows a combination of anterior subluxation of C2 relative to C3 (white arrow), as well as posterior displacement of lamina of C2 relative to C1–C3 line as manifestation of hyperextension hangman’s fracture of C2. (D) Sagittal mid-plane CT reformation shows diastasis of type 2 dens fracture (double-ended arrow). Distraction of >3 mm is commonly associated with disruption of anterior and posterior longitudinal ligaments.
FIGURE 15-11 Lower cervical spine, sagittal reformations from computed tomography (CT). (A) This 84-year-old woman sustained C5 hyperextension injury in a fall. Lateral cervical spine shows extensive spondylosis but no obvious fracture to vertebral bodies. However, white Xs mark laminar points and show definite retrolisthesis of C5 on C6. Careful attention to laminae, particularly in the elderly, is good practice in detecting translational abnormalities. (B) Same patient as in (A). Sagittal reformation in midline from CT shows complex fracture of C6 with marked neurocanal narrowing at C5 body–C6 laminar level. Disruption of flowing osteophytosis of anterior longitudinal ligament (ALL), seen in diffuse idiopathic skeletal hyperostosis, should be presumed grossly unstable. Extent of anterior, appositional osteophytes is marked by “W” and is fairly typical of diffuse idiopathic skeletal hyperostosis. (C) Midline sagittal CT reformation shows flexion teardrop fracture of C6 in a 79-year-old man. Mechanistic classification of these fractures is based on relation of height relative to width of avulsed fragment (H and W). Teardrop fracture (black arrow) separates anterior inferior corner of C6 vertebral body from its corpus. In this case, H is greater than W, compatible with hyperflexion injury. In lower cervical spine, hyperextension injuries tend to have greater width than height of their corner fragments and can be from anteroinferior or anterosuperior corner. Note this relation is different at C2, where hyperextension fragments typically have greater height than width, due to peculiar shape of anteroinferior corner of C2. (D) Parasagittal CT reformation shows oblique corner fracture through the C7 lateral mass, with anterior and inferior displacement of C6 lateral mass relative to inferior cervical spine (white arrow).
FIGURE 15-12 Multimodality correlations: cervical spine. A patient with C6-7 fracture dislocation underwent pre-reduction lateral conventional radiograph (A), post-reduction sagittal reformation (B) from axial computed tomography (CT), and MRI of the spine and cord (C) and (D). (A) White Xs mark laminar points and connecting them shows disruption of spinolaminar line at C6–C7. Arrow points to fractures of inferior articular process of C6. Sagittal CT reformation (B) shows improved alignment of vertebral bodies but persistent encroachment of neural canal from bony fragments from body and posterior elements (arrow). (C and D) Sagittal magnetic resonance imaging performed using short tau inversion recovery (STIR) and gradient recalled echo (GRE) sequences following reduction of C6–C7 fracture dislocation. Single asterisk shows precervical edema and double asterisk edema in posterior spinal musculature. Long white arrow shows a region of cord swelling with heterogeneous signal, suggesting cord transection at C6 level. Short arrow shows abnormal signal within C6–C7 disk space. GRE sequences (D) show decreased signal at C6 level within the center of the cord that is compatible with hemorrhage (white square), which portends poorer neurological prognosis than edema alone.
Axial slices of thickness of 1.25–3.0 mm are obtained from the skull base through the T4 vertebral body. Images are typically reconstructed at half the slice thickness interval for creation of parasagittal and coronal reformations. Reformations are typically contiguous 2.5- to 3.0-mm thick images and can be reconstructed using either a bone or soft tissue algorithm, but evaluated using bone windows. Generally, the information gathered from these reformations is sufficient and makes plain x-rays unnecessary.
Since helical CT produces large numbers of axial images, the review of the examination is substantially facilitated by use of picture archiving and communication systems (PACS) workstations and the so-called scroll functions. In addition, use of cross-referencing tools on the PACS workstation facilitates identification of specific vertebral levels.
In many ways, the coronal reformations may be viewed using the same approach as standard frontal x-rays such as the open mouth views of the craniocervical junction and the AP view of the cervical spine, while viewing of the sagittal reformations can be performed with guidelines used for the lateral cervical spine. Particular attention in parasagittal images to the alignment at C0–C1 will avoid missing a subtle incongruity of this typically perfectly matched joint, a finding suggestive of atlanto-occipital instability. Similarly, careful evaluation of sagittal reformations will avoid oversight of “in-plane” (axial plane) fractures, such as type II fractures of the dens and fractures of the horizontal spinous process/lamina. Nonetheless, careful attention to axial images is necessary to detect fractures involving the craniocervical junction,44 transverse processes (potential vertebral artery injury), margins of vertebral bodies, pedicles, lateral mass, or lamina and spinous processes.
The sensitivity of this survey CT for acute bony injuries to the cervical spine is above 95% with specificity around 95%. Although CT does not directly show soft tissue injuries to the spine, focal kyphosis, focal lordosis, and widening of the disk space can be used as with conventional x-rays to suggest associated injuries to soft tissue. Some authors feel that clinically important injuries to soft tissue causing biomechanical instability are almost always evident on technically adequate CTs of the cervical spine (especially on the sagittal and parasagittal reformations).45
Conventional X-Rays of the Spine
Clinical decision rules and expert recommendations provide guidelines as to who does not require an image survey of the cervical spine.46–49 Basically, oriented asymptomatic individuals without findings on a physical examination following trauma do not require subsequent imaging. Imaging of the thoracic and lumbar spine following blunt trauma is indicated when patients present with one or more of the following: (1) signs or symptoms of local injury (pain, tenderness, interspinous step-off); (2) depressed level of consciousness, including intoxication; (3) acute myelopathy or radiculopathy referable to the thoracolumbar spine; and (4) major distracting injury, including concomitant injuries to the cervical spine.50,51
Given the differences in the incidence of injury to the cervical spine in children and the differences in their distribution (far more common in the upper cervical spine) relative to adults, an examination limited to frontal and lateral views is acceptable.52 In infants 0–4 years of age and in children 5–9 years of age, AP, lateral, and open mouth views are satisfactory. All patients aged 10 and over require a minimum of three views to as many as five or six views to adequately survey the cervical spine. The minimum views include an AP view of the craniocervical junction (open mouth view), an AP view of the subdental cervical spine, and a lateral view of the cervical spine that extends down to the C7–T1 interspace. To supplement these three views, bilateral trauma oblique views and a swimmer’s lateral are often used. These views of the lower cervical spine including the cervicothoracic junction are intended to be more conclusive, but are of limited resolution. Trauma oblique views are obtained without moving the patient. The imaging cassette is placed on the surface of the table or gurney on which the patient is lying and positioned such that its leading edge is near the patient’s midline and its inferior edge is beneath a portion of the patient’s shoulder girdle. The x-ray beam is angled at 45° to the plate with the central beam centered on the anterior aspect of the patient’s neck, with approximately 10–15° of cranial angulation. Trauma oblique projections obtained in this fashion show the posterior elements and the end plates of the vertebral body.
Films of the thoracic and lumbar spine are usually obtained as separate sets of frontal and lateral projections. The upper thoracic spine may require the addition of a swimmer’s lateral view, if one has not been previously obtained as part of a cervical spine series, to show the cervicothoracic junction and upper thoracic spine. In general, if pathology is identified, the authors recommend “coned” views of the affected vertebral body. This represents more collimated images centered on the level of abnormality.
When examinations of the cervical or thoracic spine are technically inadequate, it is almost always due to the inability to adequately visualize the cervicothoracic junction and the upper thoracic spine. Swimmer’s lateral views are generally obtained with one arm elevated above the head and the other arm in caudal traction. Obviously, when there are fractures of the upper extremity, it may be either difficult or impossible to humanely obtain such studies in a conscious patient. It is possible for experienced observers to substitute carefully evaluated trauma oblique views for swimmer’s views; however, most centers would use CT in a targeted fashion when the conventional x-rays are inadequate to view this area. To reinforce the point, it is necessary to see the top of T1 on the cervical spine and the bottom of C7 on radiographs of the thoracic spine.
One of the common errors made in evaluating the cervical spine is mistaking developmental variations for pathology (Fig. 15-11). Common variations at the craniocervical junction include the following: fusion of C1 to the occiput, which may be partial or complete; failure of fusion or development of the posterior elements of C1; pseudospreading of C1 relative to C2, which may mimic Jefferson burst fractures (most common in the 0- to 4-year age range, but may be seen up through puberty); pseudosubluxation of C2 on C3 in pediatric patients, which can be recognized as normal by a normal C1–C3 spinolaminar line; and os odontoideum (an anomalous bone that replaces all or part of the dens axis and is not attached to the atlas).
In the thoracic and lumbar spine, the authors recommend a careful count of the vertebral bodies on the frontal examination to establish the correct levels, based on the number of rib-bearing (thoracic) and non-rib-bearing (lumbar) vertebrae. On the lateral view, it is important to look at the corners of the vertebral bodies, especially the anterior superior corner, which is affected in approximately 90% of all vertebral body fractures. On the frontal view, it is most important to evaluate the adjacent end plates for continuity, the lateral margins of the vertebrae, the posterior elements for pathological interspinous and interpediculate widening, and horizontal lucencies that would suggest horizontal soft tissue and/or osseous disruption of a flexion-distraction-type injury.
Once an abnormality is identified on conventional x-rays, CT and/or MRI may be used to further characterize the injury for planning of treatment and provide information on the patient’s prognosis.
Computed Tomography of the Thoracolumbar Spine
Among patients undergoing CT of the chest and/or abdomen, a review of axial images in bone algorithm and bone windows, sagittal reformations, or lateral and AP scanograms (topograms) may be used in lieu of conventional x-rays to study the thoracic and lumbar spine.53 Liberal use of CT for the further evaluation of vertebral body deformities that are thought to be related to trauma based on conventional x-rays is highly recommended. In addition, patients with high-risk mechanisms and impressive signs or symptoms without abnormal x-rays (e.g., a palpable step-off suggesting disruption of the posterior elements) should undergo a thoracolumbar CT to detect occult minimal burst fractures or Chance or flexion-distraction-type injuries.
A 2.5–3.0-mm axial slice thickness is used to acquire images from two vertebral body levels above an abnormality through two vertebral body levels below. Images are reconstructed using both bone and soft tissue algorithms; however, acquisition of the entire thoracic or lumbar spine is advised as it allows more accurate determination of the location of injury. Sagittal reformations are made in both algorithms and viewed at bone and soft tissue windows, respectively.
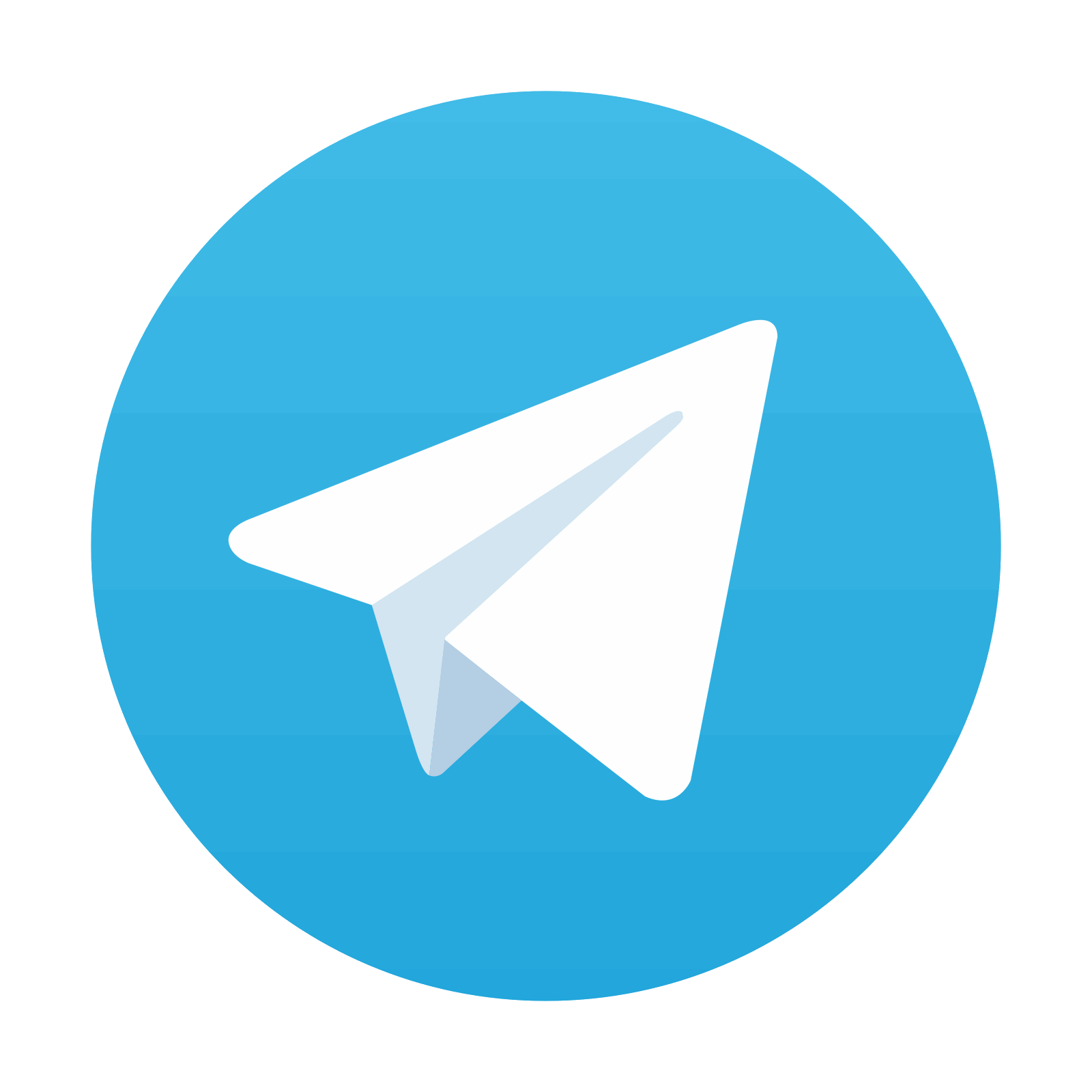
Stay updated, free articles. Join our Telegram channel

Full access? Get Clinical Tree
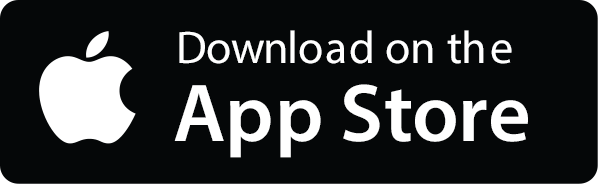
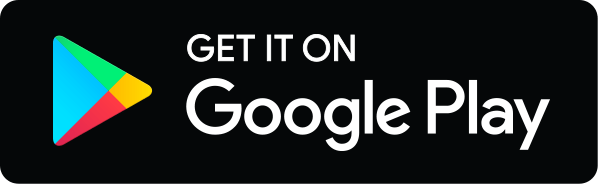