Deep venous thrombosis (DVT) is a common disease with potentially serious consequences such as pulmonary embolism (PE). Although the risk without prophylaxis of all venous thromboembolism (VTE) was 15% in one study of medical inpatients, the rate of clinically symptomatic DVT was only 6% (n = 188). VTE represents a potentially fatal disease process with a clinical presentation that is often silent or nonspecific. A substantial number of patients remain at risk as a result of the demographics of an aging population and the ability of modern medicine to treat patients with chronic disease. During the past decade, many of the long-standing controversies surrounding the natural history, diagnosis, and therapy of patients with VTE have been partially or completely reconciled, resulting in substantial changes in the diagnostic and therapeutic approach to the disease. In the evaluation of patients with suspected VTE, an understanding of what is unknown can prove invaluable to the decision-making process.
The age-adjusted incidence of first lifetime thrombosis is 1.92 per 1000 person-years, which translates into nearly 190,000 noted thromboses each year in persons ages 45 years or older in the United States.1 DVT is especially common in hospitalized patients and may constitute the greatest mortality risk in some inpatient populations. The overall incidence of a first VTE seems to be similar among men and women, but the risk is higher among women of childbearing age than among men in the same age group. This gender difference likely relates to the association of VTE with pregnancy or the use of oral contraceptives. In contrast, the risk of thromboembolism among older women is substantially lower than that among men in the same age group.2,3 The 28-day fatality rate after a first thrombosis is 11%, with cancer conferring an increased mortality risk. The 2.2-year recurrence rate after a first thrombosis is substantial at 7.7% per year.2 Thrombosis rates increase with age and are more than twofold higher in those older than age 65 years. The relatively low incidence of VTE in Asians and Hispanics has not been explained but may relate to a lower prevalence of genetic factors predisposing to VTE, such as factor V Leiden, in Asian populations (0.5%) compared with whites (5%).2
The proportion of patients with VTE categorized as idiopathic falls in the range of 26% to 47% of first-time cases; the exact figure depends partly on the definitions of idiopathic and secondary VTE.2 The majority of calf vein thrombi resolve spontaneously, and PE is uncommon. About 20% to 30% of DVTs propagate to the popliteal, femoral, or iliac veins, and 10% to 20% of all DVTs occur in proximal veins without prior calf involvement. Iliofemoral thromboses appear to be the source of most clinically apparent pulmonary emboli (Figure 31-1).
FIGURE 31-1.
Diagram of the leg veins (anterior view of the right leg). DVT, deep venous thrombosis.
(©Photo by Lianne Friesen and Nicholas Woolridge. This work is protected by copyright and the making of this copy was with the permission of Access Copyright. Any alteration of its content or further copying in any form whatsoever is strictly prohibited unless otherwise permitted by law. From Scarvelis D, Wells PS: Diagnosis and treatment of deep-vein thrombosis. CMAJ. 2006;175(9:1).)

In 1856, Virchow defined the classic triad of venous stasis, alternations in coagulation, and vascular injury as the primary factors in the pathogenesis of VTE. Since that time, a considerable amount of clinical and experimental evidence has been amassed in support of this theory of pathogenesis. Acquired and hereditary risk factors for VTE are described in Table 31-1.
Hereditary Thrombophilias |
Protein C deficiency Protein S deficiency Antithrombin III deficiency Factor V Leiden mutation Prothrombin 20210 G → A variation Hyperhomocysteinemia Dysfibrinogenemia Familial plasminogen deficiency |
Acquired Surgical Predispositions |
Major thoracic, abdominal, or neurosurgical procedures requiring general anesthesia and lasting >30 min Hip arthroplasty Knee arthroplasty Knee arthroscopy Hip fracture Major trauma Open prostatectomy Spinal cord injury |
Acquired Medical Predispositions |
Prior venous thromboembolism Advanced age (particularly older than age 60 years) Malignancy Congestive heart failure Cerebrovascular accident Nephrotic syndrome Estrogen therapy Pregnancy and the postpartum period Obesity Prolonged immobilization Antiphospholipid antibody syndrome Lupus anticoagulant Inflammatory bowel disease Paroxysmal nocturnal hemoglobinuria Behçet’s syndrome |
The prevention strategy used for DVT and PE should be based on the patient’s level of risk as suggested by the American College of Chest Physicians (ACCP) Consensus Conference on Antithrombotic Therapy. The risk categories include the following:
Low-risk patients:
Age younger than age 40 years
Uncomplicated minor surgery with clinical risk factors VTE
Risk of proximal DVT, 0.4%; risk of clinical PE, 0.2%; and risk of fatal PE, 0.002%
Moderate-risk patients:
Any surgery (minor or major) in patients between 40 and 60 years of age without additional risk factors
Major surgery in patients younger than age 40 years
Minor surgery in patients with additional risk factors
Risk of proximal DVT, 2% to 4%; risk of clinical PE, 1% to 2%; and risk of fatal PE, 0.1% to 0.4%
High-risk patients:
Major surgery in patients older than age 60 years without additional risk factors
Major surgery in patients ages 40 to 60 years who have additional risk factors
Risk of proximal DVT, 4% to 8%; risk of clinical PE, 2% to 4%; and risk of fatal PE, 0.4% to 1%
Highest risk patients:
Patients older than age 40 years with prior VTE, malignant disease, or hypercoagulable state undergoing major surgery
Patients with elective major lower extremity orthopedic surgery, hip fracture, stroke, multiple trauma, or acute spinal cord injury
Risk of proximal DVT, 10% to 20%; risk of clinical PE, 4% to 10%; and risk of fatal PE, 1% to 5%
In the DVT FREE Steering Committee, prospective registry of 5451 patients with ultrasound-confirmed DVT, the most common comorbidities were arterial hypertension (50%), surgery within 3 months (38%), immobility within 30 days (34%), cancer (32%), and obesity (27%).4 Although studies to date have yielded inconsistent results, long automobile or airplane trips appear to be risk factors for VTE.
Obesity is associated with increased risk of venous stasis and VTE. This increased risk may be attributable to elevated plasma levels of certain clotting factor such as fibrinogen, factor VII, and plasminogen activator inhibitor-1 (PAI-1) or as a result of platelet activation caused by enhanced lipid peroxidation.5 A history of DVT substantially increases the risk of subsequent thrombotic events. Patients who are undergoing lower extremity orthopedic procedures such as total hip replacement (THR) or total knee replacement (TKR) develop DVT in more than 50% of cases.6 Other surgical procedures such as spinal surgery, pelvic surgery, and neurosurgery place patients at a particularly high risk for DVT. Trauma, particularly of the lower extremities and pelvis, heightens the risk of DVT as well.
Cancer clearly augments the risk of VTE, although the precise pathogenesis of thromboembolism in cancer is not well understood. Numerous mechanisms, including intrinsic tumor procoagulant activity and extrinsic factors such as chemotherapeutic agents and indwelling access catheters, contribute to this process. Pancreatic, lung, gastric, genitourinary tract, and breast malignancies are associated with a particularly high risk of DVT.
Pregnancy and the postpartum period are the most common settings in which women younger than age 40 years acquire thromboembolic disease. DVT is more common in the third trimester and postpartum period. The use of oral contraceptive pills also increases risk for thromboembolism. The use of oral contraceptives should be avoided by women older than age 35 years with a smoking history; those with protein C, protein S, or antithrombin III deficiency; and those who are homozygous for factor V Leiden mutation.
Inherited thrombophilia is a genetic tendency to VTE that usually presents in patients younger than age 50 years and is often recurrent. The most frequent causes of an inherited (primary) hypercoagulable state are the factor V Leiden mutation; a prothrombin gene mutation; or defects in protein S, protein C, and antithrombin III. (Refer to Table 31-2 for relative risk for DVT.)
Condition | Relative Risk | Incidence (%/yr) |
---|---|---|
Normal individuals without risk factors | 1 | 0.008 |
2.5 | 0.02 | |
Hyperhomocysteinemia | 1 | — |
Prothrombin gene mutation | 2.8 | 0.02 |
History of use of oral contraceptives | 4 | 0.03 |
Factor V Leiden (heterozygous) deficiency | 7 | 0.06 |
History of oral contraceptives use plus heterozygous factor V Leiden deficiency | 35 | 0.29 |
History of factor V Leiden deficiency (homozygous) | 80 | 0.5–1.0 |
Factor V Leiden is the most common cause of inherited thrombophilia, accounting for 40% to 50% of cases. The factor V Leiden mutation leads to a hypercoagulable state for two reasons because of the critical position of factor V in both the coagulant and anticoagulant pathways.7 The relative risk for DVT is increased sevenfold for patients who are heterozygous and 80-fold for those who are homozygous for factor V Leiden mutation. Protein C is a vitamin K–dependent protein synthesized in the liver. Heterozygous protein C deficiency is inherited in an autosomal dominant fashion. The frequency of this abnormality ranges from one per 200 to one per 500 in a healthy general population.8 It is estimated that congenital protein C deficiency is present in 2% to 5% of patients presenting with thromboembolism.9 Protein S, a vitamin K–dependent glycoprotein, is a cofactor of the protein C system. The clinical presentation of patients with heterozygous protein S deficiency is similar to that of those with antithrombin or protein C deficiency because the incidence of venous thrombosis is increased.
Prothrombin 20210 G mutation, a sequence variation in the prothrombin gene (20210 GA), was described in 1996 and is estimated to occur in approximately 0.7% to 6.7% in the population, with the highest prevalence rates reported in Spain. There is an increased risk for venous thrombosis in carriers of the prothrombin G–>A20210 gene variant.
Hyperhomocysteinemia has also been identified as a potential independent factor predisposing individuals to VTE. Elevation of plasma homocysteine levels may be the result of genetic abnormalities, nutritional deficiencies of vitamins (B6, B12, folate), clinical disorders (renal insufficiency, hypothyroidism, inflammatory bowel disease), or a combination of these factors. Antithrombin (AT, formerly called AT III, also known as heparin cofactor I) is a vitamin K–independent glycoprotein that is a major inhibitor of thrombin and other coagulation serine proteases, including factors IXa and Xa.
Unselected population-based screening for inherited thrombophilia is not recommended for the following reasons. First, the condition is present in low frequency in the general population. Second, the penetrance is low for the symptomatic condition among carriers of the most common thrombophilic conditions. Last, there is no safe, cost-effective, long-term method of prophylaxis.
Classic symptoms of DVT include swelling, pain, and discoloration in the involved extremity. Symptoms in the calf alone are often the presenting manifestation of significant proximal vein involvement, and some patients with whole-leg symptoms are found to have isolated calf vein DVT. A 1984 study published in Lancet defined calf symptom sensitivity, specificity, and accuracy respectively as follows: edema, 97%, 33%, and 70%; pain, 86%, 19%, and 58%; and warmth, 72%, 48%, and 62%, respectively.10 The physical examination may reveal a palpable cord reflecting a thrombosed vein, ipsilateral edema, warmth, or superficial venous dilatation. However, the clinical diagnosis of DVT is not sufficiently accurate because the symptoms and findings are often nonspecific. “Classic” findings, such as Homan’s sign (calf pain with flexion of the knee and dorsiflexion of the ankle), Moses’ sign (pain with calf compression against the tibia), and a palpable cord, occur infrequently and are nonspecific.
Surgical procedures, trauma, pregnancy, heart failure, and immobility increase the risk of DVT. The presence of recurrent fetal loss suggests the possible presence of an inherited thrombophilia or antiphospholipid antibodies. Collagen vascular disease, myeloproliferative disease, atherosclerotic disease, and nephrotic syndrome may increase the risk of DVT. Hydralazine, procainamide, and phenothiazines may induce antiphospholipid antibodies that also increase the risk of DVT. A well-documented history of venous thrombosis in one or more first-degree relatives strongly suggests the presence of a hereditary defect (see Table 31-2).
Several clinical prediction rules for venous thrombosis have been developed and validated.11 The Wells rule, initially described in 1995 and revised in 1997 to include nine clinical features, proved capable of stratifying patients with suspected venous thrombosis into three probability categories: low, moderate, and high. The incidence of venous thrombosis in these categories approximates 3%, 17%, and 75%, respectively.12 By using this prediction rule in combination with lower extremity ultrasonography and D-dimer, the diagnosis of venous thrombosis can be safely excluded in patients with a low clinical likelihood of venous thrombosis. Such an approach dramatically reduces the need for contrast venography or serial lower extremity ultrasound studies.
The Wells prediction rule was subsequently revised in 2003 to include 10 clinical characteristics capable of stratifying outpatients into clinically likely and clinically unlikely categories (Tables 31-3 and 31-4). For outpatients falling into the clinically unlikely category, DVT was reliably excluded when the result of a sensitive D-dimer assay result was negative, thereby limiting the need for ultrasound evaluation. The ability to exclude venous thrombosis in outpatients using a clinical prediction rule and negative results of a D-dimer assay has been confirmed in other studies.13 It should be emphasized that clinical prediction rules constructed and validated in outpatients should be viewed critically before they are applied to an inpatient population.6
Clinical Feature | Score |
---|---|
Active cancer (treatment ongoing or within the previous 6 months or palliative) | 1 |
Paralysis, paresis, or recent plaster immobilization of the lower extremities | 1 |
Recently bedridden for more than 3 days or major surgery, within 4 weeks | 1 |
Localized tenderness along the distribution of the deep venous system | 1 |
Entire leg swollen | 1 |
Calf swelling by more than 3 cm compared with the asymptomatic leg (measured below the tibial tuberosity) | 1 |
Pitting edema | 1 |
Collateral superficial veins | 1 |
Alternative diagnosis (e.g., cellulitis, Baker’s cyst, lymphedema, venous insufficiency, leg injuries, leg swelling in paralyzed limb) more likely than DVT | –2 |
High probability | ≥3 |
Moderate probability | 1 or 2 |
Low probability | ≤0 |
Modification: | |
This clinical model has been modified to take one other clinical feature into account: a previously documented deep vein thrombosis (DVT) is given the score of 1. Using this modified scoring system, DVT is either likely or unlikely, as follows: | |
DVT likely | ≥2 |
DVT unlikely | ≤1 |
However, the Wells prediction rule performs best in younger patients without comorbidities or a history of DVT. |
D-dimer is a marker of endogenous fibrinolysis and should therefore be detectable in patients with DVT. Enzyme-linked immunosorbent assay (ELISA), quantitative rapid ELISA, and advanced turbidimetric D-dimer determinations are highly sensitive tests that are helpful in the diagnosis of VTE.
The evaluation of a pretest probability model in conjunction with D-dimer testing has also been performed as a means to decrease the number of serial ultrasound examinations and to reduce the number of false-negative and false-positive ultrasound studies in patients suspected of having acute DVT.12,14,15,16,17,18,19 The use of D-dimer is controversial. In addition, the precise role of D-dimer assays as an adjunct to ultrasound examination for DVT has not been definitively established.20 Although the negative predictive value is exceptionally good in low-risk patients, it is unacceptable in high-risk patients. Ongoing investigation into the role of D-dimer assays as a part of the diagnostic workup of VTE continues, but these assays have not been standardized, and the results are variable and depend on thrombus location, size, interpretation of the results, and other factors.21
In the most recent study by Wells et al,17 1096 consecutive outpatients suspected of having DVT were scored according to the clinical likelihood of having a DVT and were then randomized to undergo ultrasound imaging alone or to undergo D-dimer testing followed by ultrasound imaging unless the D-dimer test result was negative and the patient was considered unlikely to have a DVT. Only 0.4% of patients in whom DVT was ruled out by D-dimer testing developed DVT. The authors concluded that DVT could be ruled out in a patient who was scored to be clinically unlikely to have a DVT and who had a negative D-dimer test result (using two different D-dimer assays with high negative predictive value for DVT). They also concluded that ultrasonography could be safely omitted in patients with a negative D-dimer result and a low clinical likelihood of DVT. Although the results of this randomized trial are promising, it is important to note that the study population consisted of outpatients, and further validation studies for high-risk inpatients with multiple comorbidities need to be performed.
Although imaging studies of the lower extremities are more readily available and are associated with lower morbidity and cost, they may be less accurate than invasive techniques. Thus, accurate assessment of the role of noninvasive imaging studies in the diagnosis of lower extremity thrombosis requires a direct comparison with the diagnostic results obtained from invasive testing. Clinical outcomes need to be determined in prospective studies on consecutive patients. A detailed discussion of the role of ultrasonography, venography, and impedance plethysmography (IPG) follows.
Ultrasonography. It is currently estimated that 1 million patients per year undergo ultrasound examination for suspected DVT. Of these examinations, only 12% to 25% have positive results.20 Venous ultrasonography has become the most widely used diagnostic modality for the diagnosis or exclusion of acute DVT. Duplex ultrasonography is considered to be the primary noninvasive diagnostic method for DVT. There are several types of venous ultrasonography. They include compression ultrasound (B-mode imaging only), duplex ultrasound (B-mode imaging and Doppler waveform analysis), and color Doppler imaging alone. Although these types of venous ultrasonography are sometimes used interchangeably, their sensitivities and specificities for detecting acute DVT vary.20 The diagnosis of venous thrombosis using compression ultrasonography is made by the findings such as abnormal compressibility of the vein, abnormal Doppler flow omitting the presence of an echogenic band, or an abnormal change in diameter during the Valsalva maneuver.
The optimal technique of lower extremity vein ultrasonography depends on the anatomical location being evaluated. Whereas compression ultrasonography is typically performed on the proximal deep veins, specifically the common femoral, femoral, and popliteal veins, a combination of duplex ultrasonography and color Doppler imaging is more often used to interrogate the calf and iliac veins.21 Unfortunately, venous ultrasonography examinations are not yet uniformly standardized and range from compression of as few as two deep veins to complete duplex and color Doppler evaluation of the entire lower extremity.22,23,24,25
There are, however, factors that influence which venous segments can be evaluated in an individual examination. These include morbid obesity, lower extremity edema or tenderness, and the presence of immobilization devices and bandages.20 In most instances, a complete color-flow venous duplex examination is the standard of care for assessment of lower extremity DVT. A venous duplex examination performed to exclude the presence of DVT should consist of evaluation of both the proximal and calf veins whenever possible.
The weighted mean sensitivity and specificity of all types of venous ultrasonography for the diagnosis of symptomatic proximal DVT are 97% and 94%, respectively. The high specificity of venous ultrasonography allows treatment of DVT to be initiated without further confirmatory tests, and the high sensitivity in diagnosing proximal DVT makes it possible to withhold treatment if the examination results are negative.20 Currently, repeat or serial venous ultrasonography seems advisable for negative examination results in symptomatic patients when a high suspicion for DVT exists and in patients for whom an alternative form of imaging is contraindicated or unavailable.21 According to Birdwell et al,22 it is safe to withhold anticoagulation in outpatients suspected of having a first episode of DVT if compression ultrasonography results are normal at presentation and on a single follow-up examination done 5 to 7 days later.
Current ultrasound technology is able to image calf veins in the large majority of patients. A well-trained technologist can interrogate these veins in 80% to 98% of cases using a combination of B-mode, Doppler waveform analysis, and color Doppler (Figures 31-2 and 31-3).26 Rose et al26 showed that in technically adequate studies, the sensitivity and specificity of color Doppler in isolated calf veins exceeded 90%. Others have also shown duplex ultrasonography and color Doppler sensitivities for detecting isolated calf vein thrombosis to be greater than 90%.26,27,28 Isolated calf vein thrombosis accounts for 20% of symptomatic DVT (see Figures 31-2 and 31-3). There are diagnostic limitations to the use of compression ultrasonography. Isolated thrombi in the iliac vein or the femoral vein are not detected by this method.29 Serial studies are required if the initial ultrasound study results are negative and high level of clinical suspicion exists. Approximately 2% of patients with an initially negative ultrasound result develop a positive result when retested 7 days later.30 Patients with pelvic neoplasm or abscesses may demonstrate an isolated noncompressible femoral vein even in the absence of thrombosis.31
Venography. Venography is performed according to a specific protocol described by Rabinov and Paulin in 1972.32 Contrast venography has traditionally been the accepted reference imaging examination for the diagnosis of DVT.33,34 Because of its high sensitivity for the detection of small, asymptomatic lower limb DVT, venography enables clinical trials to be conducted with smaller sample sizes than those evaluating clinical outcomes.20,35,36,37 The most reliable criterion for the diagnosis of venous thrombosis is a constant intraluminal filling defect evident in two or more views. Other criteria, such as nonvisualization of deep veins, venous collaterals, or no constant filling defects, are less reliable. However, there are downsides to using venography in the diagnosis of VTE. The study is not easy to interpret, especially in patients with a history of venous thrombosis. Other negative aspects for using venography include the high cost of the procedure and the potential for allergic reactions and nephrotoxicity from dye. The technique of venography is now practiced less often and taught to fewer radiology trainees.33
Doppler ultrasonography has limitations in the detection of asymptomatic DVT, particularly calf vein thrombi.37,38 These asymptomatic (silent) DVTs are often nonocclusive and small (1–2 cm) and are usually not associated with venous distension or impaired blood flow, which are important factors for the ultrasonographic diagnosis of symptomatic DVT.26,39 Although ultrasonography has both a sensitivity and specificity approaching 95% for the detection of symptomatic proximal DVT, this sensitivity decreases to 26% to 40%, respectively, for asymptomatic DVT.20,38,40,41 Conversely, contrast venography maps out and records venous anatomy in a form readily interpretable offsite and has a far higher rate of detection of asymptomatic distal DVT. Therefore, venography may be useful for asymptomatic DVT when ultrasonography is not diagnostic.
Impedance Plethysmography. IPG, an indirect technique that measures the rate of venous outflow, has been well standardized and carefully validated against contrast venography.42 IPG requires the patient to lie still while a thigh cuff is inflated. The change in blood volume at the calf is measured from the impedance of the calf as determined by electrodes wrapped around it.43 After rapid deflation of the cuff, the proportional change of impedance over the subsequent 3 seconds is used to measure venous outflow obstruction. Most facilities do not have either the equipment or skilled personnel to perform impedance plethysmography. False-positive results in IPG may be seen in variety of clinical scenarios that limit its role. These include diminished arterial inflow (congestive heart failure, shock, peripheral arterial disease, clinical situations in which impede venous return is impaired such as right ventricular failure, obstructive lung disease) and in circumstances of increased intrathoracic pressure (mechanical ventilation). Patients must lie still for at least 2 minutes and must be positioned correctly to avoid venous outflow obstruction. For this reason, it is difficult to perform this procedure in patients with paraplegia and in those with casted legs. Proximal vein thrombosis can be detected by this technique with a sensitivity and a specificity of 91% and 96%, respectively.43 Detection of calf vein thrombosis is poor by IPG. However, IPG may be helpful in assessing recurrent DVT. A distinct advantage of IPG in this setting is that it normalizes at a rapid, predictable rate after a first DVT compared with compression ultrasonography.44,45
Recently, algorithmic approaches (Figure 31-4) using a combination of clinical assessment, D-dimer measurements, and ultrasonography have been adopted into clinical practice with the goals of standardizing the diagnostic approach to DVT and reducing the number of negative ultrasound examination results.12,14,15,16,19 The utility of algorithms in the diagnosis of DVT, however, depends on the availability of diagnostic testing, the expertise of the technologists performing the ultrasound examinations, the patient population, the cost and reimbursement of the different tests, and the interpretation of the results. There have been no large, randomized, multicenter studies comparing the outcomes of branching pathways that included the adequate sample sizes needed to test the safety of these algorithms. However, there have been rigorously performed cohort studies that have incorporated algorithms using clinical pretest probability in combination with ultrasonography, D-dimer testing, or both.17
FIGURE 31-4.
Diagnostic algorithm using D-dimer testing and ultrasound imaging in patients with suspected deep venous thrombosis (DVT).
(From Zierler BK: Ultrasonography and diagnosis of venous thromboembolism. Circulation. 2004;109:I-9–I-14; with permission.)
*Duplex ultrasound includes complete evaluation of the lower extremity, including compression, Doppler waveforms, and color Doppler of the calf veins and iliac veins.
† Contrast venography (CV) or MR venography (MRV) are warranted if the initial duplex study is technically inadequate or nondiagnostic.

DVT of the lower extremities and PE, respectively, are the most frequent and life-threatening manifestations of VTE. Hence, the main goal in the initial treatment of patients with acute DVT is prevention of thrombus extension as well as early and late recurrences of VTE. Currently, these goals are achieved by using either unfractionated heparin (UFH) or low-molecular-weight heparin (LMWH) followed by an oral anticoagulant drug.
Anticoagulant therapy as a basis for treatment of DVT was initially proposed by Barritt and Jordan46 in a 1960 study in which they compared anticoagulant therapy with no anticoagulant therapy in patients with symptomatic DVT or PE. In this trial, patients with an acute PE who were treated with 1.5 days of heparin and 2 weeks of vitamin K antagonist (VKA) therapy had a significantly reduced rates of recurrent PE and mortality.46 Another retrospective study done in 197447 demonstrated that treatment of VTE with heparin infusion resulted in a significant reduction of both thromboembolic morbidity and mortality.
Initial treatment with oral anticoagulants may induce hypercoagulability. Therefore, it is now customary to treat patients with DVT initially with heparin until appropriate anticoagulation is achieved. The importance of using heparin as initial treatment along with a VKA was shown in a randomized, controlled study that reported a threefold higher rate of recurrent VTE in patients who received VKA alone.48 Patients with DVT should be treated with anticoagulants as soon as the diagnosis is confirmed by objective testing. If the clinical suspicion is high or if there is a delay before diagnostic testing can be performed, treatment should be started before confirmatory tests are completed.49
A number of pharmacologic and nonpharmacologic options are available for the initial treatment of patients with DVT. Some of the pharmacologic options include intravenous (IV) UFH, LMWH, and fondaparinux. Clinical trials have established that for heparin to be efficacious, a critical therapeutic level of heparin should be obtained within the first 24 hours of treatment.48,50,51,52,53,54 This therapeutic level corresponds to a measured activated partial thromboplastin time (aPTT) of 1.5 times the mean of the control value or the upper limit of the normal aPTT range, with a target range (aPTT ratio) of 1.5 to 2.5. The importance of reaching this therapeutic range was demonstrated in a randomized study in which recurrent thromboembolism occurred in 2.7% of those patients who reached the aPTT therapeutic threshold within 24 hours compared with 8.2% of those who did not reach this therapeutic range.55 An audit done in 1988 at three university-affiliated hospitals documented that 60% of treated patients failed to achieve an adequate aPTT response during the initial 24 hours of therapy and that 30% to 40% of patients remained subtherapeutic over the next 3 to 4 days.56 Consequently, most hospitals use nomograms to more effectively achieve a therapeutic range within the first 24 hours after initiating continuous heparin infusion. The starting dose of IV UFH for the treatment of patients with DVT is either a weight-adjusted regimen of a 80 U/kg bolus followed by 18 U/kg/h or a bolus dose of 5000 U followed by a continuous infusion of at least 30,000 U for the first 24 hours. Subsequently, the infused dose of UFH is adjusted using a standard nomogram to reach and maintain the aPTT that corresponds to therapeutic heparin levels (0.3–0.7 IU/mL anti-Xa activity by the amidolytic assay).49 This adjustment is important because heparin binds nonspecifically to plasma and cellular proteins, thus resulting in a variable anticoagulant response. Although a strong correlation between subtherapeutic aPTT values and recurrent thromboembolism exists, the relationship between supratherapeutic aPTT (i.e., an aPTT ratio >2.5) and increased bleeding is not as clear.57 Heparin is usually overlapped with warfarin for a minimum of 4 to 5 days until the International Normalized Ratio (INR) has been within the therapeutic range (2.0–3.0) for two consecutive days.58 The administration of IV UFH has a narrow therapeutic window requiring close monitoring and is therefore associated with increased cost and substantial manpower requirements. The use of IV UFH for longer than 1 month has been associated with the development of osteoporosis.59 Moreover, the risk of developing heparin-induced thrombocytopenia (HIT) increases with longer durations of patient exposure to heparin. For these reasons, it is necessary to switch from an IV UFH infusion to another form of anticoagulation such as a VKA when treating patients with DVT.
LMWH can be administered subcutaneously (SC) without the need for laboratory monitoring because of its less nonspecific binding, which leads to greater bioavailability. Additionally, LMWH also carries a lower risk of heparin HIT compared with UFH. These advantages make LMWH a popular alternative to UFH for the acute treatment of DVT. Randomized, controlled trials of treatment for acute DVT or PE have demonstrated that LMWHs are at least as effective and safe as UFH.60,61,62,63,64,65,66 A meta-analysis found a significantly lower mortality rate at 3 to 6 months among patients treated with LMWH compared with those receiving UFH (odds ratio [OR], 0.71, 95% confidence interval [CI], 0.53–0.94), with similar thromboembolism and bleeding complications between the treatment groups.67 LMWH significantly reduces both the occurrence of major hemorrhage during initial treatment of DVT and overall mortality at follow-up.68 Laboratory monitoring when using LMWH is advisable under certain clinical circumstances. Doses of LMWH vary in patients who are obese, have renal dysfunction, or are pregnant. Monitoring anti–factor Xa levels has been suggested for these patients with dose adjustment to a target range of 0.6 to 1.0 U/mL 4 hours after injection for twice-daily administration or 1.0 to 2.0 U/mL for once-daily administration. For obese patients, a weight-based prophylactic dosing of LMWH may be preferable to fixed dosing.
Outpatient treatment with LMWH is another therapeutic option when treating patients with DVT. However, this approach is unsuitable for patients with massive thrombosis, serious co-morbidities, or a high risk of hemorrhage (e.g., older age, recent surgery, history of bleeding, renal or liver disease). Currently, there is no Food and Drug Administration–approved indication for the use of LMWH as outpatient treatment for DVT with concurrent PE. When none of these complicating factors exists, LMWH can be used safely and effectively at home as the initial antithrombotic treatment as an alternative to standard inpatient heparin therapy (Table 31-5).69,70,71,72,73 Health care dollars can potentially be saved if patients can be treated safely and effectively as outpatients with LMWH. A 2007 Cochrane analysis involving approximately 1700 participants concluded that patients treated with home LMWH had a significantly lower rate of VTE recurrence (relative risk [RR] 0.61; 95% CI, 0.42–0.90) than those treated in the hospital with UFH or LMWH.74 Mortality rates and major and minor bleeding episodes were similar between the treatment groups.
Study Source | Treatment Regimen | Mortality, N/N (%) | Recurrent VTE, N/N (%) | Major Bleeding Rate, N/N (%) |
---|---|---|---|---|
Koopman et al71 | Nadroparin SC BID vs IV heparin | 14/202 (7) vs 16/198 (8) | 14/202 (7) vs 17/198 (9) | 1/202 (0.5) vs 4/198 (2) |
Chong et al70 | Enoxaparin (1.5 mg/kg/d SC) vs IV heparin | 2/150 (1.3) vs 2/148 (1.3) | 2/150 (1.3) vs 8/148 (5.4) | 15/150 (10.0) vs 20/148 (13.5) |
Levine et al72 | Enoxaparin (1 mg/kg SC BID) vs IV heparin | 11/247 (4) vs 17/253 (7) | 13/247 (5) vs 17/253 (7) | 5/247 (2) vs 3/253 (1) |
Fondaparinux is a synthetic highly sulfated pentasaccharide that has a sequence derived from the minimal antithrombin binding region of heparin. It binds to antithrombin with a higher affinity than the native pentasaccharide of UFH or LMWH. This causes a conformational change in antithrombin, which then significantly increases the ability of antithrombin to inactivate factor Xa. The advantage of using fondaparinux is a lack of interaction with platelets or platelet factor 4. Therefore fondaparinux, unlike UFH or LMWH, does not induce HIT.75,76 The potential problem with the use of this drug is the relative longer half-life and lack of a reversible agent for utilization in the setting of bleeding, although the risk of such an event does not seem to be increased compared with LMWH. Although no antidote is known, high doses of recombinant factor VIIa (90 μg/kg) have been shown to partially normalize the prolonged aPTT in healthy volunteers who received therapeutic doses of fondaparinux.77 In the randomized, double-blind Matisse DVT trial, 2205 patients were treated with a once-daily SC dose of fondaparinux or twice-daily SC LMWH, enoxaparin, for at least 5 days. No difference in recurrent VTE, major bleeding during treatment, or death at 3 months was observed.78 Therefore, once-daily fondaparinux appears to be at least as effective and safe as twice-daily body weight–adjusted enoxaparin for the initial treatment of patients with symptomatic DVT.
Other options for the treatment of DVT include placement of inferior vena cava (IVC) filters thrombolysis or thrombectomy. A large, randomized controlled trial looked at the usage of permanent IVC filter insertion as an adjunct to anticoagulation treatment in patients with an acute DVT who were considered to be at high risk for PE. The study showed that at 8 years, IVC filters reduced the risk of PE but increased that of DVT and had no effect on survival.79 In another trial, 400 patients with proximal DVTs were randomly assigned to receive either standard anticoagulation alone or anticoagulation plus insertion of an IVC filter. Although only 1% of patients in the latter group experienced PE compared with 5% of the former (anticoagulation only) group at 12 days after randomization, there were no significant differences in survival or symptomatic PE between the two groups at 2 years of follow-up. Moreover, patients who received an IVC filter had a significantly higher rate of recurrent DVT.79 Thus, in high-risk patients with proximal DVT, the initial beneficial effect of vena caval filters for prevention of PE was counterbalanced by an excess of recurrent DVT, without any difference in mortality. Other rare complications of IVC filter placement include perforation, misplacement, and migration. Currently, the only indication for an IVC filter in the setting of an acute VTE is in patients who have an absolute contraindication to anticoagulant therapy (e.g., active bleeding, recent surgery, hemorrhagic stroke), those who have recurrent VTE despite adequate anticoagulation, and in those patients who have extremely limited pulmonary vascular reserve such that another thromboembolic event might be fatal. Based on current data, insertion of an IVC filter as an adjunct to anticoagulation in the general population with VTE is not indicated. Retrievable filters that may be used instead of a permanent IVC filter are available. A systematic review identified six prospective case series with broad ranges of indications for filters. In these case series, four different types of retrievable filters were inserted. Among patients in whom percutaneous removal of the filter was attempted, the filter was successfully removed in 91%. Surgery was necessary to remove the filter from 1%, and filters could not be removed because of large trapped thrombi in 9%.80 Although filters may be an option in patients who have a transient contraindication to anticoagulation, more studies are needed to establish the benefits of retrievable filters over permanent ones.
In the past, immobilization was recommended by some as part of the treatment of DVT. Studies have shown faster resolution of pain and swelling with early ambulation and leg compression compared with immobilization, with similar incidences of new PE after 10 days of treatment in both groups.81,82,83,84 Therefore, early ambulation with anticoagulation has replaced treatment of acute DVT with bedrest and anticoagulation.
Even though there might be more rapid and complete lysis of DVT and less postphlebitic syndrome86,86 associated with the use of thrombolytic therapy, it is seldom used in patients with DVT. This lack of enthusiasm for the usage of thrombolytics may be because thrombolytics increase the bleeding risk and the clinical relevance of achieving earlier relief of venous obstruction is not clear. In a meta-analysis comparing streptokinase as a thrombolytic versus UFH, streptokinase was associated with a 3.7 (CI, 2.5–5.7; P < .0001) and 2.9-fold (CI, 1.1–8.1; P = .04) increase in the extent of thrombolysis and major bleeding, respectively.87 The risk of death and early recurrence in patients with DVT is low if an appropriate dose of anticoagulant is started promptly.88 Currently, the most common scenario for thrombolytic therapy for DVT is the presence of a massive proximal lower extremity or iliofemoral thrombosis associated with limb-threatening ischemia (phlegmasia cerulea dolens) or severe symptomatic swelling.88 The major risks of acute iliofemoral venous thrombosis are PE and limb loss from reduced peripheral perfusion. Approximately 40% to 50% of patients presenting with iliofemoral venous thrombosis have scintigraphic evidence of pulmonary emboli, which are asymptomatic in most instances. Hence, studies have been done to assess if thrombolysis or thrombectomy would reduce the risk of these events over using only anticoagulation therapy.
In the short term, patients treated with catheter-directed thrombolysis (CDT) may obtain better patency and competence than those treated with standard anticoagulation.89 A systematic review suggested that CDT is riskier than anticoagulant therapy on the basis of an increased risk of major hemorrhage (11.3% versus 5.9%).90 Furthermore, a decision analysis suggested that patients are not willing to risk potential adverse outcomes associated with thrombolytic therapy to prevent postthrombotic syndrome (PTS).86 Thrombolytic therapy in patients with DVT therefore remains controversial. Thrombolytics are currently reserved for patients with limb-threatening thromboses with a short duration of symptoms and a low risk of bleeding.91 These patients include those with an iliofemoral DVT who have had symptoms for less than 14 days, have good functional status, and have a life expectancy of more than 1 year.49 After successful CDT in patients with an acute DVT, correction of the underlying venous lesions using balloon angioplasty and stents is recommended.49 Systemic thrombolytic therapy may be used to reduce acute symptoms and post-thrombotic morbidity only if CDT is not available.49 The ACCP guidelines recommend using CDT over operative venous thrombectomy in low bleeding risk patients. Operative venous thrombectomy may be considered in a select group of high bleeding risk patients if appropriate expertise and resources are available.49 Only small retrospective studies have investigated percutaneous mechanical venous thrombectomy as a modality to remove thrombus, and the therapeutic efficacy of this approach has been inconclusive.92 Thrombolytic therapy as an intervention is fraught with considerable risks and potential lack of long-term benefits. An acknowledgment of the absence of high-quality supportive data must be discussed with the patient before using thrombolytics in this setting.93
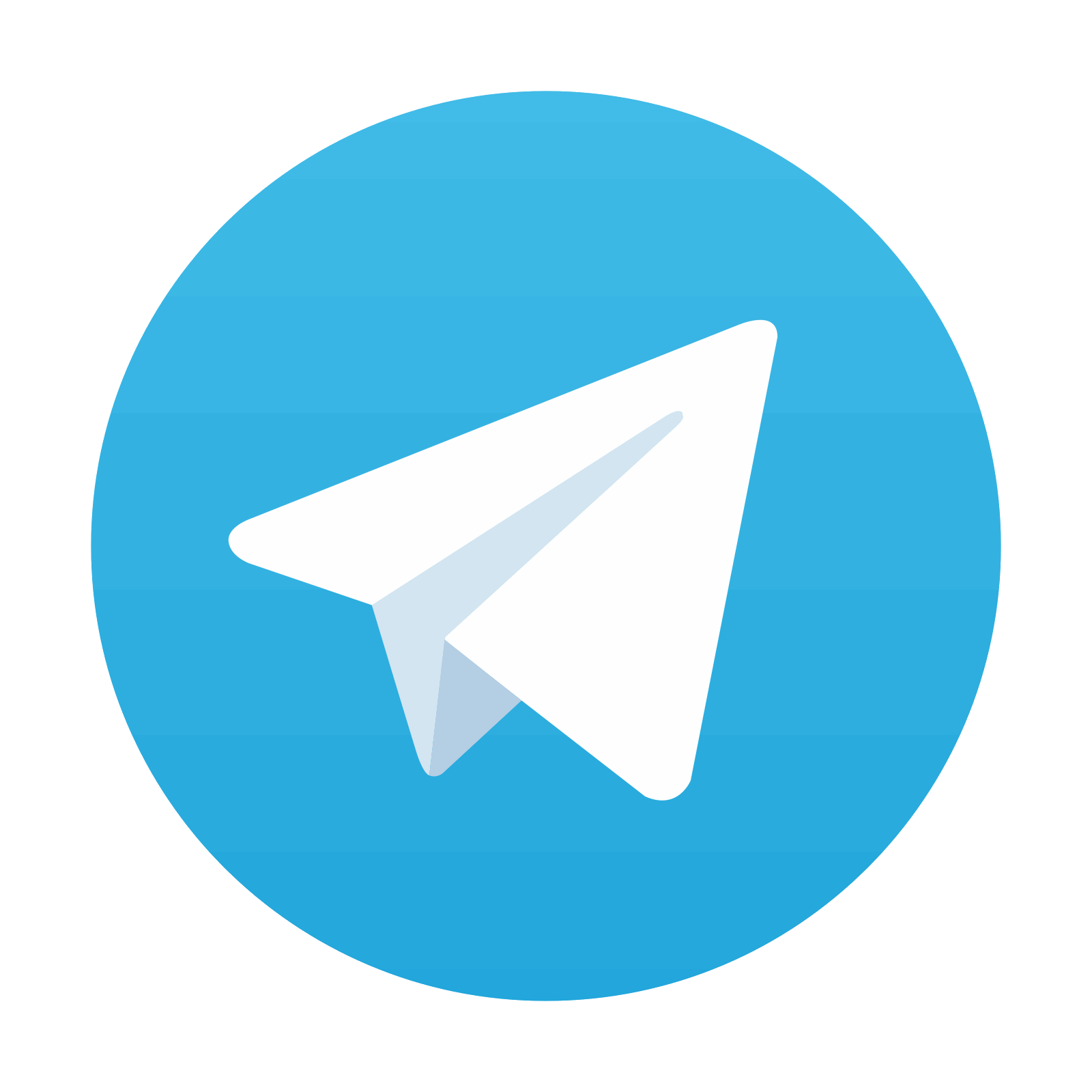
Stay updated, free articles. Join our Telegram channel

Full access? Get Clinical Tree
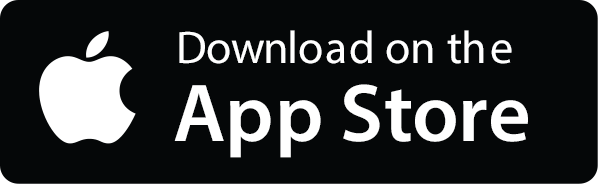
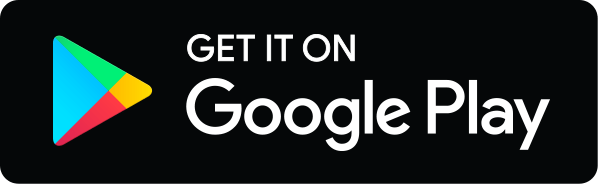