Fig. 16.1
Risks factors and mechanisms that plausibly link diabetes with vascular disease. ROS reactive oxygen species, NO nitric oxide
16.2.1 Endothelial Dysfunction
The endothelium has a central role in vascular homeostasis; it permits the vasculature to adapt to several stimuli and is fundamental for regulation of vascular tone and structure. Endothelial cells produce a broad spectrum of substances, of which the most characterized is nitric oxide (NO) that is generated from the metabolism of L-arginine by the endothelial NO synthase (eNOS) constitutively expressed by endothelial cells [13]. Under normal conditions, endothelial stimulation induces the production and release of NO, which diffuses to circumjacent tissue and cells and promotes its cardiovascular protective effects by relaxing media layer smooth muscle cells and by preventing adhesion molecule expression, leukocyte adhesion, and migration into the arterial wall, muscle cell proliferation, and platelet adhesion and aggregation [13]. The regulation of endothelial function is largely vascular area specific, hence promoting different responses in various organs and tissues. Within the same vascular area, it depends mainly on the vessel size, that is, large arteries (macrocirculation) and arterioles (microcirculation) [14]. In disease conditions, such as in diabetes, hypertension, and dyslipidemia, the endothelium undergoes functional and structural alterations and loses its protective vascular role, becoming a pro-atherothrombotic structure [12–14]. In the initial stages, the main endothelial alteration is functional and is referred as endothelial dysfunction. Impaired NO bioavailability is characteristic of this condition. It can be due to a reduced production of NO or, more frequently, to an increased breakdown by reactive oxygen species (ROS) [13]. The endothelium activates several pathways, trying to compensate NO deficiency. The production and release of other endothelium-derived vasodilators such as prostanoids and other endothelium-derived hyperpolarizing factors guarantee some, although impaired, endothelium-dependent vasodilatation. In association with NO deficiency, the endothelium produces substances and mediators that injure the arterial wall, including endothelin, thromboxane A2, prostaglandin H2, and ROS [13]. Diabetes-related endothelial dysfunction is present early at disease beginning or at prediabetic conditions and antecedes morphologic and structural vascular changes [13, 15], which includes accelerated disappearance of capillary endothelium [16], weakening of intercellular junctions [17], altered proteins synthesis, and altered expression/production of adhesion glycoproteins on endothelial cells [16–19], which promote attachment of monocytes and leukocytes and their transendothelial migration [17]. Endothelial dysfunction is the initial focus of atherosclerotic lesions that appears later during the course of diabetes [20, 21] and has itself been associated with adverse cardiovascular prognosis [22].
16.2.2 Biochemical Mechanisms Associated with Vascular Damage in Diabetes
In cells where glucose transport is not completely dependent on insulin (e.g., in vascular endothelial, renal, and retinal cells, as well as in peripheral nerves [23]), hyperglycemia increases glucose intracellular concentration and several other glycolytic intermediates [24] that are critical substrates for various important biochemical pathways [25]. In the presence of chronic hyperglycemia, there is an inappropriate downregulation of insulin-dependent transporters, which exposes cells to the continuous influx of high amount of glucose from the extracellular space into cytosol. This promotes production of excess intracellular ROS [26–28]. ROS are the final inducers and also the initiating elements of, at minimum, four interrelated hyperglycemia-activated paths: (1) the polyol pathway, with its associated alterations in the redox state of nicotinamide-adenine dinucleotide phosphate (NADP) and its reduced form NADPH [28, 29]; (2) the covalent change of intracellular components by reactive advanced glycation end products (AGEs) [30, 31]; (3) the de novo synthesis of diacylglycerol, which makes active several protein kinase C isoforms [32, 33]; and (4) the augmented flux by the hexosamine path [27, 28]. The activation of these biochemical ways, at minimum partially mediated by glucose-related increased osmolarity [34], can explain why persistent elevation of glucose affects the biochemical homeostasis of cardiovascular cells, finally leading to the evolvement of diabetic vascular (mainly micro) complications [35]. Each of the pathways mentioned above is activated by hyperglycemia-induced ROS formation, in the form of an overproduction of superoxide anion through the mitochondrial electron transport chain [36], in conjunction with an overproduction of NO [37], which jointly lead to production of a toxic reaction product, the peroxynitrite anion [38, 39].
Hyperglycemia is related to increased oxidation stress [26], augmented leukocyte-endothelial interaction [40], and glycation of constitutional body proteins, including lipoproteins, apolipoproteins, and clotting factors, which progressively increase vasomotor tone, vascular permeability, growth, and remodeling [15–18]. Furthermore, hyperglycemia retards endothelial cell replication [15, 18] and increases extracellular matrix production [41], which may thicken the basal membrane. It also augments endothelial cell death [15, 18] and enzymes involved in collagen synthesis [41]. Long-term hyperglycemia, but not only this, leads to the formation of modified molecular species, by nonenzymatic reactions between the aldehydic group of reducing sugars with proteins, lipids, or nucleic acids [42]. The formation of these advanced glycation end products (AGEs) occurs mainly by glycation, by the polyol pathway, and by glycoxidation and oxidative stress [43]. All the conditions that promote AGEs formation are present in diabetes and are aggravated by the occurrence of renal failure [44]. AGEs promote proinflammatory cellular reactions that can damage tissues, frequently directed to specific organs. AGEs are involved in atherosclerosis development by at least two mechanisms: by direct tissue deposition and by interaction with the receptor of AGEs (RAGE) [43]. Due to tissue accumulation, AGEs directly cross-link with proteins of extracellular matrix (ECM), increasing arterial stiffness and entrapping other macromolecules [45]. The other mechanism by which AGEs promote their harmful effects is by receptor mediated paths, and RAGE is the most investigated receptor [46]. The RAGE-AGE interaction alters cellular signaling, promotes gene expression, and increases the discharge of proinflammatory substances [47]. The RAGE interacts with AGEs and also with various different proinflammatory ligands that, like AGEs, augment the atherosclerotic process [48]. In atherogenesis, this multiligand receptor is highly expressed in activated endothelial cells, vascular smooth muscle cells, and inflammatory cells and, through interaction with its ligands, begins and maintains the pathologic process leading to atherosclerotic lesion in the arterial intima [48]. These mechanisms are summarized on Fig. 16.2.
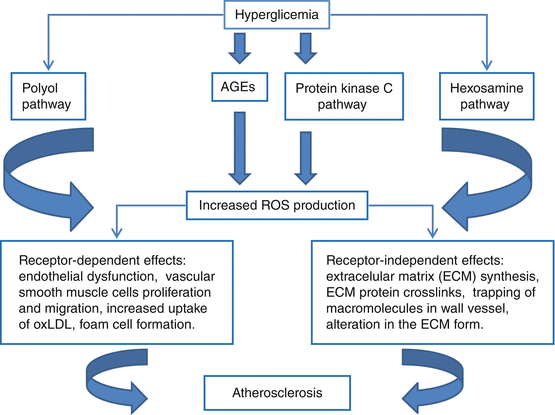
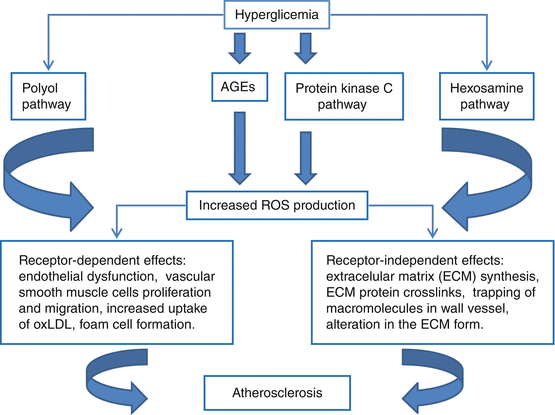
Fig. 16.2
Schematic model of vascular lesion associated with chronic hyperglycemia. ROS reactive oxygen species, AGEs, advanced glycation end products
16.2.3 Insulin Resistance
Insulin resistance, present in most of type 2 diabetic patients, is associated with impaired vascular insulin signaling and blunted vascular effects of insulin [49]. The mechanisms of insulin resistance are yet incompletely understood. Insulin resistance may reflect the combined influence of obesity, dietary patterns, and lifestyle and can also result in elevated blood pressure, impaired glucose, fat and lipid metabolism, inflammation, and a prothrombotic environment [50]. The most sensitive tissues for the insulin-glucose uptake reaction are the skeletal muscle and adipose tissue. However, insulin receptor signaling exerts important biological effects on vascular cells and regulates vessel dilation and contraction [51, 52]. Indeed, insulin resistance decreases blood flow to skeletal muscle [16, 53]. Moreover, insulin receptor signaling regulates monocyte differentiation into macrophages [54]. Hyperglycemia is not only a consequence but also a worsening factor for insulin resistance. Impaired insulin signaling in tissues impacts on vascular dysfunction, hypertension, hyperglycemia, dyslipidemia, and other metabolic disorders. These risk factors jointly contribute to endothelial dysfunction, an early event in atherosclerosis development [12, 13, 55].
16.3 The Link Between Micro- and Macrovascular Disease in Diabetes
Both types of vascular complications share common pathogenetic mechanisms [56–58]. The walls of the large arteries receive their nutrient and oxygen supply from the lumen and also from small vessels located in the adventitia. This plexus of microvessels in the arterial wall of the arteries is called the vasa vasorum [59]. Several studies suggested that microvascular disease may contribute to atherosclerotic progression in type 2 diabetes [56–58, 60–63]. By focusing on the role of neovascularization of vasa vasorum in promoting atherosclerosis progression and plaque rupture, these investigations support the link between micro- and macrovascular disease. Large-artery microvessels may also be targets to diabetic damage, in the same way it occurs in the microvessels of the retina, of the kidney glomerulus, and of the peripheral nerves [64]. A study including young patients with type 1 diabetes showed that the vasa vasorum of the aorta presented pathological characteristics of diabetic microvascular disease and that the lesion of vasa vasorum was an important determinant of aortic atherosclerosis development [65]. Another investigation also demonstrated increased microvessels in the aorta of diabetic patients in relation to nondiabetic individuals [66]. It is plausible that its physiopathological mechanisms involve a response of the microcirculation of the artery wall to ischemia and hypoxia [59], similar to which also leads to microvascular alterations in other territories, like the retina in diabetes [67]. Taking into account these previous investigations suggesting the participation of the vessel-wall microcirculation in the pathogenesis of diabetic atherosclerosis [56–58, 63, 64], Arcidiacono and colleagues proposed that the microangiopathy of the wall of large arteries could provide, at least in part, the explanation for the more severe and rapidly evolving form of atherosclerotic process observed in diabetes [64].
16.4 Screening for Vascular Disease in Diabetes
The presence of subclinical vascular disease confers an increased cardiovascular morbidity and mortality in patients with diabetes [68]. However, screening tests did not decrease the occurrence of cardiovascular events in patients with diabetes [69]. Nonetheless, several ways of evaluating noninvasively the presence of vascular disease may improve cardiovascular stratification risk, particularly, in patients at intermediate risk [68]. These methods are better predictor than biomarkers, such as ultrasensitive reactive C protein and lipoprotein-associated phospholipase A2. The identification of patients at increased cardiovascular risk may permit interventions to reduce the burden of vascular disease, hence of vascular outcomes, and may improve compliance and adherence to treatment [68]. The measurement of common carotid intima-media thickness and plaques by ultrasonography and of central aortic arterial stiffness by carotid-femoral pulse wave velocity are among the simple, easily available and safe methods that can be employed for investigation of subclinical vascular disease [68]. Coronary calcium score, although can also improve cardiovascular risk stratification, has higher costs and a lower availability; so, its use is not easily implemented in clinical practice [70, 71]. The increase in the wall-to-lumen ratio of small arteries in adipose tissue of gluteal biopsies can refine evaluation of risk in diabetic patients [72]; nevertheless, because of its invasiveness, it is also not appropriate for general practice. Similarly, endothelial dysfunction has been associated with an increased risk of vascular outcomes [21], but it still lacks simpler and noninvasive techniques for investigating endothelial responsiveness to various stimuli. Additionally, the added value of using imaging methods to improving patient outcomes has not been demonstrated yet in randomized controlled studies [68].
Physical examination, fundoscopic exam performed by an ophthalmologist, electrocardiogram, and laboratory examination can identify the presence of asymptomatic micro- and macrovascular diabetic disease. Table 16.1 summarizes the routine exams that should be performed in diabetic patients to investigate the presence of micro- and macrovascular disease.
Table 16.1
Summary of routine examinations that should be performed in patients with diabetes to identify the presence of macro- and microvascular complications
Physical examination |
Palpation of dorsalis pedis and posterior tibial pulses |
Ankle-brachial index measurement |
Neurologic examination of lower limbs, including: |
Patellar and Achilles tendinous reflexes |
Evaluation of proprioception, vibration and monofilament sensation |
Retinal fundoscopy |
Laboratory exams |
Urinary albumin excretion with spot urine albumin-to-creatinine ratio |
Serum creatinine and estimated glomerular filtration rate |
Resting electrocardiogram |
16.5 Treatment of Vascular Disease
There are various evidences from clinical trials that treatment of hyperglycemia, hypertension, dyslipidemia, and antiplatelet therapy are able to reduce morbidity and mortality related to vascular disease [73–76]. Indeed, multifactorial risk factors treatment seems to have greatest benefit on reducing cardiovascular morbidity and mortality in type 2 diabetes [77]. Table 16.2 summarizes the current treatment recommendations for diabetes aiming to reduce cardiovascular disease burden.
Table 16.2
Schematic recommendations for glycemic, blood pressure, lipid, and antiplatelet treatment in diabetes
Parameter | Treatment target | Medication |
---|---|---|
Glycemic control – HbA1c | <7 %a | Metformin plus other oral drugs or insulin in type 2 diabetes |
Blood pressure control | <140/85–80 mmHgb | ACE inhibitors or ARBs as drugs of choice plus CCBs or diuretics |
Lipid control – LDL-cholesterol | <100 mg/dl (individuals without CVD) | High-intensity statins |
<70 mg/dl (individuals with CVD) | ||
Antiplatelet treatment | Primary preventionc | Aspirin (75–162 mg) |
Secondary prevention | Aspirin (75–162 mg) clopidogrel (75 mg) for patients with aspirin contraindication | |
Acute coronary syndromes | Consider double antiplatelet therapy for at least 1 year |
16.5.1 Hyperglycemia
The relation between glycemic control and development of microvascular complications is clearer than for macrovascular disease [5]. A meta-analysis, including 33,040 diabetic patients from five randomized clinical trials comparing conventional vs. tight glycemic control, demonstrated a 17 % reduction in myocardial infarction risk without effect on stroke or on all-cause mortality [74]. Based on three recent randomized studies [78–80] in high-risk patients with type 2 diabetes that could not demonstrate a reduction in cardiovascular risk with HbA1c values lower than 6.5 % in relation to standard values (7–7.9 %), current recommendation is a target HbA1c lower than 7 % [5].
16.5.2 Hypertension
Hypertension affects most of patients with type 2 diabetes and patients with type 1 diabetes with underlying nephropathy. It is a major risk factor for both micro- and macrovascular disease development and progression. In a meta-analysis, clinic blood pressure values >115/75 mmHg have been associated with increased cardiovascular events and mortality risks in general populations [81]. However, randomized clinical trials demonstrated beneficial effect of reduction of systolic blood pressure to lower than 140 mmHg and of diastolic pressure to lower than 80–85 mmHg [82–84]. Currently, there is not enough evidence of benefits of lower blood pressure thresholds in diabetes, and the present recommendation is a clinic blood pressure target of <140/80 mmHg [5]. Ambulatory blood pressure monitoring seems to provide better cardiovascular risk stratification than clinic blood pressures in type 2 diabetes, with lower blood pressure targets, and should be performed whenever available [85].
The renin-angiotensin system inhibitors may be particularly beneficial in initial hypertension treatment in patients with diabetes. They may have effects in atherogenesis prevention and renal protection beyond blood pressure lowering [86]. Although some studies have shown superiority of renin-angiotensin system antagonists in reducing cardiovascular risk over other antihypertensive drug classes in patients with diabetes [87–89], a meta-analysis demonstrated no significant differences among antihypertensive drugs in improving patient prognosis, suggesting that the blood pressure reduction is the most important effect of these medications [90]. Indeed, most diabetic patients with hypertension will need multiple drug therapies to achieve blood pressure targets and commonly will present resistant hypertension, but renin-angiotensin system antagonists remain the cornerstone of antihypertensive drug treatment in patients with diabetes [5].
16.5.3 Dyslipidemia
Diabetic patients have an increased prevalence of lipid abnormalities that augments their already high cardiovascular risk. Several interventions may help to achieve lipid level targets, such as physical activity, diet changes, and weight loss. Many clinical trials demonstrated a reduction in cardiovascular risk, both in primary and secondary prevention, with statins use in patients with diabetes [91–97]. A meta-analysis in diabetes, including 14 randomized trials of statins, confirmed a reduction of global and vascular mortality [98]. LDL-cholesterol shall be <100 mg/dl (2.6 mmol/L) in patients without manifest cardiovascular disease and <70 mg/dl (1.8 mmol/L) in those patients with clinical cardiovascular disease [5, 99]. As in nondiabetic individuals, the beneficial effects of statins are greater in diabetic patients with higher baseline risk [5].
The combination of low HDL-cholesterol and high triglycerides is the most common dyslipidemia in type 2 diabetes. There are few studies investigating if treating these lipid abnormalities reduces cardiovascular outcomes. A study using gemfibrozil, demonstrated a reduction in cardiovascular risk, in a subgroup analysis of diabetic patients [100]. However, a study performed in diabetic patients using fenofibrate did not demonstrate reduction in cardiovascular risk [101]. Therefore, unless there are severe hypertriglyceridemia (>1,000 mg/dl) and consequently an increased risk pancreatitis, the target should be reducing LDL-cholesterol with high-intensity statin treatment, because there is not sufficient evidence for benefits of HDL-cholesterol or triglycerides treatment as there is for statins [5]. Also, there is no current evidence that supports the association of statins with fibrates to reduce cardiovascular risk in diabetes [102, 103]. The association of statins with fibrates increases the risk of augment of aminotransferases, myositis, and rhabdomyolysis, particularly in patients with renal failure. This risk seems to be higher with gemfibrozil [104].
16.5.4 Antiplatelet Treatment
Aspirin was demonstrated to reduce cardiovascular mortality in patients with previous myocardial infarction and stroke (secondary prevention) [105]. Its beneficial effect is less clear in primary prevention [106, 107]. Aspirin is currently recommended (75–162 mg) in diabetic patients with a history of cardiovascular disease for secondary prevention. For primary prevention, they are recommended for type 1 or type 2 diabetes at increased cardiovascular risk (10-year risk of event greater than 10 %). This includes men >50 years or women >60 years and patients who have at least one major risk factor (family history of cardiovascular disease, hypertension, dyslipidemia, smoking, or albuminuria) [108]. In diabetic patients with cardiovascular disease with contraindication to aspirin use, clopidogrel 75 mg should be used. In patients with acute coronary syndromes, double antiplatelet therapy is recommended for at least 1 year [105].
16.6 Conclusions
Currently, treatment is based on the control of the risk factors that begin the vasculopathy, by keeping strict glycemic, lipid, and blood pressures control, in conjunction with regular physical activity, changes in diet habits, weight control, and pharmacological interventions. In a near future, we hope basic research on the mechanisms of vascular disease may provide data to discover new therapeutic interventions that can further reduce the diabetic vascular disease burden and improve its prognosis.
References
1.
International Diabetes Federation (2013) IDF diabetes atlas, 6th edn. International Diabetes Federation, Brussels, http://www.idf.org/diabetesatlas
2.
Barceló A, Aedo C, Rajpathak S, Robles S (2003) The cost of diabetes in Latin America and the Caribbean. Bull World Health Organ 81:19–27PubMedCentralPubMed
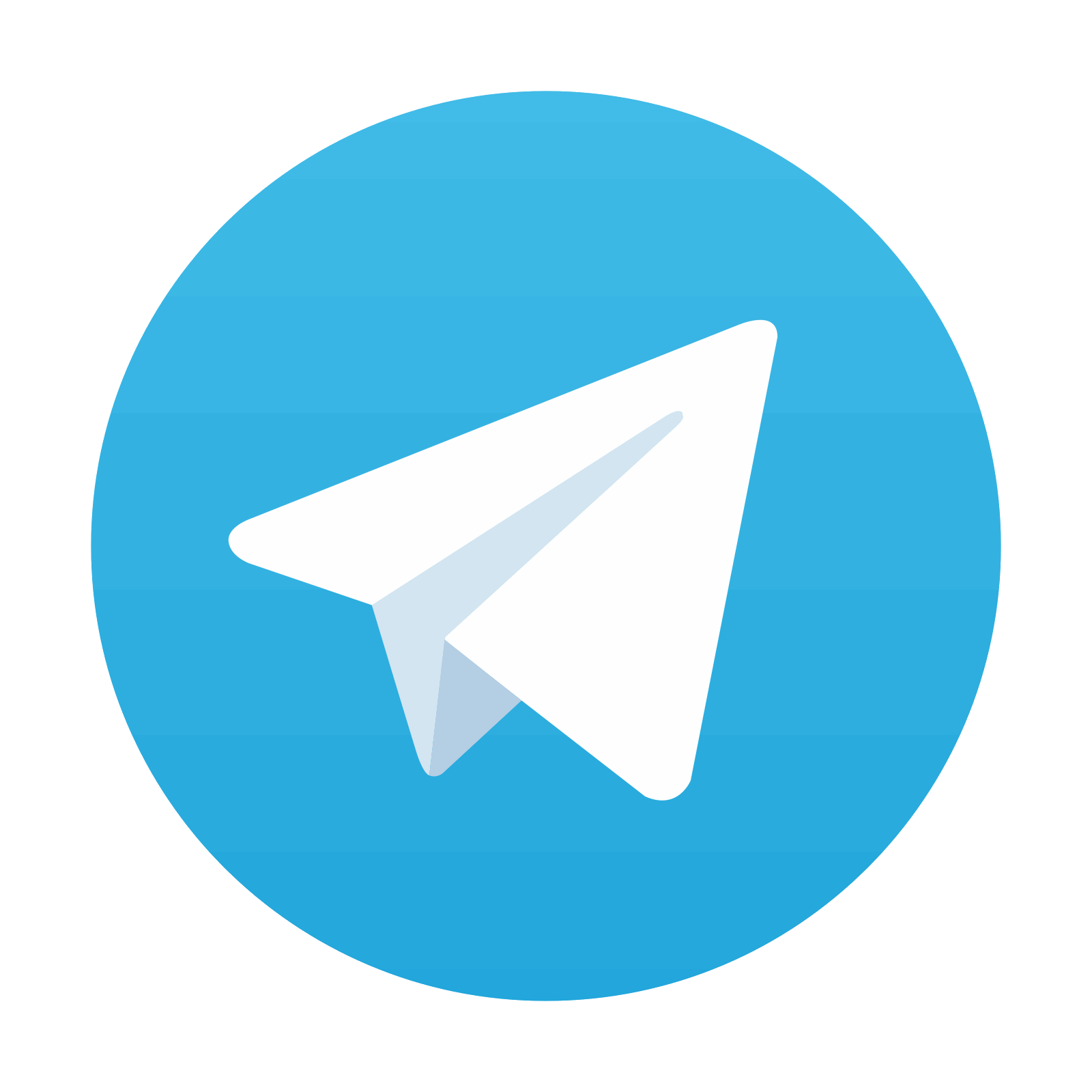
Stay updated, free articles. Join our Telegram channel

Full access? Get Clinical Tree
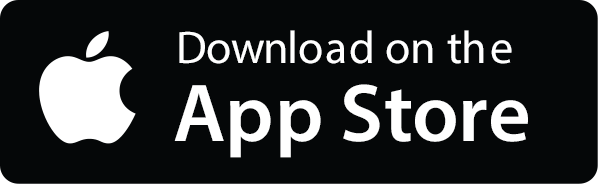
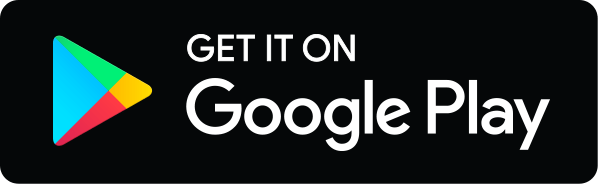