Devices for Heart Failure
Heart failure is a growing health care epidemic. It is estimated that in the United States, there are more than 200,000 people over the age of 45 who have end-stage heart failure for which medical therapy is insufficient.1 Currently, the gold standard treatment for end-stage heart failure is cardiac transplantation. However, given the limited availability of donor hearts, mechanical circulatory support (MCS) with left ventricular assist devices (LVADs) has become an alternative for patients as a bridge-to-transplantation (BTT) until a suitable donor heart becomes available,2 a permanent support as destination therapy (DT),3,4 or in certain situations as a bridge-to-recovery permitting explantation of the device, once there is normalization of cardiac function. The concept of bridge-to-candidacy is occasionally utilized in individuals who have contraindications to transplant at the time of LVAD implant, such as high body mass index (BMI), unacceptable pulmonary hypertension, malignancy, active tobacco or substance use, or untreated or unresolved psychosocial situations, which once resolved may qualify the individual for transplant listing.
There have been significant developments and advances in the design of mechanical assist devices over the past 40 years, such that utilization of these devices has presently become the standard of care in appropriate patients with end-stage heart failure at experienced medical centers worldwide. In this chapter we review the evolution of MCS, including temporary support with the intra-aortic balloon pump (IABP) and extracorporeal membrane oxygenation (ECMO), as well as more permanent available devices for the treatment for advanced heart failure, optimal patient selection, survival outcomes, potential adverse events following MCS implantation and landmark clinical trials. This overview addresses these critical issues and provides a framework for answering expected questions on the General Cardiovascular Board Certification Examination.
IMPORTANT CONSIDERATIONS REGARDING MECHANICAL CIRCULATORY SUPPORT DEVICES
MCS devices were initially developed to bridge patients to recovery versus a bridge, or alternative, to transplantation. Dr. Michael E. DeBakey, in the early 1960s, was the first to report successes with insertion of rudimentary left ventricular assist prototypes. This was the time when open heart surgery was evolving rapidly, but substantial limitations to cardiopulmonary bypass (CPB) were present. In an effort to transiently support patients who were unable to be weaned from CPB after open heart surgery, pneumatically displaced pulsatile devices were used. Slowly, devices evolved with improvements that allowed successful bridging to recovery from postcardiotomy shock and CPB, and in patients with acute myocarditis or in the peri-infarction period, when stunning of potentially viable myocardial tissue had occurred. Use of these devices for patients with advanced heart failure subsequently became more accepted as an option to stabilize a patient before cardiac transplantation. In some patients, though infrequently, recovery of ventricular function occurred and device removal was possible.
Table 18.1 summarizes issues to consider when MCS devices may be possible treatment options. Clinical settings that should prompt consideration of device use commonly include CBP wean failure, acute cardiogenic shock (usually in the setting of an acute coronary syndrome with massive myocardial infarction or fulminant myocarditis), and in the chronic, severely decompensated heart failure patient (American College of Cardiology [ACC]/American Heart Association [AHA] and Heart Failure stage D) as a BTT or as an alternative to transplantation. Rarely, MCS devices are inserted during resuscitative efforts in individuals with the sudden cardiac death syndrome.
TABLE
18.1 MCS Devices: Considerations
When making decisions regarding the type of MCS required, consideration of the support system role becomes important. This is related to whether biventricular function assistance is required or individual ventricular assistance is needed. There are times where hybrid device pairings are utilized. For example, once an LVAD is implanted and the patient develops subsequent right ventricle (RV) failure, a temporary right ventricular assist device (RVAD) may be implanted until there is enough RV recovery that allows explant or eventually transplant. If a total artificial heart (TAH) is implanted, the only indication for this is as a bridge to transplant. With a portable driver that is now approved, these patients will be discharged to home to wait for transplant, which was never possible prior to this development.
TYPES OF MECHANICAL SUPPORT DEVICES
The most commonly used MCS device is the IABP. This is a relatively simple device that can be inserted percutaneously through the femoral or axillary artery (on occasion it is inserted intraoperatively and in other configurations) and produces short-term diastolic blood flow augmentation that is particularly beneficial in acute coronary syndromes. The device unloads the heart during systole and augments diastolic retrograde perfusion of the coronary arteries by inflating at precisely timed moments within the diastolic cardiac cycle. Often, placement of the IABP is a bridge to a more sophisticated MCS device. IABPs can be used only for several days before risk of arterial trauma, infection, device failure, or thrombotic complications force their removal. Flow augmentation is not substantial with these devices, but afterload reduction and increased coronary perfusion frequently is of enough benefit to see patients recover significant ventricular function and survive cardiogenic shock. Advantages of IABPs include their ready availability, ease of insertion, simplistic design, and relatively low cost.
Extracorporeal support devices include continuous-flow (CF) axial or centrifugal pumps that can be inserted percutaneously or during an operative procedure to expose, most commonly, the femoral arteries and veins. A frequently used CF extracorporeal device in an acute situation is the Biomedicus CF device, which is sometimes coupled with an oxygenator to provide CBP. Placing an oxygenator between the device and the arterial input cannula is the configuration referred to as ECMO support. More sophisticated extracorporeal devices are placed at the time of thoracotomy and cardiotomy and generally are pneumatic or electric motor displacement devices with pumping chambers connected paracorporeally to exteriorized cannula. The cannula can be set up in a variety of configurations so that left ventricular, right ventricular, or biventricular bypass can be achieved. The first successful use of a ventricular assist device was by Dr. Michael E. DeBakey in 1966, when an extracorporeal pneumatically displaced pulsatile device was used in a patient who could not be weaned from CPB after valvular heart surgery for rheumatic heart disease with severe heart failure. A cannula was placed in the left atrium for inflow into the device, with the arterial outflow cannula anastomosed to the right axillary artery. The patient survived the operative procedure, was extubated without complications, and native heart recovery occurred. The device was removed in the intensive care unit under local anesthesia, with the cannula being occluded subcutaneously and then allowed to thrombose. The patient survived hospital discharge and led an active life until being mortally injured in an automobile accident.
Intracorporeal devices have been a desirable goal because of the potential for complete component implantation. With a completely implantable system, there are no percutaneous connections and the risk of infection is decreased dramatically. However, there are multiple controlling elements that must be buried within the patient, including the pump itself, cardiac and arterial conduits, a compliance chamber (for pulsatile devices), a pump controller, and a power system with transcutaneous capabilities for energy transfer. Because of the complexity surrounding development of such a device, present intracorporeal devices are for the most part only partially implanted devices. These devices can be implanted intrathoracically or subdiaphragmatically and are either CF or pulsatile units.
COMPONENTS AND CONFIGURATIONS OF MECHANICAL CIRCULATORY ASSIST DEVICES
The first generation of pumps are affixed to inflow and outflow conduits and valves to control blood direction, with the pump chamber itself located between the valves and conduits. The interior of the pump represents the blood component–device interface, where problems such as thrombosis and hemolysis begin. The pump controller is linked to circulatory pressure and an electrocardiographic monitor. Sophisticated electronics regulate a pressure–rhythm pump interface system that usually controls the amount of flow and pressure a device generates. The pump driver or activator is the actual flow-generating system and is either a pneumatically displaced diaphragm or an electric motor moving pusher plates back and forth. The pump requires a power source, and this must come from wall-socket alternating current or some form of a battery pack. Because pneumatic pulsatile devices require displacement of gas-driven volume, an air-displacement management system is necessary, and this can be accomplished with either an external venting line or an implanted compliance chamber. External venting lines also break the skin barrier and are a second externalized access for infection. Implanted compliance chambers have problems with obstruction and breakage of the constantly moving membrane. Because of mechanical wear, the duration of support with the first-generation of pulsatile pumps was approximately 18 to 24 months, at which point the device had to be exchanged if further mechanical support was indicated.
The second generation of mechanical circulatory assist devices utilizes rotary blood pump technology to generate CF and are designed to provide more durable support. There are two types of CF: centrifugal or axial flow. The bearings of the CF rotating impellas are of three designs: blood-immersed, hydrodynamic, and magnetically levitated that generate minimal heat and are near frictionless. These newer pumps are smaller in size and lighter in weight compared to the first generation pulsatile pumps and allow for wider clinical availability (i.e., implantation in women and children). Due to the smaller size of the pump, surgical implantation is technically easier and infection risk is reduced with the smaller percutaneous driveline and the absence of air-displacement systems. The absence of internal valves with only a single internal moving component reduces mechanical wear of the device, with the current longest duration of CF support to date with the HeartMate II device (HM II, Thoratec Corporation, Pleasanton, CA) being over 6 years. These devices are near silent in operation with minimal vibration resulting in improved patient satisfaction. Examples of a pulsatile and a CF pump are depicted in Figure 18.1 and important differences described in Table 18.2.5 The components of the HM II LVAD are depicted in Figure 18.2.2 Table 18.3 summarizes the system components.
FIGURE 18.1 A smaller CF LVAD is shown in the lower left. A larger pulsatile LVAD is shown in the upper right
TABLE
18.2 Comparison of Pulsatile- and Continuous-Flow Ventricular Assist Devices
FIGURE 18.2 Components of a CF LVAD.
TABLE
18.3 MCS Devices, Components, and Configurations: System Requirements and Options
The SynCardia TAH, as seen in Figure 18.3, is the first FDA-approved TAH as a bridge to transplant, receiving its approval on October 15, 2004, following a 10-year clinical study. There have been more than 900 implants worldwide of the TAH. This device is indicated for eligible patients waiting for heart transplant who require biventricular support. Patient selection is critical and must fulfill the following conditions: BSA ≥ 1.7 m2, Echo: LVEDD ≥ 70 mm, chest CT scan: anterior-posterior dimension at T-10 from posterior sternum to anterior spine ≥ 10 cm, and chest x-ray: cardiothoracic ratio ≥ 0.5.
FIGURE 18.3Components of the SynCardia Total Artificial Heart.
PATIENT SELECTION
Optimal patient selection for MCS in the treatment of advanced heart failure is of utmost clinical importance and is a topic that has repeatedly been tested on previous board examinations. All patients considered for MCS ideally need to undergo a thorough evaluation including assessment of the severity of their heart failure (New York Heart Association [NYHA] Class, ACC/AHA Stage, cardiopulmonary exercise stress testing, invasive hemodynamic studies, and echocardiography). Close evaluation is needed of other comorbidities, end-organ function, anatomic considerations, social support system, and psychological status.
Table 18.4 summarizes end-organ compromise that should raise concerns for MCS candidacy. Mancini et al.6 described the value of peak oxygen consumption in cardiopulmonary exercise stress testing in the timing of cardiac transplantation in outpatients with severe heart failure. This landmark study has generally been accepted by the ventricular assist device (VAD) community in considering MCS therapy in appropriate patients who have a peak oxygen consumption of <14 mL/kg/min who achieved an adequate anaerobic threshold. Similarly, although no strict hemodynamic or clinical criteria exist, potential MCS candidates generally have systolic blood pressures <90 mm Hg, pulmonary capillary wedge pressure >20 mm Hg, systemic vascular resistance >2,000 dynes, falling urine outputs despite diuretics (<20 mL/h in an adult), and a cardiac index of <2/L/min/m2 despite the use of inotropic agents, vasopressors, and occasionally IABP
TABLE
18.4 Challenges that may Compromise Outcomes
Because heart failure is a disease with a wide spectrum of symptoms and presentations, the Interagency Registry for Mechanically Assisted Circulatory Support (INTERMACS) profile was developed to help classify patients with advanced heart failure.7 The seven profiles are listed in Table 18.5. Although most (~60%) of patients recently implanted with MCS have been in INTERMACS profiles 1 and 2,8 emerging evidence suggests that in patients implanted with CF pumps, less acutely advanced heart failure patients have higher short- and long-term survival following LVAD implantation and shorter length of stays compared to patients who are more acutely ill.9 It is generally accepted that MCS is used to rescue patients with INTERMACS profiles 1 and 2 who are potential BTT candidates. There is now a shift to implant LVADs in inotrope-dependent, advanced heart failure patients with lower INTERMACS profiles. Studies are underway to further investigate their outcomes compared to those on chronic inotropic therapy.
TABLE
18.5 Intermacs Levels
The first set of formal recommendations regarding patient selection for mechanical support was described in the consensus statement from the Conference on the Current Applications and Future Trial Design of the Mechanical Cardiac Support in 2000.10 A set of guidelines still utilized was published by the International Society for Heart and Lung Transplantation (ISHLT) in 2006.11 Due to the varied experiences in the use of MCS as well as the many types of available devices, a single set of guidelines applicable to all patients is not possible. However, the discussion below highlights the most important general considerations in selecting appropriate candidates for MCS for end-stage heart failure.
Age
Age alone should not be a contraindication to MCS. In general, patients over the age of 60 have more comorbidities than younger patients with an inverse survival relationship, but acceptable outcomes have been reported in multiple studies in select patients ≤70 years of age.12–14 Although policies vary by institution, if a durable assist device is implanted in a patient >70 years old, they are generally for patients with the intention of DT or for select patients as a bridge-to-transplant.
Body Habitus
Because of the size of the larger, first-generation pulsatile intracorporeal LVADs, it was recommended that these devices should not be implanted in patients with a body surface area of <1.5 m2 to ensure adequate thoracic and abdominal capacity to accommodate the size of the device. These devices are rarely used in the current era of MCS. The new generation of CF pumps allows a larger group of individuals to benefit from MCS. Individuals whose body surface area is >2.5 m2 or with an ideal body weight of >150% may pose technical challenges intraoperatively. Currently, a BMI of >35 would not permit a patient to be a transplant candidate, although a device may be placed as a bridge to candidacy pending appropriate weight loss. There is limited experience regarding concurrent weight loss surgery to allow patients to then become transplant eligible, but a topic that we will hear more about in the future.
Renal Function
There have been multiple studies showing improvement of renal function following implantation of both pulsatile and CF devices in patients without intrinsic kidney disease.15–18However, patients with preoperative creatinine ≥3.0 mg/dL have worse outcomes following implantation.19 Patients with creatinine values in this range may still be considered for MCS if renal injury is acute and is likely to be reversible. Patients with end-stage renal disease on dialysis are not candidates for MCS. There have been cases of patients being supported with VAD therapy that have progressive renal disease and require renal replacement therapy. This poses a much higher risk of morbidity and mortality, primarily due to infection.
Pulmonary Function
Patients with severe underlying lung disease (baseline FEV1 <1.0) and requiring continuous oxygen supplementation are not appropriate candidates for MCS, as they often have difficulty weaning from postoperative mechanical ventilation, are prone to pulmonary infections, and may continue to be dyspneic once their cardiac status has stabilized. Potential candidates for MCS require formal pulmonary function tests, appropriate imaging studies, and pulmonary evaluation. Patients with mild-moderate lung disease may be considered for MCS.
Hepatic Function
Patients with cirrhosis and liver transaminases ≥ 3x the normal limit are at higher risk for morbidity. If known RV dysfunction is present with associated liver dysfunction, then the need for biventricular support should be considered in patients otherwise being considered for LVAD implantation. Patients with intrinsic cirrhosis or portal hypertension should not be considered candidates for MCS. In certain cases, a liver biopsy and hepatology evaluation may be useful in differentiating primary cirrhosis from secondary congestive hepatopathy. Patients with viral hepatitis are not a contraindication to MCS.
Coagulation Abnormalities
Intra-and perioperative bleeding is a significant risk factor for morbidity and mortality following MCS implantation, and the identification of preoperative coagulation abnormalities is essential. Patients with a spontaneous international normalizing ratio (INR) >2.5 off any anticoagulation are at higher risk and generally not candidates for MCS. In addition, patients with perioperative heparin-induced thrombocytopenia (HIT) are generally not considered candidates, but if MCS is considered, alternative methods of anticoagulation such as direct thrombin inhibitors should be used.
Infectious Concerns
Sepsis is one of the most common causes of morbidity and mortality following MCS implantation. If an infectious etiology is discovered, aggressive anti-infective therapy should be administered and implantation, if possible, be delayed until the infection is cleared. In fact, a white blood cell count of >10,000/µ,L has been found to be a risk factor of post-implant mortality.12 Patients considered for MCS therapy are more prone to develop fungal infections that are particularly difficult to eradicate.20,21 If a device gets implanted while a patient is actively infected, the offending organism can colonize the hardware and will be unable to be eradicated, even with long-term intravenous anti-infective therapy.
Arrhythmias
Unless there is a primary underlying proarrhythmic etiology, such as giant cell myocarditis, ventricular arrhythmias often resolve following LVAD implantation, since wall stress is reduced and the LV is unloaded. LVADs, in the presence of normal pulmonary vascular resistance (PVR), essentially produce a Fontan-like circulation allowing the RV to fill the pump despite even the most malignant dysrhythmias and is tolerated well clinically in MCS-supported patients.22 At this time, pacemakers and implantable cardioverter defibrillators (ICDs) maintain their usual indications in MCS patients, although little has been studied in this particular field.
Pulmonary Hypertension
Elevated pulmonary pressures are frequently seen in patients with advanced heart failure. This may be due to chronic congestion, severe mitral insufficiency, and elevated pulmonary capillary wedge pressure leading to remodeling of the pulmonary vasculature. Careful consideration must be made to identify whether the patient has pulmonary venous hypertension, pulmonary arterial hypertension, or a combination of both with a transpulmonary gradient >15, PVR > 3 WU, and an elevated pulmonary capillary wedge pressure. In some instances, an LVAD will be placed as DT, since the elevated PVR precludes listing for transplant. With medical therapy and unloading of the ventricle with the LVAD, there may be improvement in PVR and allow listing for transplant.
Right Ventricular Function
The evaluation of RV function deserves special attention in patients being considered for LVAD, especially in patients implanted with a second-generation CF device. RV failure following LVAD implant is an important cause of morbidity and mortality for patients that often require prolonged inotropic therapy or ultimately placement of a RVAD. There have been a number of risk scores devised in an attempt to better predict which patients may develop RV failure after LVAD implantation, to allow for better medical optimization prior to the LVAD implant or for planned simultaneous biventricular support. Fitzpatrick et al. performed a retrospective analysis from 266 patients who underwent LVAD implantation at a single center from 1995 to 2007 and found that the multivariate risk factors predicting the need for biventricular mechanical support were cardiac index ≤2.2 L/min/m2, RV stroke work index ≤0.25 mm Hg × L/m2, severe preoperative RV dysfunction, preoperative creatinine ≥1.9 mg/dL, previous cardiac surgery, and systolic blood pressure <96 mm Hg.23 Multiple types of LVADs, the majority of which were pulsatile devices, were reviewed in that study. A recent review of patients implanted with a CF LVAD enrolled in the HM II LVAD BTT clinical trial showed that multivariate independent predictors of RV failure following LVAD implant included a central venous pressure/pulmonary capillary wedge pressure ratio >0.63, need for preoperative ventilator support, and blood urea nitrogen level >39 mg/dL.24 The difficulty in consistently predicting RV failure lies, in part, on the unmasking of native RV dysfunction in the presence of restored cardiac output and increased venous return following LVAD implantation. It is estimated that approximately 20% to 25% of patients undergoing LVAD implant develop RV failure.25 This continues to be an area of active investigation.
Anatomic Considerations
Perhaps one of the most important anatomic considerations prior to LVAD implantation is the competency of the aortic valve. Following LVAD implantation, the LV is unloaded and pressure rises in the aortic root with output from the device. Pathologic specimens either at time of transplant or autopsy may show commissural fusion of aortic leaflets leading to a central jet of aortic insufficiency. Mild to moderate aortic regurgitation prior to implant may progress to more severe grades of aortic regurgitation and worsening heart failure. This situation is best avoided by preemptively repairing at least moderate grades of aortic insufficiency at the time of LVAD implantation. Other important considerations include repairing severe mitral and tricuspid insufficiency and converting mechanical aortic and mitral valves to bioprosthetic valves at the time of VAD implant. There is increased risk of thrombosis of the mitral valve due to a lower INR goal and in the aortic valve that may remain in the closed position due to VAD speed and lack of native contractility. Interatrial septal abnormalities such as a patent foramen ovale and atrial septal defect are generally closed at the time of surgery. Complex congenital heart anatomy and hypertrophic cardiomyopathy may present surgical challenges that preclude LVAD implantation.
Nutritional Status
Cardiac cachexia (BMI <21 kg/m2 in males and <19 kg/m2 in females) is a strong predictor of postoperative morbidity and mortality.26 Prealbumin and albumin levels should be measured in potential MCS candidates and optimized prior to surgery. Reports of total parenteral nutrition (TPN) to boost nutritional status and improve postoperative outcomes remain anecdotal at this point in time and are not routinely recommended.
Neurologic Function
In patients presenting in acute cardiogenic shock who are supported by mechanical ventilation at the time of initial evaluation for MCS candidacy, it is often difficult to ascertain the patient’s residual neurologic function or to predict full neurologic recovery. This is especially true if any period of anoxic brain injury was sustained as a result of a cardiac arrest. In such cases, a temporary mechanical support device should be employed to allow sufficient time to reassess neurologic function and appropriateness for more durable types of mechanical support. In the advanced heart failure population, low cardiac output states may contribute to confusion or suspected dementia, and then a full neurologic exam, CT scan of the brain, and neuropsychiatric testing are warranted.
Ideally, all age-related, gender-specific, health maintenance exams should be performed prior to consideration of MCS. If any malignancy is discovered, further workup should be pursued. Some patients with potentially curable tumors may undergo MCS implantation, as a means to prolong life to undergo further workup and treatment to become eligible for transplantation, or as DT. Patients with metastatic cancers are not candidates for MCS.
Psychiatric Conditions
Successful MCS therapy requires patient compliance and attention to the maintenance of the pump with changing batteries, keeping the driveline exit site clean, appropriate response to pump alarms, and close follow-up in the clinic setting. The presence of any psychiatric conditions hindering the patient’s ability to carry out his/her responsibility caring for the device should raise concerns regarding the patient’s candidacy for MCS. Such conditions may include substance abuse/addiction, severe depression, severe anxiety, uncontrolled schizophrenia, and personality disorders that may lead to lack of compliance with care.
Social Evaluation
A thorough evaluation should be undertaken with the assistance of a social worker to ensure that a candidate’s support system of family and/or friends as well as the home environment is suitable and conducive to the candidate’s success in adjusting back to daily life with MCS. Such a support system is also essential to provide meticulous wound care once the patient is discharged to home and to be present in case emergencies arise, such as pump malfunction. Despite being an optimal candidate from a medical standpoint, the absence of an adequate social network may preclude the use of MCS in certain patients.
ASSESSMENT OF OPERATIVE RISK
Included in a patient’s candidacy for MCS involves a detailed assessment of operative risk. There have been several studies looking at composite risk scores for postoperative mortality in BTT patients.17,27 The largest study to date is the Destination Therapy Risk Score that estimated 90-day in-hospital, post-LVAD implantation, mortality by Lietz et al.28 They found that the most important determinants of 90-day, post-LVAD mortality were poor nutritional status, hematologic abnormalities, markers of end-organ dysfunction, right ventricular dysfunction, and lack of inotropic support. In particular, in the order of the most weighted risk factor, a platelet count ≤148 × 103/µL, serum albumin ≤ 3.3 g/dL, INR > 1.1, use of vasodilator therapy, mean pulmonary artery pressure ≤25 mm Hg, aspartate aminotransferase > 45 U/mL, hematocrit ≥34%, blood urea nitrogen >51 U/dL, and lack of intravenous inotrope use were all multivariate risk factors for 90-day in-hospital mortality following LVAD implantation. It should be noted that these studies evaluated patients implanted with the pulsatile LVADs, although their applicabilities to those patients considered for CF LVAD implantation are likely to be similar and studies are currently under way to investigate this question.
OUTCOMES AFTER CIRCULATORY SUPPORT IMPLANTATION
Infection and bleeding were two of the most common adverse events seen post VAD implant in the 2005 Registry from the International Society for Heart and Lung Transplantation Mechanical Circulatory Support Device (ISHLT-MCSD).12 Table 18.6 summarizes the postimplantation patient-related events. Infection was seen in approximately one-third of patients with almost 30% having significant bleeding episodes. Neurologic dysfunction was observed in 14% of the registry patients.
TABLE
18.6 ISHLT MCSD 2005 Registry Analysis (N = 655): Postimplantation Patient-Related Events
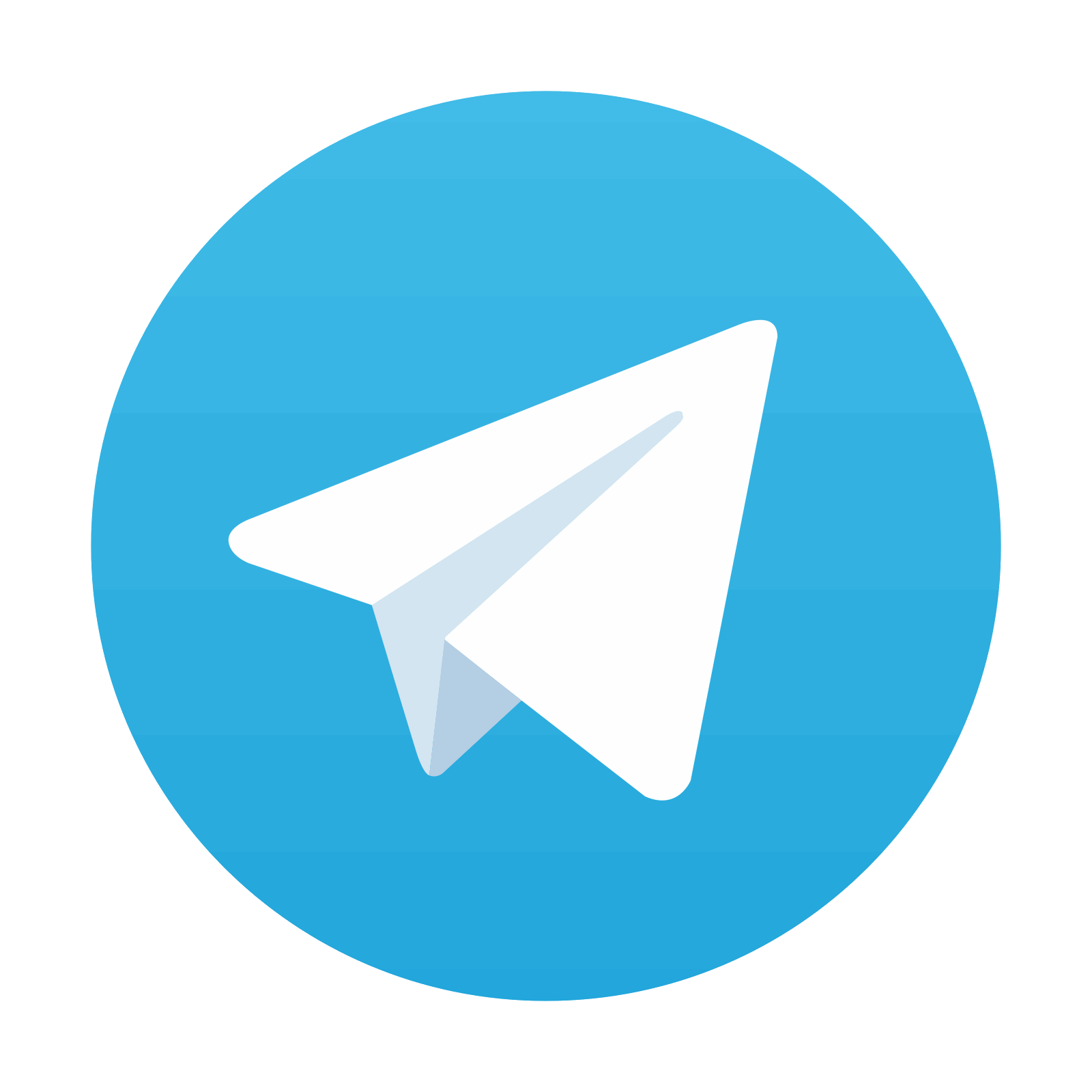