Summary
Echocardiography is a very important tool for the diagnosis and follow-up of children with congenital and acquired heart disease. One of the challenges that remains in paediatric heart disease is the assessment of systolic and diastolic function in children, as this is influenced by growth, morphology and loading conditions. New echocardiographic techniques, such as tissue Doppler, deformation imaging and three-dimensional echocardiography, have great potential application in this field. They may provide new insights into the influence of growth, morphology and loading on cardiac mechanics, and could become useful clinical tools. In this review, we discuss the potential use and limitations of these new echocardiographic techniques in paediatric and congenital heart disease.
Résumé
L’échocardiographie est un outil très important dans le diagnostic et le suivi des patients avec cardiopathies congénitales et acquises. L’évaluation de la fonction systolique et diastolique chez l’enfant reste un problème due aux influences liées à la croissance, la morphologie ventriculaire et les conditions de charge. Les nouvelles techniques échographiques comme le Doppler tissulaire, l’imagerie de déformation et l’échographie 3D ont des applications potentielles importantes dans ce cadre. Elles peuvent apporter des nouvelles informations concernant la mécanique cardiaque dans différentes conditions pendant la croissance. Dans cette revue, on présente les indications potentielles et les limitations de ces techniques échographiques chez l’enfant.
Introduction
Echocardiography has become the most important noninvasive technique for the diagnosis and follow-up of heart disease in children. Cross-sectional Doppler echocardiography allows a detailed description of cardiac anatomy and haemodynamics. Currently, the majority of children are referred for cardiac surgery based on echocardiography only. The diagnostic accuracy for describing cardiac morphology is extremely high, with a reported incidence of diagnostic errors of only 87 errors in more than 50,660 echocardiograms in an established paediatric echocardiography laboratory , demonstrating the level of accuracy that can be reached.
Cardiac MRI and cardiac computed tomography are the main imaging techniques used for extracardiac anatomy (pulmonary arteries and aortic arch) if not visualized properly by echocardiography. Diagnostic cardiac catheterization has become more obsolete and is restricted mainly to haemodynamic assessment in more complex lesions and the assessment of pulmonary vascular resistance. One of the challenges remaining for paediatric echocardiography is the availability of good techniques for assessing systolic and diastolic ventricular function. Most functional variables used in echocardiography were developed for the assessment of the morphologically normal LV. The diversity of congenital heart defects complicates the interpretation of functional variables, because of the anatomical variability, the effect of growth on myocardial function and the differences in loading conditions. For the LV, adult techniques are extrapolated to paediatrics often without good validation. For the RV and the single ventricle, qualitative subjective assessment is the technique used routinely in most laboratories.
During the past decade, different echocardiographic techniques have been developed that allow a more detailed analysis of cardiac function. These techniques have potential application in and substantial benefit for the assessment of ventricular dysfunction in paediatric patients. TD echocardiography and speckle-tracking-based strain imaging provide direct quantitative information about myocardial motion and deformation, which is more geometry independent than measurement of EF; they give more insight into myocardial mechanics and could provide guidance in treatment and response to therapies. Current 3D echocardiographic techniques enable the acquisition of full volumetric datasets, which can be analysed offline for the calculation of ventricular volumes, mass and EF. The impact of these technologies on paediatric functional echocardiography will be discussed in the current review.
Tissue Doppler velocities
The analysis of pulsed TD signals to interpret cardiac motion has been used since the early 1960s . It took until the 1990s for it to be recognized as a potentially useful clinical technique for the assessment of global and regional myocardial function . In a pig ischaemic model, tissue velocities were shown to change very quickly and consistently after the induction of ischaemia . Several subsequent clinical studies investigated the use of regional myocardial velocities in various adult diseases, such as ischaemic heart disease, aortic insufficiency and hypertrophic cardiomyopathy . The advantage for paediatric and congenital heart disease is that these techniques are geometry independent and can be applied to any chamber morphology.
Colour TD imaging was introduced in the early 1990s as an alternative technique for measuring tissue velocities. In contrast to pulsed Doppler, which measures peak velocities, it uses autocorrelation techniques to measure regional mean velocities. This technical difference explains why colour TD-derived myocardial velocities are on average 15–20% lower than pulsed wave-derived myocardial velocities ( Fig. 1 A and B ). Very high frame rates can be obtained by image optimization (> 250 frames/second), which is very useful for the analysis of short-lived myocardial movements, such as during the isovolumetric periods, and is important for adequate temporal resolution at higher heart rates. An advantage of colour TD is that tissue velocities can be recorded simultaneously in different myocardial segments during the same cardiac cycle. This allows the comparison of regional wall motion and timing of cardiac events between different myocardial segments during the same cardiac cycle, which is important for dyssynchrony evaluation.

The limitations of TD velocity imaging are related to its angle dependency (Doppler technique) and the unidimensional assessment of myocardial motion (longitudinal, circumferential or radial). Global cardiac translation of the entire heart during the cardiac cycle will also affect the measurement and tethering effects between myocardial segments; if a dysfunctional segment is moved by a healthy segment, this can also contribute to regional motion masking regional dysfunction.
Clinical application of tissue velocities in children
One of the challenges when introducing new techniques into paediatric echocardiography is that the methodology must be validated first for paediatric use, especially the establishment of normal values for different paediatric age groups. Normal paediatric TD data have been published by different groups . In these studies, it was shown that tissue velocities vary with age and heart rate. Eidem et al. included 325 children and showed that pulsed-wave TD velocities also correlate with cardiac growth variables, especially the LV end-diastolic dimension and LV mass , indicating that tissue velocities are not entirely geometry independent. This has important implications when applying this methodology to children with congenital heart disease, where there is a large variability in ventricular geometry. Apart from the influence of geometry, changes in loading conditions also affect TD velocity measurements. Acute preload changes clearly affect tissue velocities , while this is less clear for the effect of chronic volume loading, where the ventricle has adjusted to the chronic load . Studies in children with chronic LV volume loading related to a ventricular septal defect or patent ductus arteriosus have documented only minimal changes in TD velocities compared with normal paediatric controls . Acute increase in afterload results in decreased TD velocities , while chronic adaptation by the concentric hypertrophic response results in decreased longitudinal velocities during the remodelling process. In children with aortic valve stenosis, systolic longitudinal velocities were shown to be reduced in the basal segments of the interventricular septum and the LV lateral wall. This could be an effect of hypertrophy and associated subendocardial dysfunction. In an adult population with aortic stenosis, the degree of reduction in longitudinal function was shown to be related to the degree of fibrosis in the LV and was also predictive of outcome after aortic valve replacement . Kiraly et al. showed that in children with aortic valve stenosis, longitudinal TD velocities were reduced more significantly compared with radial velocities. More research is warranted in children, as the mechanisms for adaptation to increased pressure loading could be different in the paediatric population.
As congenital heart disease frequently affects the RV, data on right ventricular TD velocities have been published in different conditions that affect the RV. With the predominantly longitudinal orientation of the RV myofibres, the quantification of longitudinal function is especially important when assessing RV function. Good correlations between systolic velocities and RV EF were found in an adult population that included patients with congenital heart disease . Several studies have shown elevated RV systolic velocities in patients with ASDs before closure of the defect, which normalized within 24 hours after closure . Quantitative assessment of right ventricular performance after repair of tetralogy of Fallot has also been the subject of considerable investigation. TD velocities are decreased in tetralogy patients after repair, with some RV regional wall motion abnormalities . However, in these patients, regional functional variables obtained at the base of the RV free wall correlate less well with RV EF assessed by MRI, as this includes the patched and often dilated right ventricular outflow tract, influencing the assessment of global RV function. Hence, the use of regional variables in this condition is still unproven and requires further study.
The use of TD velocities in functionally univentricular hearts has also been studied. Frommelt et al. used serial measurements for the evaluation of patients with hypoplastic left heart syndrome from the neonatal period until after the second stage palliation, and showed a trend towards a decrease in systolic and diastolic tissue velocities, with no difference regarding the type of initial palliation. However, the interpretation of these data is difficult, as there were significant changes in loading conditions and also changes in ventricular growth that could have influenced the observed changes. Vitarelli et al. evaluated ventricular function at midterm (7.4 ± 2.8 years) after Fontan completion, using myocardial velocities derived from the computed tomography dose index. They compared morphologically LVs (tricuspid atresia, double inlet LV) with normal controls and analysed the anterior and inferior wall (groups more comparable). There were significantly lower systolic and early diastolic tissue velocities in the Fontan group, which correlated well with the EF and the mass/volume ratio, respectively.
Tissue velocities are used in the evaluation of mechanical dyssynchrony. Mechanical dyssynchrony, as assessed by TD imaging and speckle tracking, is often present in patients with cardiomyopathy unrelated to electrical dyssynchrony, and correlates with the severity of LV dysfunction . This probably reflects regional differences in myocardial dysfunction. Mechanical dyssynchrony by TD imaging was analysed in only 64% of patients in a multicentre European study evaluating the current practice and results of cardiac resynchronization therapy in paediatric and congenital heart disease .
Overall, the effect of growth and variability in loading conditions on myocardial velocities limits their use in paediatric heart disease, except for serial follow-up in the same patient. However, only limited data are available. For example, after heart transplantation in children, a significant decrease in systolic and diastolic RV tissue velocities has been noted 3–6 months before terminal graft failure .
Tissue velocity tracings have also been used to calculate myocardial performance index, with good correlations with standard pulse Doppler measurements . The use of TD imaging tracings is especially useful for the RV, allowing simultaneous measurement of systolic and diastolic events. It has been described as being useful in the assessment of patients after repair of tetralogy of Fallot and other congenital heart diseases . An animal study by Cheung et al., using invasive measurements, demonstrated that the myocardial performance index was affected significantly by acute changes in loading conditions and was unable to detect acute changes in contractile function consistently , making its interpretation difficult in clinical settings. Additionally, we believe that if you combine systolic and diastolic time intervals in a single index, you do not know how to interpret the index when it is abnormal or when it changes; when it is abnormal, you can only conclude that something is wrong with either the systolic or diastolic function or that the loading of the heart changed. We do not use it routinely in our laboratory because of these considerations.
To overcome the effect of loading conditions on the measurements, myocardial acceleration during IVA was proposed as an index of myocardial contractility. IVA calculates the average rate of myocardial acceleration during the IVA period, which makes it insensitive to afterload changes and also, to some extent, to preload changes. In experimental studies, IVA has been validated as a sensitive noninvasive index of RV and LV contractility, which is unaffected by preload and afterload within a physiological range . It requires imaging at very high frame rates, as IVA is a short-lived event (30–40 ms). The disadvantage of IVA is that it is extremely sensitive to heart rate due to the force–frequency relationship. The heart rate sensitivity has been used to its advantage, to assess contractile reserve by studying the force–frequency relationship during pacing, dobutamine stress echocardiography or exercise . However, intra- and interobserver variabilities have been shown to be problematic. The reproducibility of LV IVA was demonstrated to be relatively poor, especially in patients with impaired LV function . Moreover, Lyseggen et al. showed that LV IVA is not a good variable for assessing regional myocardial function and, with non-physiological preload changes, is also preload sensitive. In paediatric patients, LV IVA was used after heart transplantation, where it was shown to be a useful noninvasive marker of allograft rejection .
The clinical use of RV IVA seems more promising. In patients with repaired tetralogy of Fallot, RV IVA was shown to be reduced and was related to the degree of pulmonary insufficiency, suggesting reduced contractile RV function in patients with pulmonary regurgitation or, alternatively, that the increased loading also affects the RV IVA measurement. For patients with a systemic RV, systolic functional reserve was assessed by dobutamine stress echocardiography, and the change in IVA during dobutamine exposure was shown to correlate well with the change in the end-systolic pressure–volume relationship assessed by conductance catheter measurements . The force–frequency relationship was studied noninvasively using IVA in the perioperative period in patients after congenital heart surgery . There was a large variability in the postoperative response, with a preserved force–frequency relationship after ASD closure and significant reduction after the arterial switch operation. The same principle could be used during stress echocardiography, as demonstrated by Pauliks et al. . In single ventricles, the technique can be used to assess contractile reserve, and initial data suggest a preserved systolic reserve in children after the Fontan operation .
Deformation imaging: strain, strain rate
Myocardial velocities are influenced by global cardiac translational motion and myocardial tethering, which limits their use in the assessment of regional myocardial function. This limitation can be overcome by using regional myocardial deformation or strain imaging. Two different technologies are currently available for studying regional myocardial deformation. The first technique is based on TD imaging and is based on calculating myocardial velocity gradients. The second is based on tracking speckles on the grey-scale images in different frames throughout the cardiac cycle, and calculates the displacement of the speckles throughout the cardiac cycle. Two variables for cardiac deformation can be calculated ( Fig. 1 C and D). Regional strain rate is the rate of deformation (per second) and is calculated as the velocity difference between two segments of myocardium divided by the distance between them. In younger children, we use computational distances of 4–5 mm in the radial direction and 8–9 mm in the longitudinal direction. Regional strain represents the amount of deformation (%) or the fractional change in length, caused by an applied force, and is calculated by integrating the strain rate curve over time during the cardiac cycle.
Experimental studies suggest that deformation variables can be used as noninvasive indices of ventricular function. End-systolic strain measurements correlate well with EF measurements, while peak systolic strain rate correlates relatively well with dP/dt and end-systolic elastance .
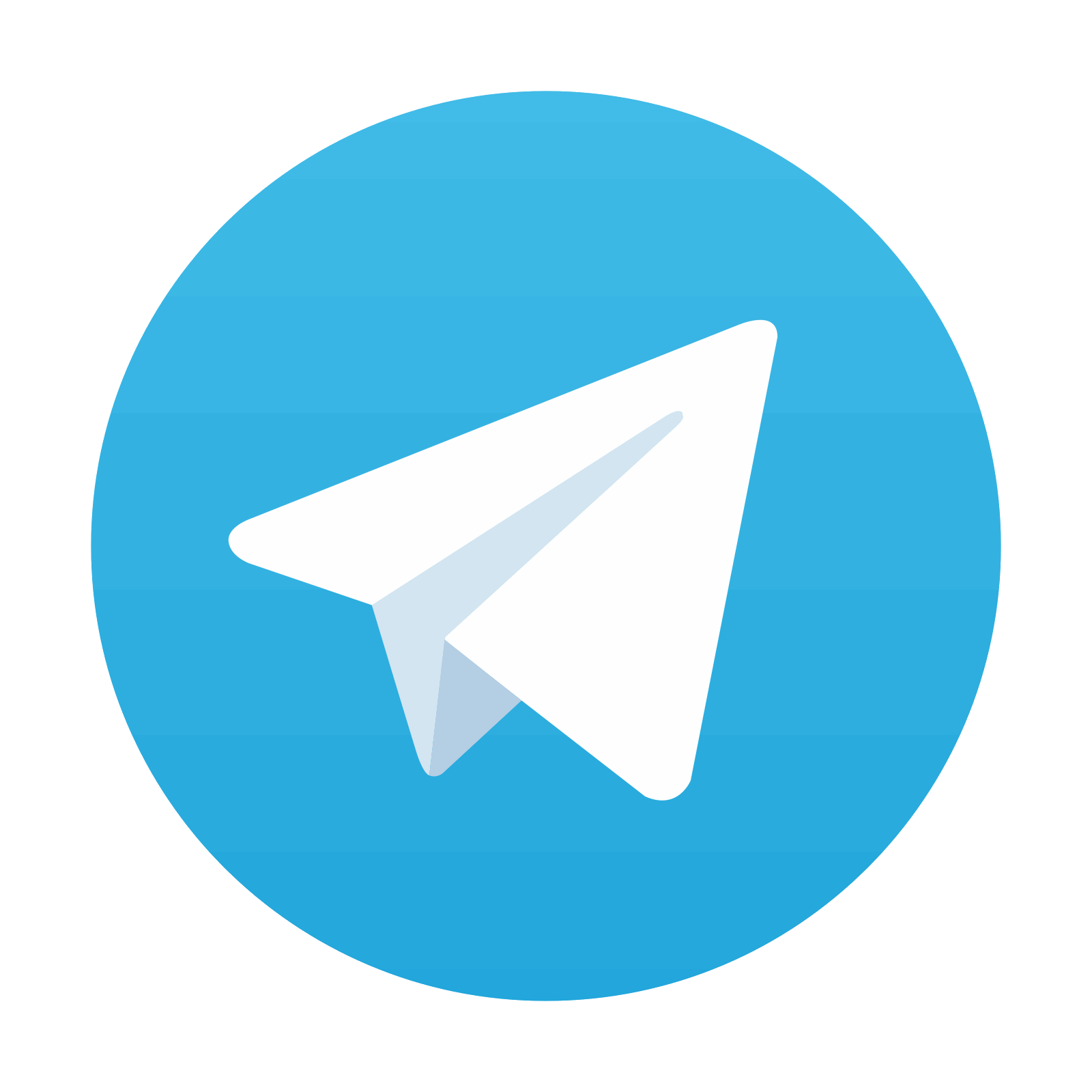
Stay updated, free articles. Join our Telegram channel

Full access? Get Clinical Tree
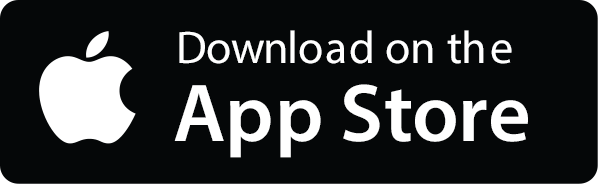
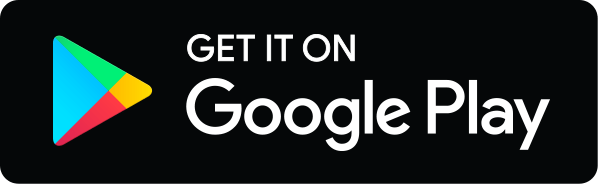