Fig. 1
Effects of sympathetic activation and the role of afferent renal sympathetic nerves in the development and control of hypertension (Reproduced with permission of Springer: The role of the kidney and the sympathetic nervous system in hypertension. Paediatric Nephrology 2015 (Thomas and Dasgupta 2015))

Fig. 2
Diagram demonstrating the radiofrequency renal denervation procedure

Fig. 3
Image showing renal denervation catheter in the left renal artery at the point of delivering radio-frequency ablation
It has been demonstrated in both animal models and humans that those with primary hypertension have higher sympathetic nerve activity (SNA) compared to those with normal blood pressure (Esler et al. 2003; Grassi et al. 1998; Yamada et al. 1989). Increased SNA causes vascular remodelling and smooth muscle hypertrophy leading to increased peripheral resistance (Oparil et al. 2003). These changes in the peripheral vasculature play important roles in the development and maintenance of chronic hypertension. Moreover, increased SNA in hypertension contributes to end organ damage as suggested by the correlation between left ventricular mass and reduced vascular compliance and elevated circulating noradrenaline (Marcus et al. 1994; Grassi et al. 1995). SNA overactivity may also be responsible for diastolic hypertension in young as indicated by the correlation between heart rate and diastolic blood pressure suggesting autonomic nervous system imbalance in the form of relative SNA overactivity (Kim et al. 1999). SNA overactivity has also been implicated in obesity related hypertension. Both the renal and cardiac sympathetic activities have been demonstrated to be increased in this situation (Esler 2000).
1.1 Mechanism of Sympathetic Stimulation in Hypertension
The mechanism of sympathetic stimulation in hypertension is complex but perhaps involves both baroreceptor and chemoreceptor pathways. Both central and peripheral baroreceptors are reset at higher blood pressures in hypertension; and returns to normal as blood pressure is lowered with treatment (Chapleau et al. 1988; Guo et al. 1983; Xie et al. 1990). Factors implicated in sympathetic stimulation are summarised in Box 1.
Box 1: Factors Implicated in Activation of Sympathetic Nervous System in Hypertension
Abnormal sympathetic innervation and renal morphogenesis
Arterial baroreceptor resetting
Chemoreceptor stimulation to hypoxia and apnoea
Mental stress – recurrent stresses promotes increased vascular reactivity and maladaptive remodelling
Renal injury – stepwise relationship between declining renal function and SNA
Oxidative stress – experimental data show antioxidants reduce SNA
Reduced nitric oxide bioavailability – animal and human data showing ADMA correlates with SNA
Angiotensin II
Endothelin
Reproduced with permission of Springer: The role of the kidney and the sympathetic nervous system in hypertension. Paediatric Nephrology 2015 (Thomas and Dasgupta 2015).
1.2 Role of Renal Sympathetic Nerves in Hypertension
Renal nerves are the ‘communication link between the central nervous system and the kidney’. The major structural and functional components of the kidney, the vessels, glomeruli, and tubules are innervated. In response to various stimuli, both mechanical and chemical, the efferent renal sympathetic nerves are activated. Activation of the efferent renal sympathetic nerves leads to reduction in renal blood flow and glomerular filtration rate, increase in renal tubular sodium and water reabsorption, and increase in renin release. Inhibition of these nerves has the functionally opposite effects. The afferent renal sympathetic nerves generally, in response to stretch, exert an inhibitory effect through facilitating sodium and water excretion. This reno-renal reflex plays an important role in maintaining sodium and water homeostasis in various physiological and pathological states. However, in states of kidney disease and injury the excitatory fibres in the afferent sympathetic nerves are stimulated which leads to increased peripheral and renal sympathetic nerve activity leading to peripheral vasoconstriction, increased cardiac output, renal vasoconstriction, increased renin release and tubular salt retention all of which contribute to elevation of systemic blood pressure. It is postulated that it is the activation of these fibres that contribute to initiation and maintenance of essential hypertension (DiBona and Sawin 1999; DiBona and Kopp 1995, 1997).
The evidence for the important role that the renal sympathetic nerve play in the pathogenesis and maintenance of hypertension come from animal experiments. There is a suggestion that the association between the SNS and the kidney starts in utero. In rodents, glial cell line-derived neurotrophic factor (GDNF) is essential to the normal development of renal sympathetic innervation and glomerular morphogenesis (Sainio et al. 1997). Deletion of a single GDNF gene in mice results in abnormal renal morphogenesis with the kidneys developing fewer, larger glomeruli and higher arterial pressures (~20 mmHg) compared to control animals with two copies of the gene (Cullen-McEwen et al. 2003). In spontaneously hypertensive rats (SHR), neonetal sympathectomy reduce blood pressure long-term (Gattone et al. 1990; Head 1989). This is further confirmed when kidneys from neonatally sympathectomised SHR are transplanted into untreated SHR. Mean arterial pressure is lower in these animals compared to the control group in which kidneys from hydralazine treated animals are transplanted into untreated SHR. This suggests that neonatal sympathectomy induces chronic changes to SHR kidney function leading to reduction of mean arterial pressure even when extrarenal sympathetic tone is restored (Grisk et al. 2002). It is suggested that it is the impairment of the reno-renal reflex mentioned above that contributes to the development of hypertension in SHR. Furthermore, there is a lot of evidence demonstrating renal denervation can delay development and attenuate several different types of experimental hypertension (Stella and Zancetti 1991).
Experiments in both rat models and humans have demonstrated that the SNS exhibits preferential activation of renal sympathetic fibres in response to baroreceptor unloading (Scislo et al. 1998; Johansson et al. 2003). In the human experiment enalaprilat, an intravenous angiotensin-converting enzyme inhibitor, was administered to healthy individuals. There was a minor drop in mean arterial pressure, but renal noradrenaline spillover increased to 26 % and 49 % in response to low and high dose enalaprilat respectively, whilst the cardiac and total body noradrenaline spillover remained constant. These findings suggest that in healthy individuals without activated renin angiotensin system, there is selective stimulation of renal sympathetic nerve activity in response to baroreceptor unloading (Johansson et al. 2003). Purported mediators of baroreflex resetting include angiotensin II and oxygen free radicals (Abboud 1974; Li et al. 1996).
1.3 Historical Treatments for Hypertension – Modulation of Sympathetic Activity
On the basis of the vasoconstrictor and cardioaccleratory properties of the sympathetic nervous system, a number of therapies have been developed in an attempt to modify the effect of the sympathetic nervous system on blood pressure (BP) control. Efficacy of these therapies further support the important role the SNA plays in hypertension. In 1923, Fritz Bruening performed the first surgical sympathectomy for hypertension based on the hypothesis that reduction of sympathetic outflow will lead to reduction in blood pressure (Freis 1990). This original concept was further developed by other surgeons such as Peet and Smithwick (Peet 1947; Smithwick and Thompson 1953) who performed more extensive operations termed splanchnicectomy which was offered to those with severe hypertension with evidence of cardiovascular damage. These procedures resulted in impressive reduction in blood pressure but were associated with significant surgical morbidities and complicated by orthostatic symptoms. With the availability of effective medical therapy these procedures were abandoned. However, the success of surgical sympathectomy in reducing high blood pressure led to the development of drugs producing chemical sympathectomy. A number of ganglion blocking agents were developed to treat hypertension like hexamethonium and tetraethylammonium chloride (Lyons et al. 1947; Paton and Zaimis 1948). These agents were the first pharmacological agents to block sympathetic outflow from the ganglion and also one of the earliest medical therapies for hypertension. Alpha-methyldopa a centrally acting anti-hypertensive agent which acts by inhibiting sympathetic outflow through binding to α2 adrenoreceptors became available in the 1960s (Oates et al. 1960). Use of this drug is uncommon nowadays except in the context of hypertension in pregnancy. The role of sympathetic nervous system in the pathogenesis of hypertension is mediated by the alpha and beta adrenergic receptors. Beta-blockers were shown to be beneficial in the management of hypertension in 1964 (Prichard and Gillam 1964). The alpha-1 receptors present in the blood vessels are responsible for peripheral resistance. Selective alpha-1 receptor blockers were developed with a view to treating hypertension by reducing elevated total peripheral resistance (Nash 1990). However, in practice these agents have been found to have very modest blood pressure lowering effect (Heran et al. 2012).
Another example of sympathetic modulation to achieve blood pressure control is bilateral nephrectomy that used to be carried out in dialysis patients with refractory hypertension (Zazgornik et al. 1998). The improvement in blood pressure control in this situation is likely to be due to removal of the sympatho-excitatory effects of the damaged kidneys.
2 Catheter-Based Renal Denervation in Treatment Resistant Hypertension
A greater understanding of the role of the sympathetic nervous system in the pathogenesis and maintenance of primary hypertension and the lessons learnt from modulation of sympathetic activity in hypertension control has led to a number of novel device based therapies of which catheter based renal denervation (RDN) is the foremost. Initial encouraging results from clinical studies led to more than 10,000 procedures being carried out in treatment resistant hypertension (TRH) across the world in the last few years.
2.1 The Procedure
The renal nerves arise from T10 to L2 spinal segments, arborise around the renal artery and primarily lie within the adventitia of the artery. This presents an opportunity to ablate the renal sympathetic nerves percutaneously via the lumen of the main renal artery using a catheter connected to a radiofrequency generator. The access is gained through the common femoral artery which is punctured, under conscious sedation and local anaesthetic injection to the groin, using ultrasound guidance (Figs. 2 and 3). A guide sheath is introduced using a standard Seldinger technique. A renal angiogram is performed using a non-ionic contrast agent to delineate the renal artery, ostia, bifurcation and accessory arteries. If the renal artery anatomy is suitable, a renal denervation catheter is positioned in the renal artery under fluoroscopic guidance at an initial position proximal to bifurcation of the renal artery. The early renal denervation catheters developed were unipolar with a flexible tip fitted with an electrode that can discharge radiofrequency waves when connected with a radiofrequency generator. More recently multipolar catheters of various designs have been developed. These are fitted with multiple electrodes capable of delivering multiple ablations simultaneously.
The treatment involves the delivery of relatively low power and precisely focused radiofrequency bursts through the wall of the renal artery to disrupt the surrounding renal nerves lying in the adventitia. Depending on the type of the catheter being used, unipolar or multipolar, it is repositioned after each treatment to ablate the sympathetic nerves in each quadrant of the renal artery in a helical pattern. Catheter tip temperature and impedance are constantly monitored during ablation, and radiofrequency energy delivery is regulated according to a predetermined algorithm. After a satisfactory treatment, a repeat selective renal arteriogram is taken to ensure patency of the renal artery post-ablation. The process is then repeated in the other renal artery and/or any accessory renal arteries. Following successful completion of the procedure a vascular closure device is deployed.
2.2 Pre-clinical and Proof of Principle Studies of Renal Denervation
The preclinical studies of catheter-based RDN were carried out in juvenile swine model. It was shown to be comparable to direct surgical RDN via renal artery transection and re-anastomosis. As proof of success, noradrenaline spillover rates were reduced by more than 85 % after denervation (Krum et al. 2009). In humans, bilateral renal denervation was demonstrated to reduce noradrenaline spillover, decrease renin activity, and increase renal plasma flow at 30 days after the procedure (Schlaich et al. 2009).
The proof-of-concept trial of RDN in humans (SYMPLICITY HTN-1), included 45 patients with treatment resistant hypertension with a clinic systolic blood pressure (BP) ≥160 mmHg who underwent RDN using the single electrode catheter (Symplicity catheter system, Ardian Inc. Mountain View, CA, USA; Medtronic Inc., Santa Rosa, CA, USA) (Krum et al. 2009). All patients were taking at least three antihypertensive agents of different classes including a diuretic. Significant reductions in office BP readings compared to baseline were observed, −22/−11 mmHg and −27/−17 mmHg at 6 month and 12 month after RDN respectively.
Serial estimated glomerular filtration rate (eGFR), renal angiography and magnetic resonance renal angiography (MRA) were used to assess safety of the procedure. The procedure was found to be safe with no significant change in renal function and no incidence of renal artery stenosis on the MRA at 6 months. Two patients experienced adverse events; one had renal artery dissection which occurred before any radiofrequency ablation could be applied to the artery and the second had a pseudoaneurysm at the femoral access site.
To assess physiological response of renal denervation, efferent sympathetic nervous system activity at the level of the kidneys was assessed by isotope dilution renal noradrenaline spillover testing in a subgroup of 10 patients. A mean reduction of noradrenaline spillover of 47 % (95 % confidence interval: 28–65 %) was observed 1 month after denervation. These observations, alongside the substantial reductions in clinic BP suggested successful targeting of efferent renal nerves. Therefore, this initial proof-of-concept study in human subjects demonstrated that the procedure was safe and was associated with a significant BP reduction at 1 year.
Furthermore, BP reduction following renal denervation was sustained. One hundred and fifty three patients undergoing open-label RDN, including the cohort of the proof of principle study, were followed up for 3 years. There was a mean reduction of 32/14 mmHg in office BP at 3 years with 93 % of the patients having a reduction in systolic BP of greater than ≥10 mmHg (Krum et al. 2014). The average number of BP-lowering medications prescribed at baseline and 36 months were similar. Sustained BP reduction suggested that re-growth of efferent nerve fibres had not occurred over time.
2.3 Further Clinical Studies Demonstrating Efficacy of Catheter Based Renal Denervation
An open-label, multicentre, randomised control trial (RCT) of renal denervation versus usual care in patients with TRH further supported the efficacy and safety of the procedure (SYMPLICITY HTN-2) (Esler et al. 2010). In this open labelled RCT, efficacy of RDN was compared against usual care; 106 patients were randomised to either RDN or a control arm. At 6 months, there was a 32/12 mmHg reduction in office BP in the RDN group, but no difference in office BP in the control arm, when compared with baseline BP. No procedural or device-based adverse events were observed.
Following these encouraging results a number of new catheter systems were developed and the efficacy tested in clinical trials. A meta-analysis of the early studies of renal denervation further supported the BP lowering benefits of this therapy (Davis et al. 2013). In the RCTs, there were reductions in mean office BP at 6 and 12 months of 28.9/11.0 mmHg and 25.4/10.0 mmHg respectively with RDN compared with medical therapy. Similar lowering in office BP was observed in uncontrolled studies; reductions of 25.0/10.0 mmHg and 22.8/10.6 mmHg respectively at 6 and 12 months post RDN compared with baseline.
2.3.1 Pitfalls of Early Studies of Renal Denervation
There were two main criticisms of early studies of catheter based renal denervation. Ambulatory blood pressure (ABP) readings were infrequently used for primary assessment of BP response. The few studies, which reported on the ABP, showed much lower BP reductions with a mean of 13.2/7.3 mmHg at 6 months after procedure compared with baseline (Davis et al. 2013). Secondly, there was absence of blinding due to the invasive nature of the procedure. Therefore, the placebo effect could not be excluded and the medication taking behaviour of the patient may have been altered by inclusion in a device-based trial.
2.4 The Sham Controlled Trial of Renal Denervation
Sham controlled trials are considered to be the most rigorous way of assessing efficacy of new surgical techniques or device-based interventions. As such, RDN was compared against a sham procedure where the patients randomised to the sham arm received a renal angiogram only (SYMPLICITY HTN-3) (Bhatt et al. 2014). This RCT included 535 patients with TRH across 88 centres in the USA and was designed to address the issues raised regarding the design of prior open-label RDN studies. The mean change in office systolic BP after 6 months were −14.1 mmHg and −11.7 mmHg respectively in the denervation and sham arms, an absolute difference of 2.4 mmHg between the groups. The primary efficacy end point, a difference of 5 mmHg between the groups in change of office systolic BP from baseline to 6 months, was not met. The secondary efficacy end point of a 2 mmHg difference between groups in change in 24-h ambulatory systolic BP was also not met; there was a reduction of 6.75 mmHg obtained in the denervation group and 4.79 mm Hg in the sham-procedure group. However, there was no difference between the groups in major safety end points, 1.4 % in denervation group versus 06 % in the sham group. Therefore, the sham-controlled study suggests that catheter based renal denervation is a safe procedure but does not lower BP effectively in patients with treatment resistant hypertension.
2.4.1 Critical Analysis of the Sham Controlled Study
Critical analysis of this study by the investigators and others suggest a number of factors which may have been associated with better BP response in some patients within this trial (Kandzari et al. 2015; Lobo et al. 2015a). Factors which have been shown to be associated with favourable BP response within this trial include (Box 2):
- (a)
Number of ablations: Consistently greater reductions in BP were observed in patients who received a higher number of renal artery ablations in the treatment group. An increased difference in office and ambulatory BPs with an increasing number of ablations was observed after patients in the two groups were propensity score matched for the baseline characteristics. This trend was shown to be statistically significant. Twelve or more ablations in both renal arteries were found to be predictive of a greater change in ambulatory systolic BP. There was no increase in safety events corresponding to the increasing number of renal artery ablations.
- (b)
Pattern of ablation: There was a trend towards an increasing BP response with delivery of ablations in a four-quadrant pattern to neither, one or both renal arteries. However, this was not statistically significant. In the treatment arm, only 19 (5 %) patients received four-quadrant ablations in both renal arteries.
- (c)
Younger age: The subgroup of patients <65 years in age was associated with office systolic BP change in the RDN group on univariate analysis but not in the multivariable model.
- (d)
Ethnicity: A significant difference in office SBP was observed in the non-American-African subgroup as compared with the African-American subgroup, but there was no significant difference observed in mean 24-h ambulatory BP or home BP.
- (e)
Higher baseline clinic systolic BP: Multivariable analysis of the overall group identified baseline office SBP ≥180 mmHg was a positive predictor of 6-month response of office BP reduction.
- (f)
Type of antihypertensive medications used: Multivariable analysis showed use of aldosterone antagonist to positively predict an increased 6 month change in office SBP. Conversely, use of vasodilators was found to be a negative predictor for change in office SBP at 6 months.
- (g)
Higher baseline eGFR: Baseline eGFR ≥60 mL/min/1.73 m2 was shown to be an independent predictor of a greater change in ambulatory BP at 6 months.
Box 2: Factors Associated with Favourable Blood Pressure Response Observed in Clinical Studies of Renal Denervation
Younger age (<65 years)
Non African American ethnic origin
Higher baseline office systolic blood pressure (≥180 mmHg)
Concurrent use of aldosterone antagonists
Non-use of a vasodilator
Total number of ablations in both renal arteries (≥12)
4-quadrant ablation of renal arteries
Higher baseline eGFR (≥60 ml/min/1.73m2)
Factors that may have contributed to the apparent ineffectiveness of RDN in the sham-controlled study are operator inexperience and medication adherence. The procedure-related predictors observed, four quadrant ablations and total number of ablations in both renal arteries, could be a consequence of operator inexperience of a relatively new procedure. In 88 centres in the US, where the study was conducted, 364 RDN procedures were carried out. One hundred and eleven interventionists performed at least one procedure, with approximately one third doing one procedure and only 26 operators doing more than five procedures suggesting the majority of interventionists were relatively inexperienced with the RDN procedure (Lobo et al. 2015a). The procedure had been unlicensed in the US prior to this trial so the learning curve of the operators fell within the period of the trial.
Adherence to prescribed antihypertensive medication was assessed by self-reported diary completed by the patient. This is an indirect method of assessing mediation adherence which does not prove actual ingestion, is open to manipulation and is likely to over-estimate adherence. It is important to assess adherence to prescribed antihypertensive medication in patients with TRH objectively as up to half may be completely or partially non-adherent (Hameed et al. 2016; Rosa et al. 2014). Inclusion in a clinical trial may result in a change in the subjects’ pill-taking behaviour due to their awareness of being observed. This phenomenon is termed ‘the Hawthorne effect’.
2.5 Results of Studies Published After the Sham Controlled Trial
Since the publication of SYMPLICITY HTN-3, a number of studies have been published which help us understand the role of RDN in the management of treatment resistant hypertension and give us a future direction. Some of the main studies are described here.
A prospective, open-label, multicentre registry was set up to assess the safety and efficacy of RDN (Global SYMPLICITY Registry) (Böhm et al. 2015). The results of nearly 1000 patients undergoing RDN around the world confirm the favourable safety profile of the procedure. The mean 6-month reduction in office and 24-h ambulatory systolic BP were 11.6 mmHg and 6.6 mmHg respectively. This was a real-world registry with more lenient inclusion criteria. The greatest reduction in clinic and 24-h ambulatory systolic BPs (20.3 mmHg and 8.9 mmHg respectively) were seen in patients with severe hypertension at baseline: office systolic BP ≥160 mmHg with a 24-h ambulatory systolic BP ≥135 mmHg and taking ≥3 antihypertensive medication.
The results of an open-label, multicentre RCT of stepped-care standardised antihypertensive treatment (SSAHT) with or without RDN for TRH was published (Denervation for Resistant Hypertension, DNERHTN) (Azizi et al. 2015). This was a rigorously designed trial which randomised patients to either RDN or to continue on SSAHT after confirmation of TRH by ABPM 4 weeks after having been commenced on SSAHT. There were 48 patients in the RDN group and 53 patients in the control group. Adherence to antihypertensive medications was assessed using a validated Morisky Medication Adherence Scale [MMAS-8] and 24-h ABPM was used to measure the primary efficacy of RDN in this trial.
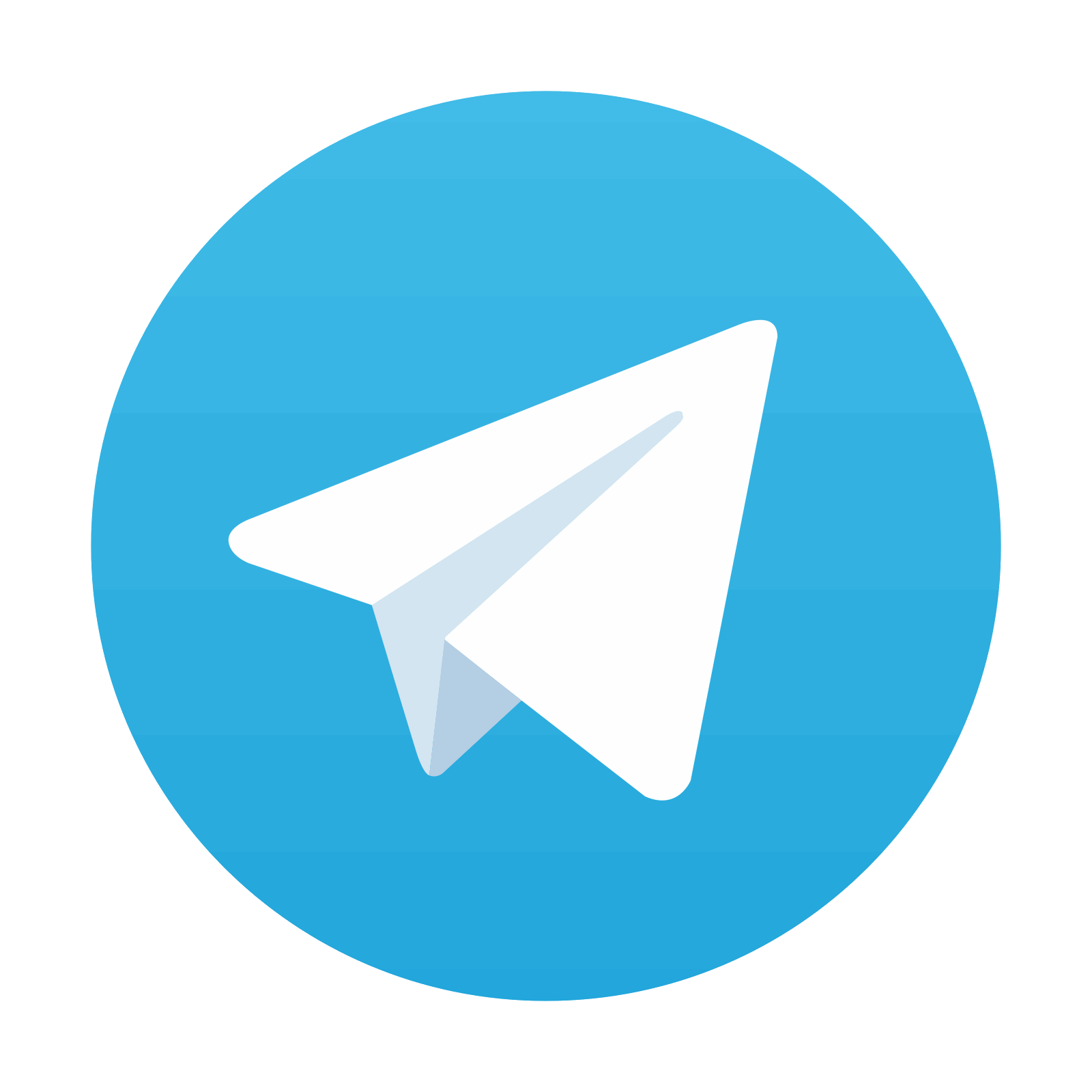
Stay updated, free articles. Join our Telegram channel

Full access? Get Clinical Tree
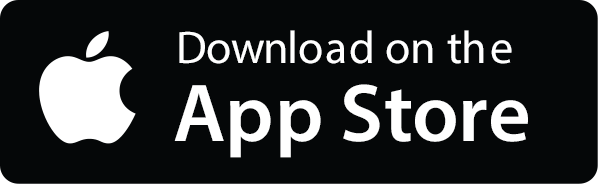
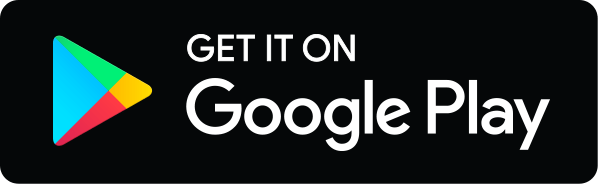