Fig. 9.1
Summary of stages of lung development from embryonic to postnatal life
Table 9.1
Sex differences at various lung developmental stages
Developmental stage | Main events | Airway structure development (branching status) | Sex differences |
---|---|---|---|
Embryonic (3–6 weeks) | Appearance of lung buds from foregut. Formation of trachea and bronchial buds. | Trachea Bronchi (2–6 generations) | – |
Pseudoglandular (6–16 weeks) | Branching of airway and blood vessels. Differentiation of epithelial cells. | Bronchioles Terminal bronchioles (6–16 generations) | Fetal growth and breathing movements detected earlier in females. |
Canalicular (16–26 weeks) | Subdivision of distal airways into canaliculi, vascularization. Differentiation of type I and type II cells, surfactant production. | Respiratory bronchioles (16–19 generations) | First functional differences appear. Surfactant secretion and phospholipid maturation are inhibited in males. |
Saccular (26–36 weeks) | Cell differentiation, type II cell maturation, surfactant secretion. Formation of sacs. Thinning of alveolar walls, formation of primary septa and acini. Extracellular matrix components and connective tissue decrease. | Alveolar ducts (19–22 generations) | Surfactant production and phospholipid profile remain more advanced in females. |
Alveolar (36 weeks—adolescence) | Alveolar multiplication (alveolarization). Alveolar enlargement and maturation. Lungs continue to grow through adolescence. Lung function (FEV1) increases with age and peaks in adolescence. | Alveolar sacs (22–23 generations) | Reduced risk for RDS and better response to surfactant therapy in girls. Faster alveolarization in females. Higher flow rate per lung volume, but smaller lung size in girls than boys. FEV1 peaks earlier in girls than boys. |
The first stage is the embryonic, which spans from 3 to 6 weeks postconception. In this phase, the lung starts developing at around day 26 of gestation as a ventral outpouching of the foregut (Fig. 9.1). This ventral diverticulum (lung bud) will start forming the trachea and the two bronchial buds that will give rise to the left and right bronchi. The asymmetry of the main bronchi is already established at this stage: the left bud is smaller and directed laterally and the larger right bud is directed more caudally [18]. Toward the end of the embryonic stage, the bronchial buds undergo subsequent divisions, resulting in the precursor structures of the pulmonary lobes, three on the right and two on the left (Fig. 9.1), and a small pulmonary circulatory system starts developing [19, 20]. At this point, no sex differences are observed in lung development.
The embryonic stage is followed by the pseudoglandular stage that extends from 6 to 16 weeks of gestation and that is characterized by bronchial development and airway branching. In this stage, the bronchial tree is developed up to the terminal bronchiole, and the respiratory parenchyma and respiratory ducts are formed, together with a primitive pulmonary circulatory system [21, 22]. Lung epithelial cells begin to differentiate into precursors for bronchial smooth muscle, cartilage, and submucosal glands in a process highly dependent on epithelial and mesenchymal interactions [21, 23]. The bronchial tree is initially coated by a cubic epithelium, which is the precursor of the ciliated epithelium, and by secretory cells and immature type II cells. In this stage, sex differences begin to appear in fetal mouth movement, an indication of fetal lung maturation, showing advanced breathing in females more than in males [24].
The next stage is the canalicular phase , starting at week 16, and extending until week 26 of gestation. In this period, the basic gas-exchanging airway units are formed by further subdivisions of the distal airways (Fig. 9.1). These canaliculi are formed by branching of the terminal bronchioles, and compose the now respiratory parenchyma. The groups of air spaces that are formed from a single respiratory bronchiole form an acinus. Vascularization of the peripheral mesenchymal tissue is the foundation for the later exchange of gases at these respiratory units. The lumen of these becomes wider and the epithelial type II cells begin to secrete surfactant, and differentiate into type I cells [22]. Capillaries move into close contact with the surface epithelium, and connective tissue is reduced. The first functional sex differences are noticed at this stage, and these are attributed to hormonal influences, as well as sex differences in gene expression. At around 16 weeks of gestation, the lungs of female fetuses are usually more mature than those of males, and begin to produce surfactant lipids [5, 10, 25, 26] (Fig. 9.1).
At the end of the canalicular phase, and the beginning of the saccular phase, spanning from weeks 26–36 postconception, a large part of the amniotic fluid is produced by the lung epithelium. At this stage, lung maturity can be measured clinically by quantifying lipid content in the amniotic fluid (ratio of lecithin to sphingomyelin), based on the production of surfactant by type II cells [27]. As indicated, female fetuses usually show a more mature phospholipid profile at this stage, which correlates with an earlier production of surfactant components [25].
During the saccular phase , drastic changes occur in the morphology of the developing lung. Further subdivisions at the terminal bronchioles give rise to clusters of sacs that will later become the alveoli (Fig. 9.1). These sacculi are internally coated with type I and type II cells and the parenchyma between them contains primary septa that are composed of networks of capillaries. These contain small protrusions that will later become alveoli via delimitation by secondary septa. At the end of the saccular phase, all generations of the conducting and respiratory branches are generated, and about 1/3 of the roughly 300 million alveoli are fully developed.
The saccular phase is characterized by type II cell maturation and surfactant production, which are promoted by paracrine communications with cells of the interstitial space. Pulmonary surfactant is a lipoprotein complex (90 % lipids, 10 % proteins), responsible for preventing alveolar collapse at low lung volumes. By decreasing surface tension at the alveolus, lung surfactant promotes gas exchange and lung mechanics. Pulmonary surfactant also enhances lung innate immunity by the actions of surfactant proteins A and D (collectins). Thus, in preterm infants born at less than 30 weeks of gestational age, surfactant deficiency greatly contributes to the development of RDS, and related morbidities such as BPD, patent ductus arteriosus, arterial pulmonary hypertension, and long-term neurological disabilities [12, 28, 29]. Surfactant synthesis and secretion significantly increase after week 30 in preparation for the transition to air breathing, and the male lung begins to synthesize surfactant later in gestation than the female lung (Fig. 9.1). This delay has been attributed to androgens and other factors, as described later in the chapter [6]. Therefore, female newborns have increased airflow rates, but lower airway resistance compared with male newborns, and they are less likely to develop RDS [11, 30].
Lastly, at the postnatal alveolar stage , starting at birth (36 weeks postconception) and extending throughout adolescence, the number of alveoli largely increases [1]. This alveolar multiplication process (alveolarization) is very active within the first 2 years of life, although the alveolar size and number of alveoli per unit area does not change much during this period. The respiratory epithelium differentiates into a variety of cell types including ciliated, goblet, basal, and Clara cells [31]. Although maturation appears to be more advanced in female fetuses than in male fetuses, girls on average have smaller lungs and fewer respiratory bronchioles than boys at birth. Female neonates also have higher size-corrected flow rates, specific airway conductance, and a higher ratio of large airways to small airways than males, and it has been suggested that boys have more respiratory bronchioles per lung volume than girls [2, 32]. Furthermore, the growth of airways is slower than the growth of lung parenchyma in boys, whereas in girls the growth of airways is proportional to the growth of parenchyma. This “dysynaptic” growth originates in early childhood, and remains constant during growth [1, 33]. Boys in general have bigger lungs than girls of the same stature, and studies have suggested that boys have more respiratory bronchioles than girls, but no sex differences have been reported in alveolar dimensions, and in number of alveoli per unit area. In terms of lung function, studies in teenagers showed that growth in forced expiratory volume in 1 s (FEV1) increases with age, reaching a peak at ages 12–13 in girls and at age 14 in boys [34, 35]. The FEV1 was shown to increase until the age of 17 in both sexes, but the continued growth in lung function after attainment of full height is higher in boys than in girls [36]. Finally, in adult life, sex differences have also been documented in lung structure and morphology [8, 33, 37, 38]. Height-matched men have larger diameter airways, total number of alveoli, and larger lung volumes and diffusion surfaces compared with women. However, for a given lung size, women have larger airways size than men [38].
In summary, given the gender effects on lung structure and function during lung development (Table 9.1), males are at higher risk for newborn and early childhood lung complications. In postnatal and adult life, however, the lower lung capacity in females, and the influence of sex hormones, makes women more prone to the damaging effects of air pollution and pulmonary limitations during exercise [39, 40]. Additionally, by modulating circulating levels of estradiol and progesterone, the menstrual cycle can also affect pulmonary function and inflammatory lung disease outcomes in women [41–43].
Hormonal Influences During Lung Development
The developing fetal lung is exposed to circulating levels of sex hormones synthesized from both maternal and fetal adrenal steroid precursors, by the action of specific lung steroidogenic enzymes [44, 45]. As indicated above, various regulatory roles have been attributed to the effects of sex hormones throughout fetal and postnatal lung development, and associated with sex differences in lung maturation. In the stages preceding the surge of surfactant synthesis, the developing male lung is exposed to higher levels of testosterone than the female lung. In the late gestational stages, both sexes are exposed to increasing levels of estradiol synthesized by the human placenta [5]. Below we describe the main known effects of male and female hormones in fetal and postnatal lung development. These are also summarized in Table 9.2.
Table 9.2
Hormonal secretion and regulatory effects in lung development
Hormone | Expression and source | Functional Effects | Proposed mechanisms of sex differences | Genes regulated |
---|---|---|---|---|
Estradiol | Week 20, in males and females. Significant increase toward late development. From human placenta. | Enhances surfactant synthesis and lung maturation. | ER expression patterns and signaling in females. | VEGF PDGF |
Testosterone | Week 9, in males. From testis. | Delays lung development Impairs fibroblast– type II cell communications. | TGFβ and EGF receptor signaling in males. AR expression patterns and DNA binding in males. Modulation of steroidogenic enzymes in males. | TGFβ EGF FGF Shh BMP Wnt |
AMH | Week 8.5, in males. From testis. | Delays airway branching. | Enhancement of apoptosis in males. | – |
Female Hormones
Fetal estradiol levels appear at the beginning of the canalicular phase, at approximately week 20 of female lung development, and continue to increase throughout birth [5]. Estrogens have been found to be responsible for the sexual dimorphism observed in lung development, for alveoli formation, and for lung maintenance and regeneration [17, 46–48]. Moreover, regulation of surfactant synthesis is positively affected by estrogens, favoring lung development and maturation in the female lung [11, 49]. Sex differences observed in the expression pattern of estrogen receptors (ER) in the bronchial and alveolar epithelium suggest that these mediate signaling of estrogens, contributing to the differential pulmonary phenotype observed in males and females [48, 50, 51].
Male Hormones
In the male lung, fetal circulatory testosterone levels of testicular origin can be found as early as week 9, at the beginning of the pseudoglandular phase [5]. High levels of androgens in males have been associated with delays in fetal lung maturation [52–54]. In animal studies, maternal treatment with the androgen dihydrotestosterone (DHT) during fetal lung development inhibits surfactant phospholipid production in the female fetal lung, whereas treatment with the antiandrogen flutamide increases surfactant phospholipid production in the male fetal lung [55, 56]. Moreover, studies have shown that androgens act on the fibroblast to delay the development communications between these and type II cells, as well as surfactant production, by a mechanism involving transforming growth factor beta (TGFβ) and epidermal growth factor (EGF) receptor signaling pathways [55, 57].
The delay in the development of male fetal lungs and its response to androgens is also dependent on the expression of functional androgen receptors (AR), and by local modulation of androgen levels by steroidogenic enzymes. These are 5α-reductase (which converts testosterone into its active form DHT), 3α-hydroxysteroid dehydrogenase (which inactivates DHT into 5α-androstane-3α,17β-diol), and 17β-hydroxysteroid dehydrogenases (responsible for conversion of androstenedione into testosterone, estrone into estradiol, and dehydroepiandrosterone into androstenediol) [52, 53, 58–61].
Androgen receptors are expressed in both male and female lung epithelial cells, and they can bind to DNA sequences and regulate the expression of genes that may be involved in the control of lung cell differentiation [62]. In addition, in utero male fetuses are exposed to higher levels of anti-Müllerian hormone (AMH) than females. Anti-Müllerian hormone is a glycoprotein hormone that is detected at approximately 8.5 weeks and continues to be expressed throughout male lung development [63]. Studies showed that AMH is required for normal male reproductive tract development and delays airway branching processes in males by enhancing apoptosis [64, 65].
Glucocorticoids
Another class of steroid hormones known to affect lung development and maturation via regulation of morphological changes and surfactant synthesis are glucocorticoids (GC). The fetal lung expresses most of the genes necessary for GC local synthesis, including the enzymes 21-hydroxylase (responsible for conversion of progesterone into deoxycorticosterone) and 20α-hydroxysteroid dehydrogenase (a steroid-inactivating enzyme), and these appear to modulate lung ontogenesis through paracrine actions [66, 67].
In the developing lung, GC induce structural changes such as thinning of alveolar walls increasing lung gas volume, and stimulation of epithelial–fibroblast cell communications and type II cell differentiation [67, 68]. Glucocorticoid regulation of surfactant protein gene expression has been extensively studied and demonstrated at the transcriptional and posttranscriptional levels [69–75]. Studies in mice lacking the GC receptor showed that these fail to progress through the canalicular and saccular phases of development, and they die of respiratory failure shortly after birth, indicating that GC signaling is essential for lung development progression [76]. In addition, GC effects on lung development have been shown to be inhibited by androgens, indicating that these effects are different in males than in females [67]. In the clinic, antenatal GC is commonly administered to mothers at risk of premature delivery to reduce the risk of RDS, and sex differences have been reported in the efficiency of these treatments [77–79]. In some cases, prenatal exposure to GC has also been linked to the development of cardiovascular, metabolic, and neuroendocrine disorders, but no sex differences have been reported for these adverse events [79–81].
Gene Expression During Lung Development
During fetal lung development progression, several genes related to cell maturation, cell proliferation, surfactant protein and phospholipid synthesis, and androgen metabolism enzymes are expressed with a sexual dimorphism at different time points [59, 82]. Additionally, numerous genes are transcriptionally modulated by sex hormones in the developing lung (Table 9.2). Androgens have been shown to regulate the expression of genes related to TGFβ, EGF, fibroblast growth factor (FGF), sonic hedgehog (Shh), bone morphogenetic protein (BMP), wingless-related family (Wnt), and other signaling pathways [55]. These play important roles at different stages of lung development : TGFβ and EGF signaling are associated with inhibition of endodermal morphogenesis and cell proliferation [55, 57], FGF is mainly involved in cell proliferation, and BMP is associated with the regulation of epithelial–mesenchymal interactions and branching morphogenesis. Similarly, Shh is known to positively affect lung development during the embryonic and pseudoglandular stages, and Wnt mainly controls cell differentiation [83, 84]. Later in gestation, differential gene expression in males and females has been described for Kruppel-like factor 5 (known to regulate surfactant metabolism), glutathione peroxidase (an antioxidant enzyme), epidermal nitric oxide synthase (related to postnatal adaptation of pulmonary circulation), and Clara cell protein 16 (a major immunomodulatory protein associated with prematurity) [85–88]. Other genes differentially expressed at later stages of the developing lung are vascular-endothelial growth factor (VEGF) , and platelet-derived growth factor (PDGF) . The expression of these genes has been reported to be higher in the lungs of females than males, and regulated by estrogens via modulation of the ER signaling pathways.
Recent studies have also shown that epigenetic changes are also implicated in the differential expression of genes and gene networks during lung development. Epigenetic changes such as gene methylation and histone acetylation can affect the developmental timing and level of transcription of numerous genes expressed throughout development, particularly those involved in alveolarization , such as insulin-like growth factor-1 (IGF1) and PPARγ (peroxisome proliferator-activated receptor gamma) [89]. In addition, epigenetic marks imposed by exposure to nicotine or other insults during pregnancy can become permanently programmed and transferred to subsequent generations [90, 91]. MicroRNAs , a recently discovered class of noncoding RNAs that control gene expression, have also been implicated in the epigenetic regulation of lung morphogenesis and maturation [92]. In a recent study, sex differences were found in the expression of microRNAs at the canalicular and saccular stages, and associated with developmental gene expression of pathways involving retinoids, IGF1 receptor, VEGF, tumor protein p53 signaling, as well as mediators of glucose metabolism. Together these studies suggest that sex-associated differential epigenetic regulation may affect the differences observed in structural and functional lung development in males and females [93].
Environmental Exposures and Lung Development
Environmental exposures can dramatically affect lung development, and depending at which stage they occur, they can affect males and females at differential rates [41, 94–99]. For example, any insult that occurs in the pseudoglandular phase may cause developmental alterations, resulting in changes to the airway branching system. Similarly, in the saccular stage, developmental damage already affects the gas-exchange components and results in structural alterations of the later pulmonary parenchyma. In addition, impairment of the fetal airway development, for example, due to in utero exposure to toxins, may increase the risk of childhood respiratory infection and disease [100]. It has been described that in utero smoke exposure and postnatal exposure to smoke and/or air pollution may influence airway and lung development, which may lead to small airway abnormalities and asthma later in life [1, 101]. In this regard, some studies showed that maternal smoking affects lung function of female infants more than that of male infants, but others reported the opposite effect, or no effect, indicating that the damaging effects of smoke exposure may be dependent on the frequency, concentration, and time of exposure [94–96, 102–105]. Perinatal nicotine exposure affects lung growth and differentiation by altering developmental signaling pathways that are necessary for fetal lung development, resulting in the predisposition to childhood asthma [91].
< div class='tao-gold-member'>
Only gold members can continue reading. Log In or Register a > to continue
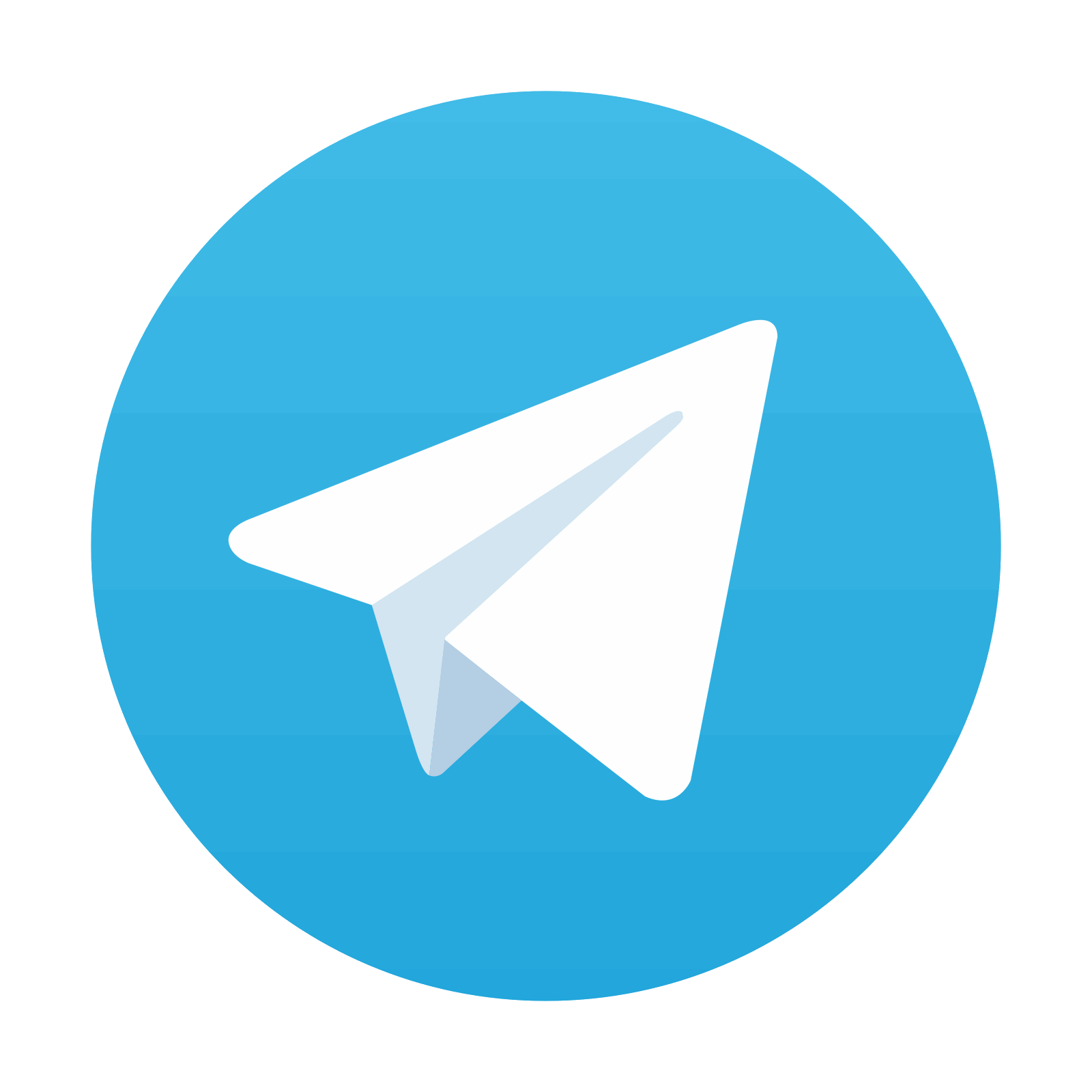
Stay updated, free articles. Join our Telegram channel

Full access? Get Clinical Tree
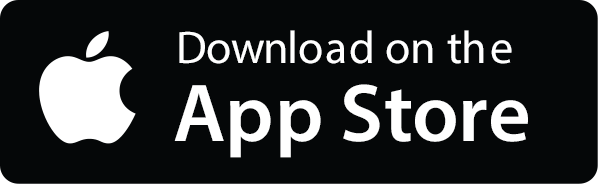
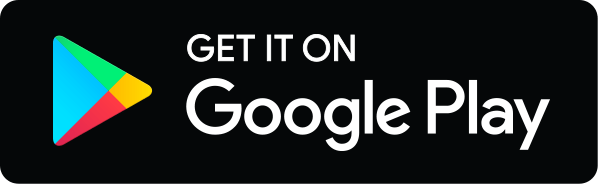