Summary
The prevalence of iron deficiency is high – even in the absence of anaemia – in patients with chronic heart failure (HF). Although iron deficiency is easily diagnosed with two biomarkers (serum ferritin and transferrin saturation), it is underdiagnosed in patients with HF. Iron is not only necessary for red blood cells, but also for cells in tissues with high-energy demands (heart, muscle, brain). Even before the onset of anaemia, HF patients with iron deficiency have decreased physical and cognitive performances and a poorer quality of life. Moreover, iron deficiency is a risk factor, independent of anaemia, of unfavourable outcome (death or heart transplantation) in patients with chronic HF. Several randomized controlled studies have shown improvement in exercise capacity, New York Heart Association functional class and quality of life after correction of iron deficiency. The results of these clinical trials, which are supported by European guidelines, suggest considering iron deficiency in HF as a possible therapeutic target.
Résumé
La prévalence de la carence martiale– même en l’absence d’anémie –est élevée chez les patients présentant une insuffisance cardiaque chronique. Bien que la carence martiale soit facilement diagnostiquée avec deux paramètres biologiques (ferritine sérique et coefficient de saturation de la transferrine), elle reste toutefois sous-diagnostiquée chez ces patients. Le fer est nécessaire, non seulement aux cellules de la lignée érythrocytaire, mais également aux tissus ayant une consommation énergétique importante (cœur, muscles, cerveau). Bien avant que l’anémie ferriprive soit constituée, les patients avec une carence martiale ont des performances physiques et cognitives diminuées et une qualité de vie dégradée. Chez les patients insuffisants cardiaques, la carence martiale est un facteur de risque (décès, transplantation cardiaque), indépendant de l’anémie. Plusieurs essais randomisés contrôlés ont montré l’amélioration des performances physiques, de la classe fonctionnelle NYHA, et de la qualité de vie après correction de la carence martiale. Les résultats de ces essais cliniques, soutenus par des recommandations européennes, suggèrent de considérer la carence martiale des patients insuffisants cardiaques comme une cible thérapeutique potentielle.
Background
This position paper reports data on iron deficiency in patients with heart failure (HF), which are based on: a review and analysis of recent articles on iron metabolism and HF; national and international guidelines for the management of HF, and iron deficiency diagnosis and treatment; and the experience of the authors with iron deficiency in HF patients.
In recent years, iron deficiency has emerged as a newly recognized co-morbidity of chronic HF. Independently of anaemia, iron deficiency occurs frequently in HF patients, contributing to cardiac and peripheral muscle dysfunction, and is a strong predictor of poor clinical outcome . Recent controlled randomized studies have shown that iron treatment in chronic HF has favourable effects on exercise capacity, New York Heart Association (NYHA) functional class, left ventricular ejection fraction (LVEF) and quality of life . Despite this, the diagnosis and management of HF patients with iron deficiency remains largely unrecognized in the cardiologist community. However, diagnostic tools and treatments already exist are relatively inexpensive and may lead to important health benefits for HF patients.
This document is intended for use by cardiologists, and the recommendations herein propose preferred approaches for the diagnosis and treatment of iron deficiency in HF. Recommendations from position papers are not considered to have the prominence of practice guidelines. Nevertheless, based on the latest literature, this position paper should facilitate and improve patient care by presenting the best practices in this emerging area.
Recent perspectives on iron deficiency physiopathology
Iron is necessary not only in the haem of haemoglobin for oxygen transport, but also as a cofactor for several enzymes. For example, iron ions play central roles in the mitochondrial respiratory chain and in tissue oxygen storage in myoglobin . Therefore, iron is necessary in cells that require sustained adenosine triphosphate synthesis, such as skeletal myocytes and cardiomyocytes, in addition to cells of the erythropoietic lineage .
In healthy individuals, approximately two-thirds of body iron is contained in the haemoglobin of mature erythrocytes (1800 mg) and precursors of the erythropoietic lineage (300 mg) . Iron is also stored in liver parenchymal cells (1000 mg), with 10–15% found in myoglobin and different enzymes. About 10 mg of iron are ingested daily, although only 1–2 mg are absorbed by duodenal cells. Approximately 3 mg of iron (< 0.2% of total iron) are bound to serum transferrin. Iron is also recycled from senescent red blood cells (600 mg) in macrophages of the reticuloendothelial system (liver, spleen, bone marrow). Thus, iron is continuously exchanged between senescent red blood cells and bone marrow (daily recycling of 20–25 mg of iron).
Iron metabolism and regulation have been revisited recently, and classical clinical findings, such as iron sequestration during chronic inflammation, are now elucidated at the molecular level . The proteins ferritin and transferrin play key roles in the storage and transport of iron. Ferritin stores iron in tissues and, in practice, serum ferritin is the first indicator of iron stores. Absolute iron deficiency is the result of low serum ferritin levels, reflecting insufficient tissue stores of iron as a consequence of chronic blood loss (e.g. gastrointestinal bleeding due to aspirin or oral anticoagulants) or inadequate iron intake (e.g. elderly, malnutrition, malabsorption).
Transferrin carries iron in plasma and extracellular fluids. The iron-transferrin complex is fixed by the transferrin receptor on the membrane of iron-requiring cells, followed by internalization of transferrin to acquire intracellular iron. In chronic inflammation, iron stores are sufficient, but iron cannot be mobilized from the tissue stores to the circulating pool; this status defines functional iron deficiency. The ferritin level may be within normal or high ranges. In this case, the transferrin saturation (TSAT) level should be measured, with a decrease of TSAT indicating a failure to deliver iron to cells and suggesting a diagnosis of functional iron deficiency. The level of circulating iron decreases due to: iron retention in macrophages of the reticuloendothelial system; and decreased intestinal absorption of iron . Retention of iron in macrophages and decreased absorption of iron by intestinal cells are related to increased synthesis of hepcidin, a newly discovered peptide produced by the liver that plays a central role in iron metabolism regulation . There is an important therapeutic consequence of this mechanism: correction of iron deficiency is more rapid and more efficient via the intravenous route compared with oral administration because the intestinal barrier is bypassed and iron sequestration is overcome . These mechanisms have important implications in inflammatory diseases or when malabsorption is present, including HF, which is often associated with mild inflammation and with malabsorption in case of peritoneal oedema.
Clinical consequences of iron deficiency
The best known consequence of iron deficiency is anaemia; however, anaemia is the result of advanced-stage iron deficiency. Iron deficiency has important clinical consequences before the onset of anaemia , with many chronic diseases worsening if iron deficiency occurs, even in the absence of anaemia . In a systematic literature review of animal and human studies, the causal relationship between iron deficiency and decreased physical capacity/work was clearly established, even in patients without anaemia. This impairment of exercise capacity is related to mitochondrial dysfunction and a decrease of oxygen storage in myoglobin, resulting in decreased energetic efficiency . The consequences of iron deficiency on cognitive performances, emotions and behaviour have also been demonstrated . Of note, exercise performance and cognition in patients with iron deficiency were improved after iron supplementation .
Other experimental or clinical studies have shown more specifically the consequences of iron deficiency on cardiac muscle. In an animal model, chronic iron deficiency resulted in structural abnormalities, with increased size and weight of the heart . A recent study reported that iron content and transferrin receptor expression were decreased in cardiomyocytes from HF patients . In a prospective study in patients with chronic HF, patients who were non-anaemic and iron deficient had a 2-fold greater risk of death than anaemic iron-replete patients . Iron deficiency was shown to be a strong predictor, independent of anaemia, of unfavourable outcome (e.g. death or heart transplantation) in patients with systolic chronic HF . Iron deficiency was also an independent predictor of mortality in chronic HF patients; disease severity and the level of the N-terminal fragment of pro-B-type natriuretic peptide (NT-proBNP) were associated with the degree of iron deficiency .
Prevalence of iron deficiency in patients with heart failure
Iron deficiency occurs frequently (30–50%) in patients with chronic HF, with or without anaemia . In a cohort study of 955 patients with HF, iron deficiency was reported in 43% of anaemic patients and 15% of non-anaemic patients . In a separate study, iron deficiency anaemia was diagnosed by bone marrow aspiration in 73% of 37 patients with advanced HF . More recently, iron deficiency was diagnosed in 37% of 546 patients with HF (including 32% of patients without anaemia) . In 157 patients with chronic HF, functional iron deficiency was diagnosed in 43% of patients, although there was apparently no deficiency of iron stores . In an international study, the prevalence of iron deficiency was 50% in 1506 patients with chronic HF (including 46% of patients without anaemia) . A recent cohort study in patients hospitalized for decompensation of HF reported iron deficiency in 69% of men and 75% of women (57% and 79% in non-anaemic patients, respectively) .
Diagnosis and initial evaluation of iron deficiency in heart failure
Biological marker of iron stores: serum ferritin
Values of serum iron have substantial variation between HF patients, in addition to nycthemeral variations. Therefore, measurement of serum iron is no longer recommended for the diagnosis of iron deficiency. Instead, the level of serum ferritin, which stores iron in tissues in a non-toxic form, is used as a marker of iron deficiency, because serum ferritin levels correlate with total body iron stores.
In the general population, reference ranges of serum ferritin are within 15–300 μg/L . Iron deficiency is considered to be certain for serum ferritin < 15 μg/L . However, ferritin is also an acute phase inflammatory protein and, as such, its concentration increases in chronic HF and other chronic diseases due to inflammation processes. Therefore, in patients with chronic diseases, increased ferritin levels do not always reflect high iron stores, and the customary thresholds that indicate iron deficiency may not apply. Nanas et al. showed that serum ferritin, at a cut-off at 17 μg/L, minimized the incidence of iron deficiency compared with the gold standard, which is bone marrow aspiration with specific staining of iron (Perls’ Prussian blue staining of ferric irons in tissue, mostly within ferritin) . Coenen et al. showed that, in patients who had anaemia associated with chronic disease and ferritin concentrations < 70 μg/L, iron deficiency, as determined by bone marrow iron stain, was always present . One method to account for the increase in ferritin values caused by inflammation is to raise the cut-off that defines iron deficiency . Current thresholds for serum ferritin are derived largely from the clinical literature, specifically from studies examining the highest ferritin concentration among patients with microcytic iron deficiency anaemia who had no stainable iron in the bone marrow (or, alternatively, who showed a therapeutic response to iron) .
Biological marker of iron delivery to cells: transferrin saturation
Transferrin is the specific transport protein for iron in plasma; it delivers iron to cells via the transferrin receptor. Serum transferrin can be measured directly by a specific immunological assay or indirectly by determining the total iron binding capacity (TIBC), as transferrin is the primary iron-transport protein. Because transferrin concentration is sensitive to several factors, it is not used in practice to determine iron deficiency .
TSAT is a frequently used marker of iron delivery to cells that assesses the fraction of sites on transferrin that are actually occupied by iron ions; it is calculated by dividing the serum iron concentration by the serum transferrin concentration (or by the concentration of the substitute TIBC). TSAT represents the amount of iron available to erythroblasts and other iron-requiring cells, and it is not (or slightly) affected by inflammation in chronic diseases; a TSAT < 20% is insufficient to meet normal requirements for erythropoiesis .
Available guidelines for the diagnosis of iron deficiency
In the 2012 guidelines of the European Society of Cardiology (ESC) for the diagnosis and treatment of HF , the evaluation of iron status is recommended in all ambulatory patients suspected of having HF. In these guidelines, iron deficiency is defined in accordance with the selection criteria for iron treatment in the study by Anker et al. : ferritin < 100 μg/L; or ferritin 100–299 μg/L plus TSAT < 20% ( Table 1 ). Use of this pragmatic definition allows the identification of patients with chronic HF who could benefit from iron replenishment.
European Society of Cardiology 2012: general guidelines for HF | French Health Agency (Haute Autorité de santé) 2011: general guidelines for iron deficiency | Present position paper: Expert opinion specific to iron deficiency in HF | |
---|---|---|---|
Diagnosis of iron deficiency | Iron deficiency is a co-morbidity of HF that must be detected | For suspicion of iron deficiency, serum ferritin is the first-line iron variable (no other biological variable is necessary) | Iron deficiency should be systematically assessed in all HF patients |
Iron deficiency is defined by the selection criteria for chronic HF patients eligible for iron treatment in the study by Anker et al. : ferritin < 100 μg/L or ferritin 100–299 μg/L and TSAT < 20% | In the case of inflammation, chronic renal insufficiency or non-contributory result of serum ferritin (normal or elevated value with strong suspicion of iron deficiency), serum iron associated with transferrin (for calculation of TSAT) can help in the diagnosis | Iron deficiency is defined by: ferritin < 100 μg/L (absolute iron deficiency) or serum ferritin 100–299 μg/L and TSAT < 20% (functional iron deficiency) | |
No indication for diagnosis by serum iron alone or in addition to serum ferritin | |||
Treatment of iron deficiency | Iron therapy may be considered as a treatment for chronic HF patients (correction of iron deficiency in the study by Anker et al. used intravenous iron) | No specific recommendations for the treatment of iron deficiency in HF patients | For all patients with chronic HF or hospitalized for acute decompensation of chronic HF with biological evidence of iron insufficiency, iron supplementation should be considered |
The effects of treating iron deficiency in HF with preservation of ejection fraction and the long-term safety of iron therapy in HF are unknown | Oral iron supplementation is the first-line treatment, with assessment of efficacy after 3 months | ||
Intravenous iron (iron sucrose, iron dextran, ferric carboxymaltose) should be administered if oral iron is inefficient or poorly tolerated, or if a rapid increase in iron stores is needed (e.g. in symptomatic anaemia) | |||
The efficacy of intravenous iron should be assessed after 3 months |
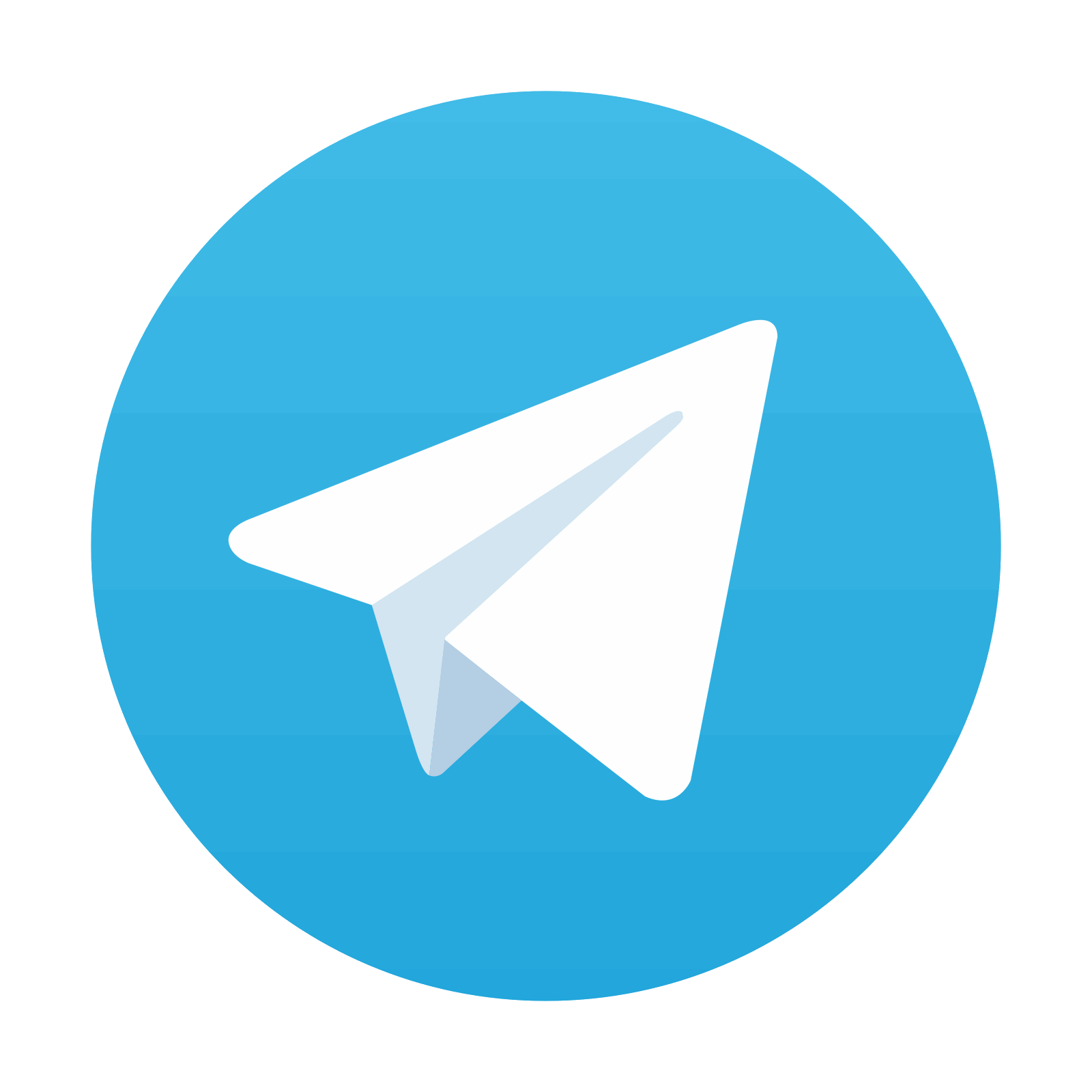
Stay updated, free articles. Join our Telegram channel

Full access? Get Clinical Tree
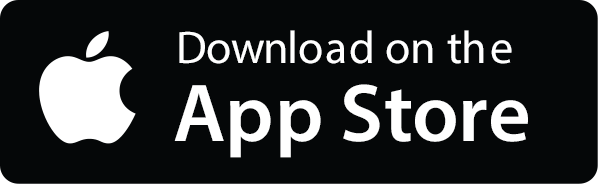
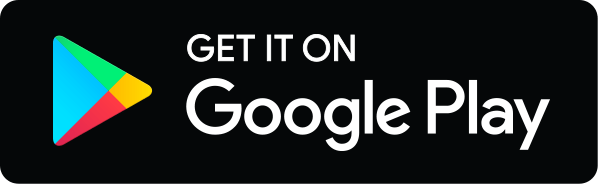
