(1)
Project-team INRIA-UPMC-CNRS REO Laboratoire Jacques-Louis Lions, CNRS UMR 7598, Université Pierre et Marie Curie, Place Jussieu 4, Paris Cedex 05, France
Abstract
Protein phosphorylation is a common, reversible, post-translational modification of proteins (Vols.1 – Chap. 5. Protein Synthesis and 3 – Chap. 1. Signal Transduction). Except pseudophosphatases, phosphatases removes a phosphate group from their substrates. They thus antagonize kinases that attach phosphate groups to Ser, Thr, and Tyr residues in the same substrates using ATP. Phosphorylated residues SerP, ThrP, and TyrP account for about 86, 12, and 2% of the phosphoproteome, respectively. Mammalian genomes encode about 100 protein Tyr kinases and phosphatases, and about 100 and about 25 protein Ser/Thr kinases and phosphatases.
Protein phosphorylation is a common, reversible, post-translational modification of proteins (Vols.1 – Chap. 5. Protein Synthesis and 3 – Chap. 1. Signal Transduction). Except pseudophosphatases, phosphatases removes a phosphate group from their substrates. They thus antagonize kinases that attach phosphate groups to Ser, Thr, and Tyr residues in the same substrates using ATP. Phosphorylated residues SerP, ThrP, and TyrP 1 account for about 86, 12, and 2% of the phosphoproteome, respectively. Mammalian genomes encode about 100 protein Tyr kinases and phosphatases, and about 100 and about 25 protein Ser/Thr kinases and phosphatases.
Protein phosphatases can be grouped into 3 main categories according to their structure and catalytic function. The largest category of protein phosphatases corresponds to the superfamily of classical, cytosolic, large, phosphoprotein SerP/ThrP phosphatase (PP1–PP7)2 that includes the families of phosphoprotein phosphatase (PPP) and Mg
– and/or Mn
-dependent protein phosphatases (PPM1α–PPM1ε, among others).3 Protein TyrP phosphatases and aspartate-based protein phosphatases constitute a second and a third category (Tables 8.1 and 8.2).


Table 8.1
Categories of human protein phosphatases and examples of functions (Part 1) Subclass of protein Ser/Thr phosphatases (Source: [641]; NFκB: nuclear factor-κB; PP: protein phosphatase; PPM: protein phosphatase, Mg
or Mn
dependent; TGFβ: transforming growth factor-β). The PPP family can be subdivided into PP1, PP2 (including PP4 and PP6), PP3, and PP5 subfamilies.


Type | Role |
---|---|
Subclass of protein Ser/Thr phosphatases | |
PPP superfamily | |
PPP family | |
PP1 | Chromosome condensation |
PP2 | Chromatid cohesion |
PP3 | Immune response |
PP4 | DNA repair |
PP5 | Cell stress |
PP6 | NFκB signaling |
PP7 | |
PPM family | |
PPM1 | TGFβ signaling |
Table 8.2
Categories of human protein phosphatases and examples of functions (Part 2) Subclass of protein Tyr phosphatases (PTP; Source: [641]; AcP1: acid phosphatase-1, soluble [or lmwPTP]; DuSP: dual specificity phosphatase; EyA: Eye absent; FCP: TF2F-associating C-terminal domain phosphatase; HAD, haloacid dehalogenase; lmwPTP: low-molecular-weight protein phosphotyrosine phosphatase; MAPK: mitogen-activated protein kinase; MKP: MAPK phosphatase; MTM: myotubularin; NRPTP: non-receptor [cytosolic] protein Tyr phosphatase; PI: phosphoinositide (phosphorylated phosphatidylinositol); PIP3: phosphatidylinositol trisphosphate; PRL: phosphatase of regenerating liver; PTen: phosphatase and tensin homolog; RPTP: receptor protein Tyr phosphatase; SCP: small C-terminal domain-containing phosphatase; Ssh: Slingshot; TGFβ: transforming growth factor-β).
Type | Role |
---|---|
Subclass of protein Tyr phosphatases | |
Infraclass-1 of Cys-based PTPs | |
Hyperfamily of classical PTPs | |
RPTP superfamily | |
RPTP | Cell adhesion, cytoskeleton organization |
NRPTP superfamily | |
PTPn | Insulin signaling |
Hyperfamily of DuSPs | |
Atypical DuSP | |
CDC14 | Cytokinesis |
MKP | MAPK signaling |
MTM | PI metabolism |
PTP14a (PRL) | |
PTEN | PIP3 dephosphorylation |
Ssh | Actin dynamics |
Infraclass-2 of Cys-based PTPs | |
CDC25 | Mitosis |
Infraclass-3 of Cys-based PTPs | |
AcP1 (lmwPTP) | |
Infraclass of Asp-based PTPs – FCP Category | |
FCP1 | Transcription |
SCP | TGFβ signaling |
FCPL | |
HAD | Actin dynamics |
Classical protein TyrP phosphatases are subdivided in receptor-like, plasmalemmal PTPs and cytosolic (non-receptor) PTPs. Both agonists and antagonists bind to, at least, some PTPRs (e.g., PTPRf, PTPRj, and PTPRt). In addition, some PTPRs (e.g., RPTPa) are inhibited by dimerization.
8.1 Pseudophosphatases
Protein MAP3K7IP1, or transforming growth factor-β-activated kinase-1 (TAK1)-binding protein-1 (TAB1), is one of the regulatory subunits of MAP3K7 (TAK1), an effector kinase of pro-inflammatory cascades. Molecule MAP3K7IP1 possesses a fold closely related to that of Mg
– or Mn
-dependent protein phosphatase family member PPM1α (Sect. 8.3.8), but without phosphatase activity, as several key residues required for dual metal-binding and catalysis are absent [642]. It serves as an adaptor that binds to and regulates accessibility of phosphorylated residues on substrates downstream of MAP3K7 or on the MAP3K7–MAP3K7IP1 complex.


The myotubularin family of dual-specificity phosphatases (Sect. 8.3.13.7) comprises many pseudophosphatases, such as MTMR5 and MTMR13. The latter indeed contain alterations in their PTP homology domains that abrogate phosphatase activity. Phosphatases that are catalytically inactive are able to bind phosphorylated substrates and prevent their dephosphorylation. Therefore, myotubularin-related pseudophosphatases can trap mutants or regulate PI3P levels by opposing the actions of myotubularin phosphatases. Truncated forms of MTMR5 are oncogenic, as they deal with cellular growth and oncogenic transformation.
8.2 Catalytically Active Protein Phosphatases
Protein phosphatases form a group of signal transduction enzymes that, together with protein kinases, control the level of cellular protein phosphorylation. Kinase–phosphatase cascades are characterized by protein–phosphoprotein cycles that allow negative and positive commands and feedback in a signaling pathway.
Protein phosphatases dephosphorylate: (1) phospho-serine (SerP) and phospho-threonine (ThrP) in the cell or (2) phospho-tyrosine (TyrP), either exclusively or with ThrP at the plasma membrane or in the cytoplasm. Both protein Tyr and Ser/Thr dephosphorylations contribute to cell growth, proliferation, and differentiation, as they turn off or switch on actuating signaling.
Protein phosphatases mainly act on proteins, but certain protein phosphatases are able to remove phosphate from either phospholipids or mRNA. Inositide phosphatases SHIP and synaptojanins dephosphorylate inositol phospholipids (Chap. 2). SH2 domain-containing inositol 5-phosphatase SHIP1 (or IP(5)Pd) binds to Tyr residues of numerous substrates. It participates in cytoskeleton rearrangement for cell mobilization.
Dual-specificity protein phosphatases (DSP or DuSP) can dephosphorylate proteins on TyrP, SerP, and ThrP, in addition to signaling lipids and complex carbohydrates.
Protein Tyr phosphatases (PTP) and kinases (PTK) act in partnership, as they coordinate their activities to control cell signaling. Protein Tyr phosphatases dephosphorylate TyrP residues selectively on their substrates to initiate, sustain, or terminate signal transduction. Both PTP catalytic and non-catalytic domains contribute to substrate specificity. In general, protein phosphatases have less substrate specificity than protein kinases.
The pathway from the platelet-derived growth factor receptor (PDGFR, Vol. 3 – Chap. 8. Receptor Kinases) to mitogen-activated protein kinases ERK1 and ERK2 that involves Ras, Raf, and MAP2K, illustrates the kinase–phosphatase control of signaling (Fig. 8.1).

Fig. 8.1
Kinase–phosphatase cascade induced by stimulation of the platelet-derived growth factor receptor (PDGFR) associated with a balance between phosphorylation (activation) and dephosphorylation (inhibition; Source: [643]). MKP is a flexibility node of the pathway, modulating the cascade effect. Control of the Ras–ERK cascade needs the plasmalemmal recruitment of proteins serving as adaptors, anchors, and scaffolds (GRB, and SHC), and as regulators (SOS, and RasGAP). These complexes can be activated at the plasmalemma or in the endosomes, where they are able to recruit signaling molecules to trigger biological responses. ERK1/2 activates cytoplasmic phospholipase-A2 (PLA2), which produces arachidonic acid (AA). The latter stimulates protein kinase-C (PKC) via diacylglycerol (DAG). PKC in turn activates Raf (positive feedback loop). Receptor tyrosine kinases activate other effectors such as phospholipase Cγ, which stimulates PKC. Protein phosphatase-2 (PP2) dephosphorylates both Raf and mitogen-activated protein kinase kinase (MAP2K). Mitogen-activated protein kinase phosphatase (MKP) dephosphorylates ERK1/2. MAPK phosphorylates MKP, reducing MKP degradation (negative feedback).
8.3 Cytosolic Protein Serine/Threonine Phosphatases
Protein Ser/Thr phosphatases constitute many subfamilies associated with PP1 (α, β, γ1, and γ2), PP2 (PP2a), PP3 (PP2b), and PP4 to PP7 enzymes. Protein phosphatases PP1 and PP2 account for more than 90% of all Ser/Thr phosphatase activities in most cells (Table 8.3).
Table 8.3
Protein Ser/Thr phosphatases PP1, PP2 (PP2a), PP3 (PP2b), and PPM1 (PP2c), are the most abundant phosphatases. The number of genes that encode catalytic subunits is rather small ( ∼ 40 genes). These subunits have much lower specificity for their substrates than Ser/Thr kinases, but they associate with numerous regulatory subunits that determine their substrate specificity, subcellular location, and overall activity. In addition to association with diverse types of regulatory subunits, phosphatases can be further regulated by phosphorylation, methylation, and ubiquitination.
Type | Features |
---|---|
PP1 | Magnesium-dependent |
Numerous regulatory subunits | |
Intervention in glygogen metabolism, | |
Ca ![]() | |
protein synthesis, cell division | |
PP2 | Metal ion-independent |
Numerous regulatory subunits | |
Heterodimer or heterotrimer | |
Regulation of cell division, apoptosis | |
Regulation of TOR and Wnt signaling | |
Stabilization of β catenin–E-cadherin complex | |
Regulation of CamK2, CDK, ERK, IKK, PKA/B/C, S6K | |
Action in cytoskeleton restructuring | |
PP3 | Active as heterodimer |
Calcium-dependent | |
Stimulation by calmodulin | |
Regulation of NFκB, TNFα, TGFβ | |
Regulation of CamK2, MAPK | |
T-lymphocyte activation | |
PPM1 | Monomer |
Magnesium- and manganese-dependent | |
Encoded by at least 10 genes |
Among Ser/Thr phosphatases, chronophin regulates phosphorylation of cofilin, an actin regulator (Sect. 8.3.16.3). Actin monomers and filamentous actin are cyclically exchanged. Incorporation of actinATP into filaments (Factin) results from ATP hydrolysis. Recycling factors, such as cofilin and related actin-depolymerizing factors (ADF), bind actinADP of filaments to promote filament depolymerization. Filament severing simultaneously stimulates actin filament disassembly and elicits actin polymerization on newly-severed barbed ends, thereby enhancing the dynamic exchange of between Gactin and Factin. Interactions between cofilin and ADF on the one hand and actin on the other are improved by dephosphorylation by ubiquitous chronophin that thus assists cofilin-mediated actin organization. Chronophin counteracts LIMK kinase that inactivates actin-depolymerizing protein cofilin. Chronophin, or pyridoxal phosphatase, also dephosphorylates pyridoxal 5′-phosphate (vitamin-B6).
During mitotic entry, the regulation of phosphatases governs CDK1 activation. Conversely, after satisfactory spindle assembly checkpoint,4 i.e., proper chromosome segregation, cytokinesis, and reassembly of interphase cell structures, mitotic exit is achieved, at least partly, by dephosphorylations of diverse substrates, hence inactivation of mitotic kinases, particularly CDK1, PLK1, and Aurora kinases, and activation of counteracting phosphatases, especially PP1 and PP2 phosphatases [644] (Table 8.4). A regulatory axis that involves Greatwall kinase and its substrates, the PP2-inhibitors α-endosulfine and cAMP-regulated phosphoprotein ARPP19, generates a mutual inhibition between cyclin-dependent kinase CDK1 and PP2 phosphatase.5
Table 8.4
Major mitotic kinases and phosphatases and their phases of activity during the cell division cycle, especially mitotic entry, progression, and exit (Source: [644]; CDC: cell division cycle phosphatase; CDK: cyclin-dependent kinase; CenPe: centromere-associated protein-E; PKMYT: membrane-associated Tyr–Thr protein kinase; PLK: Polo-like kinase; PNuTS: phosphatase-1 nuclear targeting subunit).
Enzyme | Cell cycle phase of activity |
---|---|
(location) | |
Kinases | |
CDK1–CcnB | Prophase (nucleus), |
prometaphase, metaphase (cytoplasm, spindle) | |
Wee1, PKMYT1 | G1, G2 |
Greatwall | Prophase, prometaphase, metaphase |
Aurora-B | Prophase, prometaphase, metaphase (centromeres), |
anaphase, telophase (spindle) | |
PLK1 | G2, |
prophase, prometaphase, metaphase | |
(kinetochores, centromeres), | |
anaphase, telophase (spindle) | |
Phosphatases | |
CDC14a | G1/2 (cytoplasm, centrosome), |
prophase→telophase (cytoplasm) | |
CDC14b | G1/2 (nucleus, centrosome), |
prophase→metaphase (cytoplasm) | |
anaphase, telophase (spindle) | |
CDC25b/c | Prophase→metaphase |
PP1–CenPe | Prophase→metaphase (kinetochores) |
PP1–PNuTS | Anaphase, telophase (chromosomes), G1 |
PP2–PP2r2 | G1/2, anaphase, telophase |
PP2–PP2r5 | G1/2, |
prophase→metaphase (kinetochores) | |
anaphase, telophase (spindle) |
8.3.1 Protein Phosphatase-1
Ubiquitous cytosolic (non-receptor) Ser/Thr protein phosphatase-1 (PP1) regulates various cellular processes, in particular the glycogen metabolism, transcription, cell polarity, vesicle transfer, the DNA damage response, and cell cycle progression. It dephosphorylates multiple substrates and connects to numerous proteins. It promotes more energy-efficient fuels when nutrients abound and stimulates the storage of energy in the form of glycogen. In addition, it also enables the relaxation of actomyosin fibers as well as the return to a basal pattern of protein synthesis, and the recycling of transcription and splicing factors [645]. It also intervenes in the recovery from stress, promotes the exit from mitosis and apoptosis when cells are damaged beyond repair, and downregulates ion pumps and transporters in various cell types as well as ion channels in neurons.6
The PP1 catalytic subunits are encoded by 3 genes (PP1A, PP1B, or PP1D, and PP1G). They form stable dimers with numerous regulatory subunits that determine substrate specificity, subcellular location, and phosphatase activity. In addition to association with diverse types of regulatory subunits, these phosphatases can be further regulated by post-translational modifications, such as phosphorylation, methylation, and ubiquitination.
The diverse forms of protein phosphatase-1 result from association of a catalytic subunit (PP1c) and different regulatory subunits (Table 8.5). The catalytic subunits of PP1 actually do not exist freely in the cell, but associate with regulatory polypeptidic interactors to form various holoenzymes. These regulatory subunits allow targeting of various subcellular locations and modulating connection to stimulators and effectors. The PP1c target depends on the interaction between the regulatory and catalytic subunits that form the PP1 complex. Three isoforms of PP1 catalytic subunit exist.
Table 8.5
Subunits of protein phosphatase-1 (PPP: phosphoprotein phosphatase; Source: [76]). In humans, about 180 PP1-interacting proteins have been identified.
Subunit(s) | Gene(s) |
---|---|
Catalytic subunits | |
PP1cα | PPP1CA |
PP1cβ (PP1cδ) | PPP1CB |
PP1cγ | PPP1CG (PPP1CC) |
PP1cγ1–PP1cγ2 (alternatively spliced variants) | |
Regulatory subunits (inhibitors) | |
PP1r1a–PP1r1b | PPP1R1A, PPP1R1B |
PP1r2 | PPP1R2 |
PP1r3a–PP1r3f | PPP1R3A–PPP1R3F |
PP1r7 | PPP1R7 |
PP1r8 | PPP1R8 |
PP1r9a–PP1r9b | PPP1R9A–PPP1R9B |
PP1r10 | PPP1R10 |
PP1r11 | PPP1R11 |
PP1r12a–PP1r12c | PPP1R12A–PPP1R12C |
PP1r13b, PP1r13L | PPP1R13B, PPP1R13L |
PP1r14a–PP1r14d | PPP1R14A–PPP1R14D |
PP1r15a–PP1r15b | PPP1R15A–PPP1R15B |
PP1r16a–PP1r16b | PPP1R16A, PPP1R16B |
Interactors of PP1 include regulatory subunits and possible other regulators as well as other partners that may be regulators and/or substrates, such as phosphofructokinase, retinoblastoma protein, and Src-like adaptor SLA1 (Tables 8.6 to 8.8). Phosphatase PP1 associates with about 200 regulatory proteins to form highly specific holoenzymes (active complex with apoenzyme bound to its cofactor), thereby achieving target specificity. These regulatory proteins direct PP1 to its action locus within the cell as well as its enzymatic specificity for particular substrates. Spinophilin, or neuronal PP1 regulatory (inhibitory) subunit PP1r9b, binds PP1 and blocks a substrate binding site without altering its active site [646]. In fact, Ser/Thr protein phosphatase-1 dephosphorylates hundreds of substrates.
Table 8.6
Interactors of PP1 (Part 1; Source: [645]; AKAI: A-kinase-activated inhibitor; AnkRD: Ankyrin repeat domain-containing protein; ARD: activator of RNA decay; ASPP: apoptosis-stimulating protein of P53; CPI17: 17-kDa (PK)C-kinase-dependent phosphatase inhibitor; DARPP32: 32-kDa dopamine and cAMP-regulated protein; GADD: growth arrest and DNA damage-inducible protein; HCG5: hemochromatosis candidate gene-5 product; KEPI: kinase-enhanced PP1 inhibitor; MyPT: myosin phosphatase target subunit; NIPP1: nuclear inhibitor of PP1; PHI: phosphatase holoenzyme inhibitor; PNuTS: phosphatase-1 nuclear targeting subunit; TIMAP: TGFβ-inhibited membrane-associated protein; TP53BP: transcription factor P53-binding protein). G Subunits of PP1 have binding sites for glycogen and both PP1 and its substrates.
Interactors | Alias(es) |
---|---|
PP1r1b | PP1 inhibitor-1, DARPP32, AKAI |
PP1r7 | SDS22 |
PP1r8 | NIPP1, ARD1 |
PP1r9a∕b | Neurabin-1/2, spinophilin (subunit-9B) |
PP1r10 | PNuTS |
PP1r11 | HCG5 |
PP1r12a∕b | MyPT1/2 (MLCP) |
PP1r13 | ASPP1 |
TP53BP2 | ASPP2 |
PP1r14a | CPI17L |
PP1r14b | PHI1 |
PP1r14c | KEPI |
PP1r15a | GADD34 |
PP1r16a | MyPT3 |
PP1r16b | AnkRD4, TIMAP |
G (glycogen) targeting subunits | |
PP1r3a | GM (striated muscle) |
PP1r3b | GL (liver) |
PP1r3c | Ubiquitous GC |
PP1r3d | Ubiquitous GD |
PP1r3e | Ubiquitous GE |
PP1r3f | Ubiquitous GF |
PP1r3g | Ubiquitous GG |
Table 8.7
Interactors of PP1 (Part 2; Source: [645]; AKAP: A-kinase-anchoring protein; BCL: B-cell lymphoma; CGNAP: centrosome and Golgi-localized PKN-associated protein; NeK: NIMA (never in mitosis gene-A)-related protein kinase; NKCC: Na + –K + –Cl − cotransporter; NLK: NIMA-like protein kinase).
Interactors | Alias(es) |
---|---|
AKAP1 | AKAP149 |
AKAP9 | AKAP350, AKAP450, CGNAP, Yotiao |
AKAP11 | AKAP220 |
NKCC1 | SLC12a2 |
Vitamin-D receptor | NR1i1 |
Aurora-A and -B | STK6/7/15 and STK5/12 |
FAK | PTK2 |
NeK2 | NLK1 |
Phosphofructokinase | |
Protein kinase-R | eIF2αK2 |
BCL2, BCL2L2, BCLxL | |
Retinoblastoma protein |
Table 8.8
Interactors of PP1 (Part 3; Source: [645]; CMT: Charcot-Marie-Tooth disease; FAK: focal adhesion kinase; GRP78: 78-kDa, endoplasmic reticulum luminal Ca
-binding glucose-regulated protein; HCFc1: host cell factor-C1; Hox: homeodomain-containing (homeobox) protein; HSPa5: heat shock 70-kDa protein-5; InI: integrase interactor; NCoR, nuclear receptor corepressor; NPWBP: 38-kDa nuclear protein containing a WW domain-binding protein; PSF: polypyrimidine tract-binding protein-associated splicing factor; SARA: SMAD anchor for receptor activation; SNF: sucrose non-fermenting; SFPQ proline and glutamine-rich splicing factor; SIPP1: splicing factor that interacts with PQBP1 and PP1; SMARC: SWI/SNF-related, matrix-associated, actin-dependent regulator of chromatin; SNP70: 70-kDa SH3 domain-binding protein; Stau: Double-stranded RNA-binding protein Staufen homolog; TLX: T-cell leukemia homeobox; VCAF: VP16 accessory protein; WBP: WW domain-binding protein; ZFYVE: zinc finger, FYVE domain-containing protein).

Interactors | Alias(es) |
---|---|
PCdh7 | BH (brain–heart)-protocadherin |
HSPa5 | GRP78 |
HCFc1 | VCAF |
TLX1 | Hox11 |
NCoR | |
Neurofilament-L | CMT1f, CMT2e |
Ribosomal protein-L5 | |
SFPQ | PSF |
SMARCb1 | INI1, SNF5 |
Stau1 | |
Tau | |
Trithorax | |
WBP11 | NPWBP, SNP70, SIPP1 |
ZFYVE16 | Endofin, SARA |
In addition to cytosolic forms of PP1, such as those that target glycogen and myosin, nuclear PP1 exist [641].
Protein phosphatase-1 contains binding domains for hepatic and muscle glycogen and smooth muscle myosin that assign the catalytic subunit to these substrates [647]. The hepatic glycogen-binding domain has 2 binding subdomains, one that suppresses the dephosphorylation of glycogen phosphorylase, another that enhances the dephosphorylation of glycogen synthase.
Protein phosphatase-1 modulates functioning of some membrane proteins, such as ion carriers and receptors, and participates in various cellular processes (Tables 8.9 and 8.10). Subtype PP1γ dephosphorylates histone-3 (Thr11) after DNA damage for transcriptional repression of cell cycle regulatory genes [648].
Table 8.9
Role of protein phosphatase-1 (Source: [645]; Part 1; ↓: decrease). Phosphatase PP1 operates as an economizer, reducing energy expenditure, and a reset element (protein recycling, actomyosin relaxation, ion carrier inactivation, and exit from mitosis).
Cell process | Antagonist kinases, targets, and effects |
---|---|
Ion transport | IP3R1, RyR2; conductance ↓; |
phospholamban; SERCA activity ↓; | |
Na + –K + ATPase ↓; | |
KV7.1; conductance ↓; | |
ClC2 activation; NKCC1 inactivation | |
Cell signaling | Recruitment of PP1 to TβR1 by ZFYVE9 |
(antagonizes TβR2) | |
Cell metabolism | AMPK; glucose import; gluconeogenesis; |
use of alternative sugar and other carbon sources | |
(fatty acids, glycerol, pyruvate, and lactate); | |
repression of anabolism | |
Glycogen metabolism | Storage of glycogen |
Protein synthesis | CREB inhibition; |
SMARCb1 — chromatin remodeling; | |
eIF2αK2, RPL5 — translation; | |
SFPQ — mRNA processing; | |
recycling of transcription and splicing factors; | |
return to basal state of protein synthesis |
Cell process | Antagonist kinases, targets, and effects |
---|---|
Actin remodeling | PP1r9–PP1, PP1r12–PP1; |
actomyosin cytoskeleton dephosphorylation; | |
stress fiber relaxation | |
Cell division | Aurora — histone-3 dephosphorylation, |
chromosome decondensation | |
NeK2 — delay of centrosome splitting | |
Lamin-B — reassembly of the nuclear envelope | |
Cell cycle arrest | Retinoblastoma protein activation |
Cell survival or | BCL2, BCL2L2, BCLxL |
apoptosis | PP1–BCL2/2L2/xL–BAD complex; |
supports apoptosis of damaged cells |
The rate-limiting enzymes of glycogen anabolism and catabolism7 are glycogen synthase and phosphorylase, respectively. Phosphorylation of glycogen synthase by several kinases and of glycogen phosphorylase by phosphorylase kinase causes inactivation and activation, respectively. Phosphorylase kinase is activated by phosphorylation of its regulatory α and β subunits and by Ca
binding to the regulatory δ subunit. Glycogen synthase and phosphorylase as well as phosphorylase kinase are dephosphorylated by PP1 anchored to glycogen via glycogen-targeting (G) subunits [645].

Phosphorylation of cAMP-responsive element-binding protein (CREB) by PKA promotes the recruitment of histone acetyltransferase CBP to facilitate access of the promoter by the transcriptional machinery. Conversely, CREB action is reduced upon CREB dephosphorylation by PP1 enzyme [645].
In human cells, during mitosis, PP1 controls the attachment of spindle microtubules to kinetochores during metaphase, as they counteract Aurora-B-mediated phosphorylation of outer kinetochore components and microtubule-destabilizing factors [644]. The kinetochore protein KNL1 (kinetochore-null protein-1),8 PP1r7 regulatory subunit, and kinetochore nanomotor CenPe bind PP1 and target PP1 to kinetochores. Conversely, active Aurora-B impedes PP1 linkage to kinetochores, as it phosphorylates the PP1-binding motif of KNL1 and CenPe proteins. The mutual control between Aurora-B and PP1 can establish a switch in substrate phosphorylation state at the outer kinetochore, thereby enabling a fast response to errors in chromosome attachment. Enzyme PP1 also opposes Aurora-B phosphorylation on chromosome arms (Ser10 on histone-3).
Protein phosphatase PP1 interacts with PP3 [649]. In addition, PP3 can dephosphorylate PP1 inhibitors such as DARPP32, hence activating PP1.
8.3.1.1 Examples of Protein Phosphatase-1 Regulatory Subunits
Protein Phosphatase-1 Inhibitory Subunit-1B
Protein phosphatase-1 regulatory (inhibitory) subunit PP1r1b 9 that is a mediator of dopaminergic signaling. It is involved in cell differentiation as well as morphogenesis and functioning of several organs. Its effect depends on phosphorylation state of 4 regulatory residues (Thr34, Thr75, Ser102, and Ser137) [650].
Phosphorylation by protein kinase-A (Thr34) converts PP1r1b into a potent, high-affinity inhibitor of PP1 phosphatase. Residue Thr34 is dephosphorylated by protein phosphatase PP3 (or calcineurin). Residue Thr75 that can inhibit PKA effect is phosphorylated by cyclin-dependent kinase CDK5 and dephosphorylated by PP2 phosphatase. Residue Ser102 that is phosphorylated by casein kinase CK2 increases efficiency of Thr34 phosphorylation. Residue Ser137, once phosphorylated by casein kinase CK1, is able to decrease the activity of protein phosphatase PP3 [650]. Residue Ser137 is dephosphorylated by protein phosphatases PPM1 (PP2c). In summary, PP1r1b is inhibited when phosphorylated at Thr34, whereas PKA phosphorylation of Thr34 is prevented by Thr75 phosphorylation.
Transcription of the PPP1R1B gene is controlled by cAMP response element-binding protein. When PP1 is inhibited, cAMP response element-binding protein remains phosphorylated until PP1r1b is inactivated. Several agents (cocaine, morphine, ethanol, nicotine, methamphetamine, estradiol, etc.) as well as dopamine, other endogenous neurotransmitters, and hormones (serotonin, glutamate, GABA, adenosine, nitric oxide, opioids, neurotensin, cholecystokinin, and human chorionic gonadotropin) modulate PP1r1b phosphorylation state. Brain-derived neurotrophic factor binds to neurotrophic Tyr receptor kinase NTRK2 to activate protein kinase-B that phosphorylates CREB (Ser133).
Protein Phosphatase-1 Inhibitory Subunit-8
Protein phosphatase-1 regulatory (inhibitory) subunit-8 (PP1r8)10 is encoded by the PPP1R8 gene. It contributes to adaptation to hypoxia. This ubiquitous RNA-binding protein is required in pre-mRNA processing during a late step of spliceosome assembly [651]. In addition, it operates as a transcriptional repressor.
The PP1r8–PP1 holoenzyme can be phosphorylated (activated) by protein kinase-A (Ser178 and Ser199) and, less effectively, by casein kinase-2 (Thr161 and Ser204) as well as Lyn (Tyr264 and Tyr335).
Protein Phosphatase-1 Inhibitory Subunit-12 – MLCP
Phosphorylation of myosin regulatory light chain is a component of the regulation of cell contraction. Indeed, MLC phosphorylation status results from the balance of activity of MLC kinase (activation) and phosphatase (inactivation). Dephosphorylation causes Ca
desensitization, especially in smooth muscle cells, and reduces ability of myosin to crosslink actin filaments.

Myosin light-chain phosphatase (MLCP) is composed of 3 subunits: (1) a catalytic motif (PP1cδ); (2) a large myosin-binding sequence (MBS), i.e., either the protein phosphatase-1 regulatory (inhibitory) subunit-12A (PP1r12a), also called myosin light-chain phosphatase target subunit-1 (MyPT1), or -12B (PP1r12b), also termed MyPT2; and (3) a small subunit such as (20-kDa) smM20 protein, which elevates the Ca
sensitivity of contractile fibers in vascular smooth muscle cells.

Subunit PP1r12a is ubiquitous, whereas PP1r12b mainly lodges in the brain and striated muscles. Both PP1r12a and PP1r12b can complex with PP1cδ as well as other molecules, such as moesin and microtubule-associated MAP2 and Tau (MAPT) proteins. Moreover, PP1r12a and PP1r12b act as scaffolds of substrates for several kinases and signaling proteins such as RhoA GTPase [652]. Four alternatively spliced transcript variants can be described; 2 associated with the MBS subunit and 2 with smM20 subunit.
In fact, the PPP1R12B gene encodes both the large MBS and small smM20 subunit. The MBS subunit is also encoded by the PPP1R12A gene. Small smM20 subunit has a higher binding affinity for PP1r12a than for PP1r12b subunit.
In humans, a heart-specific small subunit hsM21 increases the sensitivity to Ca
during the cardiomyocyte contraction. Two isoforms of hsM21 encoded by the PPP1R12B gene — hsM21a and hsM21b — preferentially bind to PP1r12a and PP1r12b subunit, respectively [652]. Subunit hsM21 heightens the contraction of renal artery smooth muscle cells and rat cardiomyocytes at a given Ca
concentration.


Activity of MLCP is regulated by phosphorylation of PP1r12a and PP1r12b subunits. In the heart, hsM21 subunit supports PP1r12a phosphorylation (Thr696) by RoCK kinase. The latter indeed phosphorylates (inhibits) human PP1r12a at both Thr696 and Thr853 residues (Table 8.11).11 In addition, other kinases, such as death-associated protein kinase DAPK312 and P21-activated kinase, can phosphorylate PP1r12a (Thr696), hence causing Ca
sensitization.13

Table 8.11
Inhibition of myosin light-chain phosphatase. This trimeric holoenzyme can undergo inhibitory phosphorylation or bind to inhibitors. Inhibition of MLCP augments MLC phosphorylation state and thus contractile force of smooth muscle cells independently of Ca
ions, thereby enhancing Ca
sensitivity.


Mechanism | Agents |
---|---|
Phosphorylation | DAPK3, MRCK, cRaf, RoCK |
of PP1r12 | |
Phosphorylated | PP1r14a P, PP1r14b P, |
inhibitors | PP1r14c P |
(involved kinases) | (DAPK3, ILK, PAK, PKC, RoCK) |
8.3.2 Protein Phosphatase-2
In the cell, PP2 (or PP2a)14 exists as either a heterodimer or heterotrimer. Heterotrimeric protein phosphatase-2 (PP2) is a ubiquitous, strongly regulated phosphatase that fulfills numerous functions. Two isoforms of PP2 have been identified (with > 97% identity).
Protein phosphatase-2 is composed of: (1) a catalytic (C subunit; PP2c); (2) scaffold (structural A subunit); and (3) regulatory (B subunit) subunits (Tables 8.12 and 8.13).15
Table 8.12
Subunits of protein phosphatase-2 (Part 1; PPP: phosphoprotein phosphatase; RPc: replication protein-C; TAP42: 42-kDa 2A (two) phosphatase-associated protein; TIPRL: TIP41 [TAP42-interacting protein], TOR signaling pathway regulator-like protein). Potentially, more than 70 PP2 heterotrimers can be generated by combining various types of subunits. In mammals, both A and C subunits are encoded by 2 genes.
Subunit | Gene | Other aliases |
---|---|---|
Catalytic C subunits | ||
PP2cα | PPP2CA | RPc |
PP2cβ | PPP2CB | |
Regulatory A subunits | ||
PP2r1α | PPP2R1A | PR65α |
PP2r1β | PPP2R1B | PR65β |
Miscellaneous | ||
PP2r4 | PPP2R4 | PR53 |
IGBP1 | Igbp1 | α4, CD79aBP1, IBP1 |
TAP42 (ortholog) | ||
TIPRL | Tiprl | TIP41 |
Table 8.13
Subunits of protein phosphatase-2 (Part 2; PPP: phosphoprotein phosphatase). Four groups of B subunits are encoded by multiple genes in each group: the B group (B55 or PR55), the B(1) (or B′) group (B56 or PR61), the B(2) (or B′′) group (PR48, PR59, and PR72/PR130), and B(3) (or B′′′) group (PR93, or SG2NA, and PR110, or striatin). Striatin, SG2NA, and zinedin, the 3 mammalian members of the striatin family, are calmodulin-binding proteins that operate as signaling and scaffold proteins mainly expressed in the central nervous system, where they localize to the soma and dendrites, but not in the same neurons.
Subunit | Gene | Other aliases |
---|---|---|
Regulatory B subunits | ||
B group | ||
PP2r2α | PPP2R2A | B55α, PR55α |
PP2r2β | PPP2R2B | B55β, PR55β |
PP2r2γ | PPP2R2C | B55γ, PR55γ |
PP2r2δ | PPP2R2D | B55δ, PR55δ |
B(1) group | ||
PP2r5α | PPP2R5A | B56α, PR61α |
PP2r5β | PPP2R5B | B56β, PR61β |
PP2r5γ | PPP2R5G | B56γ, PR61γ |
PP2r5δ | PPP2R5D | B56δ, PR61δ |
PP2r5ε | PPP2R5E | B56ε, PR61ε |
B(2) group | ||
PP2r3α | PPP2R3A | B72, B130, PR72, PR130, |
PR59 (ortholog) | ||
PP2r3β | PPP2R3B | PP2R3L, PR48 |
PP2r3γ | PPP2R3G | G5PR |
B(3) group – striatin family | ||
Striatin | STRN | PR110 |
SG2NA | STRN3 | PR93 |
Zinedin | STRN4 |
The catalytic C subunit can also bind to immunoglobulin-binding protein-1,16 the mammalian ortholog of yeast Two (2A) phosphatase-associated protein of 42 kDa (TAP42), and TAP42-interacting protein (TIP41) to control TOR signaling.
Phosphatase PP2 is regulated by one regulatory subunit among the set of regulatory subunits that control PP2 substrate specificity, cellular location, and enzymatic activity. Phosphatase PP2 thus forms a large collection of oligomeric enzymes. In fact, A subunit links PP2c with many B-subunits to form more than 60 different heterotrimeric PP2 holoenzymes that can dephosphorylate many phosphoproteins [653].
Subunits PP2r2α, PP2r5α, PP2r5β, PP2r5ε are cytosolic; other PP2r2 subunits, PP2r5δ, and PP2r5γ abound in the nucleus; PP2r3β resides exclusively in the nucleus [641].
Post-translational modification of PP2 subunits (e.g., cysteine oxidation, tyrosine phosphorylation, and leucine methylation of PP2c) regulates PP2 complex formation and its activity. Stimulation by epidermal growth factor, insulin, interleukin-1, or tumor-necrosis factor-α promotes transient Tyr phosphorylation (inactivation) of PP2c. Phosphorylation targets not only PP2c, but also regulatory B subunits, notably those of the B(1) subset, in particular by protein kinase-A. Double-stranded RNA-dependent protein kinase PKR can also intervene [653]. Low PP2 activity correlates with Tyr phosphorylation of PP2c. Subunit PP2c is phosphorylated not only by activated growth factor receptors (e.g., epidermal growth factor and insulin receptor), but also Src kinases [654]. Demethylation of PP2 is carried out by PP2-specific methylesterase PME1 that inactivates PP2 by evicting manganese ions necessary for PP2 activity [655].
Midline-1 (Mid1)17 is a ubiquitin ligase member of the tripartite motif-containing protein TRIM17 family that targets the microtubule-associated pool of PP2c for degradation (Table 8.14). When Mid1 concentration decays, PP2 level rises and target of rapamycin–TORC1 signaling lowers [657].18
Table 8.14
Substrates, regulators, and partners of PP2 phosphatase.
Kinases (inactivation) | AMPK, CK2, CamK1/2/4, CDK1/2, ERK, JNK1, IKK, |
---|---|
KSR1, MAP2K1/4, MAPKAPK2, P38MAPK, PAK1, | |
PKA, PKB, PKC, PKG1, PLK, | |
S6K, RSK1/3, Src | |
Kinases (activation) | CamK4, CK1/2a, GSK3, IKK, JaK2, KSR1, PAK1, |
PKCε, cRaf, S6K, STK4, Wee1 | |
Phosphatases | PTPRa |
Peptidases | Caspase-3 |
Helicases | UPF1 |
Receptors, ion channels | NMDAR |
Other substrates | ANP32a, BCL2, CcnG1, CDC6, GADD45a, MLL, |
RBL1, RelA (NFκB), SET, Tau, Tlx1, | |
paxillin, vimentin, | |
importin-β, axin | |
PP2 regulators | Mid1 |
Heterotrimer PP2 favors cell survival [658]. Protein phosphatase-2 can both positively and negatively influence the Ras–Raf–MAP2K–ERK signaling pathway (Fig. 8.1). Moreover, PP2 regulates G2–M transition of the cell cycle [653]. Phosphatase PP2 is required to keep the CDK1–CcnB complex19 in its inactive precursor form. Phosphatase PP2 inhibits complete CDC25 phosphorylation (activation). It is also positively implicated in the exit from mitosis.
Phosphatase PP2 operates in the regulation of various signaling cascades, often in association with other phosphatases and kinases. It modulates the activity of several kinases, such as mitogen-activated protein kinases ERKs, calmodulin-dependent kinases, protein kinases-A, -B, and -C, S6K kinase, inhibitor of nuclear factor-κB IκB kinase, and cyclin-dependent kinases [653]. Agent PP2cα stabilizes the β-catenin–E-cadherin complex that mediates interactions with the actin cytoskeleton. Phosphatase PP2 participates in the axin–APC–GSK3β complex that is involved in Wnt signaling (Vol. 3 – Chap. 10. Morphogen Receptors).
In addition to its substrates, PP2 interacts with other proteins that influence PP2 subcellular location and/or activity. PP2-interacting proteins are involved in translation initiation (with the TOR pathway) and termination, as well as stress response down to cell apoptosis.
In the sarcoplasmic reticulum of cardiomyocytes, PP2 forms a complex with ryanodine receptor, FK506-binding protein FKBP12.6, protein kinase-A, PP1, and A-kinase anchoring protein to regulate ryanodine receptor activity.
Overexpression of PP2 precludes or strongly attenuates thrombin-induced F actin-made stress fiber formation and endothelial barrier protection [659]. Moreover, PP2 regulates the cytoskeleton structure in endothelial cells. Protein phosphatase-2 is associated with tight junction proteins zona occludens-1 and occludin and modulates the phosphorylation state of these junction proteins. Dephosphorylation of tight junction proteins induced by PP2 leads to their redistribution and epi- and endothelial barrier dysfunction.
In pancreatic β cells, glucose with other nutrients stimulates insulin release and triggers Ca
influx to activate calmodulin-dependent protein kinases as well as, via bRaf, ERK1 and ERK2 that participate in nutrient sensing [661].20 Calcium import also activates Ca
-dependent phosphatase PP3, which allows maximal ERK activation by glucose. In addition, PP3 controls insulin production by ERK-dependent and -independent mechanisms. Calcineurin binds to bRaf and target, in particular, Thr401 of bRaf that is used for negative feedback phosphorylation by ERK kinases, thereby counteracting negative feedback regulation of bRaf and promoting not only bRaf activation, but also bRaf–cRaf heterodimerization [661].21


8.3.3 Protein Phosphatase-3
Protein phosphatase-3 (PP3 or PP2b), or calcineurin, is a dimer composed of a catalytic (calcineurin-A) and a regulatory (calcineurin-B) subunit (Table 8.15). Calcineurin-A and -B subunits are encoded by 3 (CNAα, CNAβ, and CNAγ) and 2 (CNB1 and CNB2) genes, respectively. CNAα, CNAβ, and CNB1 are ubiquitous (particularly observed in bone marrow-derived blood cell lineages), but CNAγ and CNB2 are restricted to the germ line. Three PP3 isoforms exist (with > 80% identity).
Table 8.15
Subunits of protein phosphatase-3 (CNA, CalnA: calcineurin-A; CNB1, CalnB: calcineurin-B; CBLP: calcineurin-B-like protein; PPP: phosphoprotein phosphatase).
Subunit | Gene | Other alias |
---|---|---|
Catalytic A subunits | ||
PP3cα | PPP3CA | CNAα, CalnA1 |
PP3cβ | PPP3CB | CNAβ, CalnA2 |
PP3cγ | PPP3CC | CNAγ, CalnA3 |
Regulatory B subunits | ||
PP3r1 | PPP3R1 | CNB1, CalnB1 |
PP3r2 | PPP3R2 | CNB2, CalnB2, CBLP, PP3RL |
Phosphatase PP3 is activated by the Ca
–calmodulin complex. Calcium–calmodulin complex activates PP3 by binding to its catalytic subunit and displacing an inhibitory regulatory subunit. Activated PP3 dephosphorylates nuclear factor of activated T cells (NFAT). This transcription factor can then move into the nucleus to launch gene expression.

The PP3–NFAT axis regulates various developmental processes (development of heart valves, nervous and vascular systems, and bone) in addition to control of T-lymphocyte activity and fiber-type switching in skeletal muscle. In particular, PP3 activates the transcription of interleukin-2 that stimulates growth and differentiation of T lymphocytes when the intracellular concentration of calcium rises.
Furthermore, calcium–calmodulin-activated PP3 participates in the regulation of cardiomyocyte growth (Vol. 5 – Chap. 5. Cardiomyocytes). Enzyme PP3 is controlled by regulator of calcineurin (RCan).22 Inhibitor RCan1 impedes NFAT activation by binding to PP3 active site. Adaptor MAP3K7IP223 interacts with RCan1 to recruit MAP3K7, MAP3K7IP1, and PP3, thus forming a signaling complex [660]. Kinase MAP3K7 phosphorylates RCan1, thereby converting RCan1 from an inhibitor to a facilitator of PP3–NFAT signaling and enhancing NFAT1 nuclear translocation to promote hypertrophy of cultured cardiomyocytes. In addition, activated PP3 dephosphorylates (inactivates) MAP3K7 and MAP3K7IP1. The MAP3K7–MAP3K7IP1–MAP3K7IP2–RCan1–PP3 complex thus acts as a stimulator and feedback node, as MAP3K7–MAP3K7IP1–MAP3K7IP2-induced activation of PP3 thereafter dephosphorylates MAP3K7 and impedes MAP3K7 signaling.
8.3.4 Protein Phosphatase-4
Protein phosphatase-424 resides in the cytoplasm and nucleus, although it is mostly located at centrosomes [662]. It is involved in microtubule organization at centrosomes during cell division and meiosis.
Protein phosphatase-4 contains regulatory subunits (PP4r) that interact with the catalytic subunit (PP4c) [656]. The regulatory subunit target the centrosomal microtubule organizing centers. Three PP4 regulatory subunits have been identified: immunoglobulin-binding protein IgBP1,25 PP4r1, and PP4r2. The PP4c–PP4r1 holoenzyme may dephosphorylate HDAC3.
In response to DNA double-strand breaks, members of the phosphatidylinositol 3-kinase-like family, such as ataxia telangiectasia mutated (ATMK) and AT- and Rad3-related (ATRK) kinases, phosphorylate numerous proteins. Subsequent deactivation of these proteins can result from dephosphorylation. Phosphatase PP2 or PP4 can dephosphorylate the DNA-repair protein H2AX. Phosphatase PP4 dephosphorylates replication protein-A2 (RPa2) [663].26 Upon DNA repair and dephosphorylation of RPa2, DNA synthesis can resume and the cell cycle starts again.
Phosphatase PP4 has a pro-apoptotic role in T lymphocytes. It associates with T-complex protein TCP1 and chaperonin-containing TCP1 subunits CCT2 (CCTβ), CCT3 (CCTγ), CCT4 (CCTδ), CCT5 (CCTε), CCT6A (CCTζ), CCT7 (CCTη), and CCT8 (CCTβ), as well as TRAF6, and TLR4 [251].
In addition, PP4 interacts with components of NFκB (Rel, P50, and RelA), dephosphorylates RelA, stimulates Rel binding to DNA, and activates NFκB-mediated transcription [277] (Sect. 10.9.1).
Phosphatase PP4 also relays TNFα signal to the JNK pathway. It downregulates insulin receptor substrate-4. Lastly, PP4 interacts with, stabilizes, and activates MAP4K1 (Sect. 5.1.3). Stimulation of TCR enhances PP4–MAP4K1 interaction.
Regulatory subunits-3a and -3b of protein phosphatase-4 (PP4r3a and PP4r3b)27 are involved in the regulation of hepatic glucose metabolism. They are upregulated during fasting that promotes hepatic gluconeogenesis to maintain glucose homeostasis [664]. Their synthesis depends on peroxisome proliferator-activated receptor-γ coactivator PGC1α. The catalytic subunit of PP4 (PP4c) dephosphorylates cAMP-response element binding protein (CREB)-regulated transcriptional coactivator CRTC2 that enables transcriptional activation of gluconeogenic genes in the liver.
8.3.5 Protein Phosphatase-5
Protein phosphatase-528 localizes to the cytoplasm, centrosomes, and microtubules, or the nucleus. Phosphatase PP5, provided with auto-inhibition from its targeting and regulatory domain, acts in microtubule organization and controls the cell cycle. It regulates steroid receptor signaling [665]. It can be activated in vitro by arachidonic acid.
Its unique feature corresponds to an N-terminal extension that harbors 3 tandemly arranged tetratricopeptide repeat (TPR) motifs [666]. It is predominantly found in brain and, at a lower level, in testis, but is nearly undetectable in spleen, lung, skeletal muscle, kidney, and liver.
Protein phosphatase-5 binds to and inhibits the activated form of MAP3K5 in response to oxidative stress. It can thus prevent oxidative stress-induced, MAP3K5-dependent apoptosis. In fact, PP5 dephosphorylates the activating or inactivating phosphorylation sites of MAP3K5 enzyme.
8.3.6 Protein Phosphatase-6
Protein phosphatase-629 resides in both the nucleus and cytoplasm. It operates in cell cycle regulation. Three forms of PP6 mRNA can be detected with high levels in the heart, skeletal muscle, and testis [667].
Protein phosphatase-6 interacts with IGBP1 and TIP41. It has several binding partners, such as Sit4 (PP6)-associated proteins (SAP).
8.3.7 Protein Phosphatase-7
Photoreceptor Ser/Thr protein phosphatase-7 is produced from human retina. It has multiple Ca
-binding sites. Like PP1 to PP6, it possesses a phosphatase catalytic core domain, but has unique N- and C-termini [668]. Human PP7 is directly activated by Ca
and its activity depends on Mg
.



8.3.8 Magnesium-Dependent Protein Phosphatase-1
In humans, Mg
– and/or Mn
-dependent protein Ser/Thr phosphatases are encoded by 11 genes (Table 8.16). In addition, 2 splice variants of PPM1a and 6 splice variants of PPM1b have been identified. The PPM1 family include PPM1a to PPM1m, i.e., PP2cα to PP2cη and mitochondrial PP2cκ, as well as PP2c domain-containing protein phosphatase-1F (PPM1f).


Table 8.16
Family of Mg
-dependent protein phosphatase-1 (Source: [76]). At least 11 Ppm1 genes have been detected in humans (CamKP: Ca
–calmodulin-dependent protein kinase phosphatase; CamKPn: Ca
–calmodulin-dependent protein kinase phosphatase, nuclear subtype; Fem2: feminization of XX and XO animals protein-2; FIn: fibroblast growth factor-inducible protein phosphatase; HFeM: hereditary hemochromatosis modifier; NerPP2c: neuronal protein phosphatase-2C-related subtype, or neurite extension-related protein phosphatase-2C; POPX: partner of PAK-interacting exchange factor (PIX, or RhoGEF6/7); PP2cm: Mg
– and Mn
-dependent, PP2c-like mitochondrial protein phosphatase; PPM1L: PPM1-like subtype; WiP1: wild-type P53-induced phosphatase-1, or wound-induced protein-1).





Type | Gene | Aliases |
---|---|---|
PPM1a | Ppm1A | PP2cα |
PPM1b | Ppm1B | PP2cβ |
PPM1c, PPM1g | Ppm1G | PP2cγ, FIn13 |
PPM1d | Ppm1D | PP2cδ, WiP1 |
PPM1e | Ppm1E | CamKPn, POPX1 |
PPM1f | Ppm1F | CamKP, Fem2, HFeM2, POPX2 |
PPM1h | Ppm1H | NerPP2c |
PPM1j | Ppm1J | PP2cζ |
PPM1k | Ppm1K | PP2cκ, PP2cm |
PPM1l | Ppm1L | PP2cε |
PPM1m | Ppm1M | PP2cη, PP2cη1 |
Among PPM1 family members, PPM1h, PPM1j, and PPM1m constitute a subfamily. In humans, the PPM1 family has been enlarged to: (1) protein phosphatase PPTC7 homolog;30 (2) integrin-linked kinase-associated Ser/Thr phosphatase-2C (ILKAP, also PP2cδ); (3) PH domain and leucine-rich repeat protein phosphatases PHLPP1 and PHLPP2; and (4) pyruvate dehydrogenase phosphatases PDP1 and PDP2, hence to 17 members.
Although the PPM and PPP families do not share any sequence homology, PPM1α possesses an active site that is similar to that of the PPP family members.
In humans, several PPM1 phosphatases localize almost exclusively to the nucleus (PPM1a, PPM1d, PPM1e, and PPM1l) [641]. In addition, PPM1b, PPM1g, PPM1k, and PPM1m resides mainly in the nucleus. Isozyme PPM1g is a component of the spliceosome.
The catalytic subunits of PPM1a, PPM1b, and PPM1g are stimulated by Mg
ions. On the other hand, PPM1d is inhibited by Mg
ions.


Members of the PPM1 family are involved in many signaling axes that control cell differentiation, proliferation, growth, survival, and metabolism (Table 8.17).
Table 8.17
Members of the PPM1 family and their effects ( ⊕ →: stimulation; ⊖ →: inhibition).
Type | Substrates and partners |
---|---|
PPM1a | ⊖ → PI3K, MAP3K3/7, JNK, P38MAPK, IKKβ |
⊖ → CDK9, ⊕ →P53 | |
GluR3 | |
PPM1b | ⊖ → PI3K, MAP3K7, JNK, P38MAPK, IKKβ |
⊖ → CDK2/6/9 | |
PPM1c, PPM1g | ⊖ → Histone-2 |
PPM1d | ⊖ → ATMK, ATRK, ChK1/2, P53 |
PPM1e | ⊖ → AMPK, CamK1/4 |
RhoGEF6/7 | |
PPM1f | RhoGEF6/7 |
PPM1h | ⊖ → CKI1b, exportin-2 |
PPM1j | UbC9 |
PPM1k | membrane permeability transition pore |
PPM1l | ⊖ → MAP3K7 |
PPM1m | ⊖ → MAP3K7 |
PDP1/2 | ⊕ → pyruvate dehydrogenase |
PHLPP1 | ⊖ → PKB2/3, kRas |
PHLPP2 | ⊖ → PKB1/3 |
8.3.8.1 PPM1a
Magnesium-dependent protein phosphatase-1A31 is encoded by the PPM1A gene. Three alternatively spliced transcript variants encode 2 isoforms.
Phosphatase PPM1a impedes response to cellular stresses. It dephosphorylates (inactivates) MAP2K and MAPK enzymes, such as P38MAPK and JNK enzymes.32
Phosphatase PPM1a can also dephosphorylate cyclin-dependent kinases, in particular CDK9, the catalytic subunit of a general RNA polymerase-2 elongation factor [669].33
Upon stimulation by neurotransmitters, hormones, growth factors, cytokines, and antigens, various plasmalemmal receptors activate phosphatidylinositol 3-kinase that generates the lipid second messenger phosphoinositide 3-phosphate. In particular, the PI3K–PKB pathway supports cell growth, proliferation, and survival. Once activated, class-1A PI3K catalytic subunit (PI3Kc1 or P110PI3K) phosphorylates P85PI3K regulatory subunit, thereby impeding its lipid kinase activity (negative feedback). Like PP1 and PP2, PPM1a regulates P85 phosphorylation state. In the differentiation of fibroblasts into adipocytes, PPM1a expression rises, whereas those of PP1, PP2, and PP3 decay [670].
Overexpression of PPM1α activates P53 transcription factor to provoke G2– M arrest and apoptosis. Phosphatase PPM1α dephosphorylates metabotropic glutamate GluR3 receptor [251]. It can also interact with inhibitor latexin (Lxn), or endogenous carboxypeptidase inhibitor (ECI), that can complex with carboxypeptidase-A4.
8.3.8.2 PPM1b
Magnesium-dependent Ser/Thr protein phosphatase-1B (PPM1b)34 interacts with numerous proteins [251]: inhibitor of nuclear factor-κB kinases IKKα (or IKK1), IKKβ (or IKK2), and IKKγ, catalytic subunit-α of Ser/Thr protein phosphatase-2, cyclin-dependent kinases CDK2 and CDK6, MAP3K7,35 coregulators annexin-A1 and -A2, actin-binding proteins villin-1 and gelsolin, intermediate filament component keratin-18, S100 calcium-binding protein-A8 (S100a8, or calgranulin-A), inducible polyadenylate-binding protein PABPc4 that binds to the poly(A) tail at the 3′ ends of mRNAs,36 ATP-binding platform for protein assembly valosin-containing protein (VCP), and Ras guanine nucleotide-releasing protein RasGRP1.
Phosphatase PPM1b is widely distributed with its highest expression in cardiac and skeletal myocytes. Unlike PPM1b1 variant, PPM1b2 is not widespread, but specifically lodges in the brain and heart.
Isozyme PPM1b dephosphorylates (inactivates) IKKβ stimulator. Like PPM1a, PPM1b acts as an IKKβ phosphatase that terminates NFκB activation generated by tumor-necrosis factor-α [671]. Furthermore, PPM1b and PPM1l dephosphorylate (inactivate) MAP3K7, thereby suppressing IL1-induced activation of JNK kinase.
8.3.8.3 PPM1c (PPM1g)
Subtype PPM1c, also called PPM1g and PPM1γ, mostly resides in the heart, skeletal muscle, and testis. It helps to form the spliceosome; this splicing factor dephosphorylates specific substrates required for the spliceosome formation.
8.3.8.4 PPM1d
Overexpression of PPM1d subtype can stop apoptosis. It is overexpressed in many cancers. Phosphatase PPM1d can deactivate both P53 and checkpoint kinase ChK1 that activates P53 in response to DNA damage. Although PPM1d is stimulated by P53, it impedes the activity of P38MAPK, thereby preventing P53 phosphorylation (negative feedback). In addition, PPM1d represses ATMK signaling by eliminating ATMK phosphorylation (Ser1981) as well as removes the phosphorylation of ChK1 (Ser345) and ChK2 (Thr68) checkpoint kinases by ATMK and ATRK enzymes [670].
8.3.8.5 PPM1e
Among its substrates, Mg
– and Mn
-dependent protein Ser/Thr phosphatase PPM1e targets AMP-activated protein kinase (AMPK) [672]. This phosphatase is also an interactor of guanine nucleotide-exchange factors RhoGEF6 and RhoGEF7 that regulate P21-, CDC42-, and Rac1-activated kinase PAK1, thereby repressing PAK1 effects. Glyceraldehyde 3-phosphate dehydrogenase (GAPDH) and fructose bisphosphate aldolase as major binding partners of PPM1e phosphatase, the latter counteracting GAPDH phosphorylation by CamK1 or CamK4 [673]. Calmodulin-dependent PPM1e phosphatase indeed dephosphorylates both CamK1 or CamK4 phosphorylated by Cam2K, but not GAPDH enzyme.


8.3.8.6 PPM1f
Calmodulin-, Mg
-, and Mn
-dependent protein Ser/Thr phosphatase PPM1f, like PPM1e, dephosphorylates Ca
–calmodulin-dependent protein kinases, in particular CamK2γ.38 Whereas PPM1e localizes to the nucleus, PPM1f resides in the cytosol. Like PPM1e, PPM1f interacts with guanine nucleotide-exchange factors RhoGEF6 and RhoGEF7, thus preventing the action of P21-, CDC42-, and Rac1-activated kinase PAK1. The latter is a substrate of PPM1f phosphatase.



8.3.8.7 PPM1h
The Ser/Thr phosphatase PPM1h dephosphorylates cyclin-dependent kinase inhibitor CKI1b [674]. It controls cell cycle and proliferation of cancer cells, as it dephosphorylates exportin-2.39 It has a greater affinity for Mn
than Mg
ions. In particular, PPM1h prefers Mn
when nitrophenyl phosphate or phosphopeptide is the substrate and Mg
when casein is the substrate [675].




8.3.8.8 PPM1j
Subtype PPM1j is specifically synthesized in testicular germ cells. It can associate with ubiquitin conjugase UbC9. This association is enhanced by coexpression of small ubiquitin-related modifier-1 (SUMo1).
8.3.8.9 PPM1k
Mitochondria are involved in cellular metabolism and survival. They are integrative sites of cell signaling, as phosphorylation and dephosphorylation cycles occur in mitochondria. Many Ser/Thr protein kinases (PKA, PKB, PKC, and JNK) localize to mitochondria, where they contribute to the regulation of cell metabolism and apoptosis, as they target matrix and inner and outer membrane components (e.g., ATP synthase, voltage-dependent anion channel [porin], BCL2 antagonist of cell death [BAD], and BCL2-associated X protein [BAX]).
Conversely, mitochondrial proteins are dephosphorylated by resident mitochondrial protein phosphatases, such as protein Ser/Thr phosphatases PP1 and PP2, as well as mitochondrial protein Tyr phosphatase PTPM1, or dual-specificity phosphatase DuSP23 [676]. The latter participates in ATP production and insulin secretion.
In addition, PPM1k localizes exclusively to the mitochondrial matrix [676]. Loss of PPM1k does not affect mitochondrial oxygen consumption and ATP production, but renders mitochondria hypersensitive to membrane permeability transition pore opening. Hence, PPM1k contributes to cell death regulation [670].
8.3.8.10 PPM1l
Subtype PPM1l inhibits the MAP3K7- and IL1-induced activation of the MAP2K3–P38MAPK and MAP2K4–JNK pathways. Therefore, like PPM1a and PPM1b, PPM1l interacts directly or indirectly with several components of the MAPK modules to operate in stress responses and regulate cell death and survival [670].
8.3.8.11 PPM1m
Protein Ser/Thr phosphatase PPM1m, or PPM1m1, can localize mainly in the nucleus. An alternative splice variant — PPM1m2 — resides in the cytoplasm. This isoform inhibits interleukin-1 signaling mediated by nuclear factor-κB that triggers inflammation, as it inhibits MAP3K7, hence IKKβ [671].40 Both PP2 and PP3 also hamper the NFκB pathway. The former dephosphorylates P65NFκB (RelA), the latter dephosphorylates IκB.
8.3.8.12 PH Domain and Leucine-Rich Repeat Protein Phosphatases
PH domain and Leu-rich repeat protein phosphatases (PHLPP) constitute a family with 2 identified members, PHLPP141 and PHLPP2.42 They dephosphorylate (inactivate) all protein kinase-B isoforms (PKB1–PKB3; Ser473) as well as conventional and novel protein kinase-C isoforms. Both PHLPP1 and PHLPP2 selectively dephosphorylate PKB at Ser473, but not Thr308, which is targeted by PP2 phosphatase [670]. Phosphatase PHLPP1 dephosphorylates PKB2 that induces glucose transport upon insulin stimulation and PKB3 predominantly expressed in brain, whereas PHLPP2 targets PKB1 involved in growth and PKB3 enzyme.
Both PHLPP1 and PHLPP2 contain a PH domain for membrane recruitment and a leucine-rich repeat (LRR) domain to impede kRas activation. In particular, PHLPP1 is constitutively expressed in neurons and colocalizes in membrane rafts with kRAsGDP, prevents GTP binding to kRas, thereby precluding kRas–MAPK signaling in membrane rafts [677].
8.3.8.13 Pyruvate Dehydrogenase Phosphatases
The first irreversible reaction in glucose oxidation is catalyzed by the pyruvate dehydrogenase complex inside mitochondria. Pyruvate dehydrogenase (PDCE1) is a component of the large pyruvate dehydrogenase complex (PDC).43 Pyruvate dehydrogenase kinases phosphorylates (inactivates) Ser residues of pyruvate dehydrogenase component, thereby inhibiting the enzymatic complex. Conversely, pyruvate dehydrogenase phosphatases dephosphorylate (activate) pyruvate dehydrogenase component. All these enzymes localize in the mitochondrial matrix.
Magnesium-dependent, calcium-stimulated, pyruvate dehydrogenase phosphatase PDP1, which belongs to the protein phosphatase PPM1 category, is a heterodimer with a catalytic (PDP1c) and a regulatory subunit (PDP1r). On the other hand, PDP2 consists of a single catalytic subunit (PDP2c) [678]. Two catalytic subunit types abounds in the skeletal muscle and liver, respectively.
In skeletal myocytes and hepatocytes, insulin elevates the activity of the pyruvate dehydrogenase complex, as it causes activation and mitochondrial translocation of PKCδ that phosphorylates (activates) PDP1 and PDP2 phosphatases [679].
8.3.9 Cytosolic Protein Tyrosine Phosphatases
Classical protein Tyr phosphatases can be classified as: (1) plasmalemmal, receptor or receptor-like, transmembrane enzymes (rPTP or PTPR; Vol. 3 – Chap. 9. Receptor Tyrosine Phosphatases) and (2) cytoplasmic (cPTP or non-receptor PTPn) proteins. Protein Tyr phosphatases can dephosphorylate (inactivate) receptor Tyr kinases (RTK), such as EPH receptors [680].
In humans, among (at least) 107 known protein Tyr phosphatases, 11 are catalytically inactive, 2 dephosphorylate mRNAs, and 13 dephosphorylate inositol phospholipids. The remaining 81 PTPs dephosphorylate TyrP residue.
Phosphatases that are able to dephosphorylate TyrP residues are classified into various superfamilies (superfamilies of plasmalemmal and cytosolic protein Tyr phosphatases and dual-specificity phosphatases). Among them, dual-specificity phosphatases target not only Tyr, but also Ser and Thr residues. For example, MAPK dephosphorylation (inactivation) is catalyzed by cell cycle regulatory phosphatase CDC25 and PTen (phosphatase and tensin homolog deleted on chromosome 10), an antagonist of the PI3K–PKB pathway, hence its designation as a tumor-suppressor phosphatase (Table 8.18).
Table 8.18
Examples of signaling substances targeted by protein tyrosine phosphatases – strictly tyrosine or dual-specificity phosphatases – (PTPn11: protein Tyr phosphatase non-receptor type 6 [a.k.a. SHP2: Src homology protein Tyr phosphatase-2]; PTen: dual-specificity phosphatase and tensin homolog; MKP: dual-specificity mitogen-activated protein kinase phosphatase; Source: [680]).
Tyr phosphatase | Signaling molecules |
---|---|
PTPn11 | PDGF, endothelin-1 |
PTen | PDGF, EGF |
MKP (JNK phosphatase) | TNFα |
Protein Tyr phosphatases are classified according to the key amino acid involved in the catalysis: Cys-based and Asp-based PTPs. The Cys-based PTPs share a canonical P loop in the phosphatase domain that contains the cysteine required to form the thiol phosphate intermediate. In fact, protein Tyr phosphatases can be grouped into 4 infraclasses depending on the amino acid sequence of their catalytic domains (Tables 8.19 to 8.21). The Cys-based PTPs are indeed subdivided based on the characteristics of the catalytic domain.
Table 8.19
Classification of protein Tyr phosphatases (PTP; Part 1; NRPTP: non-receptor protein Tyr phosphatases [superfamily]; PTPR: protein Tyr phosphatase receptor; RPTP: receptor protein Tyr phosphatases [superfamily]).
Infraclass-1 Cys-based PTPs (PTPIC1) | |
---|---|
Hyperfamily of classical PTPs | |
RPTP | PTPRa–PTPRh, PTPRj–PTPRk, PTPRm, PTPRn–PTPRn2, |
PTPRo, PTPRq–PTPRv, PTPRz1, PTPRz2(?) | |
NRPTP | PTPn1–PTPn7, PTPn9, PTPn11–PTPn14, PTPn18, |
PTPn20a–PTPn20c, PTPn21–PTPn23 |
Table 8.20
Classification of protein Tyr phosphatases (PTP; Part 2; ADUSP: atypical dual-specificity phosphatase hyperfamily [aDuSP proteins]; CDC14: cell division cycle phosphatase-14; DuSP: dual-specificity phosphatase; MKP: mitogen-activated protein kinase phosphatase; MTM: myotubularin (myotubular myopathy-associated gene product); PRL: phosphatase of regenerating liver; PTEN: superfamily of phosphatase and tensin homolog [PTen] deleted on chromosome 10 [ten] and its related proteins; PTP4A: family of type-4A protein tyrosine phosphatases [PTP4a1–PTP4a3]; SBF: SET-binding factor; SSH: family of slingshot homolog phosphatases [Ssh]).
Infraclass-1 Cys-based PTPs (PTPIC1) | |
---|---|
Hyperfamily of dual-specificity phosphatases | |
MKP | DuSP1–DuSP2, DuSP4, DuSP10, STYXL1 |
ADUSP | DuSP3, DuSP11–DuSP12, DuSP13a–DuSP13b, DuSP14–DuSP15, |
DuSP18–DuSP19, DuSP21–DuSP23, DuSP26–DuSP28, | |
Laforin (EPM2a), PTPmt1, RNGTT, STYX | |
SSH | Ssh1–Ssh3 |
PTP4A | PTP4a1 (PRL1)–PTP4a3 (PRL3) |
CDC14 | CDC14a–CDC14b, CDKn3, PTPDC1 |
PTEN | PTen, TenC1 (Tns2), Tns1, TPTe1–TPTe2 |
MTM | MTM1, MTMR1–MTMR5 (SBF1), MTMR6–MTMR13 (SBF2), |
MTMR14 |
Table 8.21
Classification of protein Tyr phosphatases (PTP). (Part 3) Infraclasses 2 to 4 (CTDP1: carboxy (C)-terminal domain (CTD), RNA polymerase-2, polypeptide-A phosphatase subunit-1 [a.k.a. RNA polymerase-2 subunit-A C-terminal domain phosphatase and TF2F-associating CTD phosphatase; CTDSP: CTD-containing small phosphatase [CTDSP1 is a.k.a. small CTD-containing phosphatase SCP1 and nuclear LIM interactor (NLI)-interacting factor NIF3; CTDSP2 as NIF2 and SCP2]; CTDSPL: CTD-containing small phosphatase-like protein [CTDSPL1 is a.k.a. NIFL (NLI-interacting factor-like protein) and SCP3]).
Set | Members |
---|---|
Infraclass-2 of Cys-based PTPs (PTPIC2) | |
Acid phosphatase-1 | AcP1 |
Infraclass-3 of Cys-based PTPs (PTPIC3) | |
Cell division cycle-25 homologs | CDC25a–CDC25c |
Infraclass of Asp-based PTPs (PTPIC4) | |
Eye absent homologs | EyA1–EyA4 |
Haloacid dehalogenases | HADs |
CTD phosphatase | CTDP1 (FCP1) |
CTDSP phosphatases | CTDSP1–CTDSP1 (SCP1–SCP2), |
CTDSPL1–CTDSPL2 |
Protein Tyr phosphatases of the 3 infraclasses display a highly conserved catalytic domain ( ∼ 240 residues). Phosphatases of infraclass 1 contain a catalytically inactive CDC25 homology (CH2) domain. These phosphatases possess an Asp distant from the catalytic Cys that acts in the catalysis via protonation.
Infraclass-1 cysteine-based PTPs constitute the largest PTP subfamily with at least 102 proteins. It comprises 2 hyperfamilies: (1) classical PTPs that are strictly Tyr-specific (at least 41 members) and constitute the 2 superfamilies of transmembrane and cytoplasmic PTPs and (2) dual-specific protein phosphatases with at least 61 members that form diverse families with numerous types of substrates, from 5′ cap end nucleotide structure of primary RNA transcripts such as precursor messenger RNAs, to inositol phospholipids and SerP, ThrP, and TyrP proteins. Among these dual-specificity protein Tyr phosphatases, some have non-proteic substrates, such as phosphatase and tensin homolog and myotubularins that dephosphorylate phosphoinositides. In addition, laforin dephosphorylates phosphorylated glycogen; RNA guanylatetransferase and 5′-phosphatase (RNGTT) can remove a phosphate from the 5′ end of nascent mRNA; mitochondrial PTPmt1 selectively processes mainly phosphatidylglycerol phosphate.44
Infraclass-2 cysteine-based PTP in humans corresponds to a single phosphatase, the Tyr-specific low-molecular-weight phosphatase, that has isoforms. The phosphatase domain has a structure that differs from that of Cys-based PTPs of 2 other infraclasses. The Asp involved in the catalysis, which is N-terminally located w.r.t. the catalytic Cys in infraclass-1 PTPs, lodges in the C-terminus of AcP1 (or lmwPTP).
Infraclass-3 cysteine-based PTPs are rhodanese-related Tyr/Thr-specific phosphatases represented by 3 cell cycle regulatory CDC25 phosphatases (CDC25a–CDC25c). The phosphatase domain contains a rhodanese fold. The catalytic residue Asp is absent in CDC25 enzymes. The CDC25 phosphatases lack any sequence similarity to other DuSPs, except the canonical P-loop Cys–X5–Arg (CX5R) motif in the phosphatase domain with the Cys residue common to all Cys-based PTPs. Infraclass-3 phosphatases dephosphorylate cyclin-dependent kinases.
The last infraclass includes aspartate-based PTPs, for which the aspartic acid acts as a key element in the catalysis. Activity of these phosphatases depends on the presence of divalent cations. Members of the Asp-based phosphatase infraclass correspond to: (1) Eyes absent (EyA) proteins that are Tyr-specific protein phosphatases and operate as transcriptional coactivators and (2) bacterial haloacid dehalogenases (HAD) that are either Tyr-, Ser-, or dual Ser/Tyr-specific protein phosphatases and have various substrates (proteins, phospholipids, carbohydrates, and nucleotides).45 Other members of this infraclass dephosphorylate SerP and ThrP substrates. Chronophin regulates the cytoskeleton by processing cofilin. Carboxy-terminal domain (CTD), RNA polymerase-2, polypeptide-A phosphatase subunit-1 (CTDP1)46 dephosphorylates the C-terminal domain of RNA polymerase-2, thereby regulating transcription. Carboxy-terminal domain (CTD)-containing nuclear envelope phosphatase CTDNEP1, or Dullard homolog, participates in nuclear membrane genesis, as it dephosphorylates the phosphatidic acid phosphatase lipin, a phosphatidate phosphatase and transcriptional coactivators.47
8.3.10 Protein Tyrosine Phosphatase Regulation by Reversible Oxidation
Oxidation of the catalytic cysteine of protein Tyr phosphatases causes a reversible inactivation. Different types of oxidative modification of the PTP catalytic cysteine exist.48 This mechanism ensures signaling by reactive oxygen or nitrogen species (Tables 8.22 and 8.23). Both the general cellular redox state and extracellular ligand-stimulated ROS production can prime PTP oxidation [682] (Sect. 10.6). However, protein Tyr phosphatases differ in their intrinsic susceptibility to oxidation.49 Many types of oxidants interact with PTP active sites (Table 8.24).
Table 8.22
Protein Tyr phosphatases and reactive oxygen species (Source: [682]; AA; arachidonic acid; COx: cyclooxygenase; GF: growth factor; Gpx: glutathione peroxidase; LOx: lipoxygenase; NOx: NADPH oxidase; PLA2: phospholipase A2; Prx: peroxiredoxin; RTK: receptor Tyr kinase; SHC: Src homology-2 domain-containing transforming protein). A reducing enviroment, i.e., low activity of ROS producers and high activity of ROS scavengers, lowers PTP oxidation, thereby enhancing their activation and attenuating RTK signaling. Conversely, an oxidizing enviroment elevates PTP oxidation and improves RTK activity. Phosphorylation (activation) of SHC1 causes mitochondrial production of hydrogen peroxide (H2O2). Inhibitory phosphorylation of ROS scavenger Prx1 is another mechanism for growth factor-induced PTP oxidation.
Producers | Inhibitors |
---|---|
ROS | |
NOx | Catalase |
SHC1 (mitochondrion) | Prx, Gpx |
Peroxidized lipids | |
COx, LOx | Gpx |
PTP oxidation (inactivation) pathways | |
GF–RTK–PI3K–Rac–NOx | |
GF–RTK–SHC1 | |
GF–RTK ⊖ →Prx1 | |
GF–RTK–cPLA2–AA–peroxidized lipids |
ROS producers | |
---|---|
DuOx1 | PTPn11 oxidation for enhanced and sustained TCR signaling |
NOx | PTPn12 oxidation in focal adhesions for cell migration |
NOx1 | PTPn1 oxidation in IL-4 signaling |
NOx4 | PTPn1 oxidation at the endoplamoic reticumul in EGF signaling |
SOD1 | PTP oxidation in growth factor signaling |
ROS scavengers | |
Gpx1 | Scavenges H2O2 to protect PTen from oxidation, |
desensitization of insulin signaling | |
Gpx4 | Protection of PTPs from oxidation by peroxided lipids |
in PDGF signaling | |
Prx2 | Protection of PTPs from oxidation in PDGF signaling |
Table 8.24
Examples of PTPs inhibited by oxidants, such as ROS, RNS, peroxidized lipids such as 15-hydroperoxy eicosatetraenoic acid, and toxic constituents of ambient particulate matter, such as quinones and aldehydes (Source: [682]). Reaction with H2O2 oxidizes PTP active-site thiolates initially to sulfenic acid (SOH), and then, at high concentrations, sulfinic (SO2H) and sulfonic acid (SO3H) derivatives.
Oxidant | Target PTPs |
---|---|
Hydrogen peroxide | RPTPa, PTPn1/6/11, DuSP3/6, |
CDC25, PTen | |
Superoxide | PTPRc, PTPn1 |
Lipid peroxides | PTPn2/3/6 |
Oxidized glutathione | PTPRm, PTPn1/6, DuSP6, PTen |
S-nitrosothiols | PTPn1, PTen |
Polyaromatic quinones | PTPRc, PTPn1 |
Amino acid and peptide hydroperoxides | PTPn1 |
Peroxymonophosphate | PTPn1 |
Hypothiocyanous acid | PTPn1 |
Growth factors cause oxidation of many classical PTPs, in addition to other cell surface triggers (Table 8.25). In addition to classical PTPs, dual-specificity phosphatases (DuSP) and PTen that catalyze dephosphorylation by a cysteine-based mechanism undergo oxidation (inactivation; Table 8.26).
Table 8.25
Oxidation of PTPs by activated plasmalemmal receptors (Source: [682]; EGFR: epidermal growth factor receptor; InsR: insulin receptor; PDGFR: platelet-derived growth factor receptor; VEGFR: vascular endothelial growth factor receptor).
Plasmalemmal receptors | Target PTPs |
---|---|
G-protein-coupled receptors | |
Adiponectin | PTPn1 |
Angiotensin-2 receptor-1A | PTPn11 |
Endothelin-1 receptor | PTPn11 |
S1P2 | PTPn1 |
Urotensin-2 receptor | PTPn11 |
Receptor Tyr kinases | |
EGFR | PTPn1, PTen |
InsR | PTPn1, PTPn2, PTen |
PDGFR | PTPn11, lmwPTP, PTen |
VEGFR2 | PTPRj, PTPn1 |
Cytokine receptors | |
Interleukin-4 receptor | PTPn1 |
TNFR1 | MKP1/3/5/7 |
Immune system receptors | |
BCR | PTPn6 |
TCR | PTPn6/11 |
Integrins | |
Integrins | PTPn11, lmwPTP |
Table 8.26
Regulation of protein Tyr phosphatases (PTP) by reversible oxidation of the active site cysteine (Source: [682]; DuSP: dual-specificity phosphatase; MKP: MAPK phosphatase; PRL: phosphatase of regenerating liver; PTen: phosphatase and tensin homolog).
Category | Members |
---|---|
Infraclass-1 Cys-based PTPs | |
Transmembrane PTPs | PTPRa, PTPRc, PTPRf, PTPRj, |
(receptor-like) | PTPRk, PTPRm, PTPRz |
Cytosolic PTPs | PTPn1, PTPn2, PTPn3, PTPn6, |
PTPn11, PTPn12, PTPn13, PTPn22 | |
DSPs | DuSP1 (MKP1), DuSP6 (MKP3), DuSP10 (MKP5), |
DuSP16 (MKP7), DuSP3, DuSP12 | |
PRLs | PRL1, PTP4a1 |
Infraclass-2 Cys-based PTPs | |
lmwPTP | |
APC1 | |
Infraclass-3 Cys-based PTPs | |
CDC25 | CDC25b, CDC25c |
PTEN | |
PTEN | PTen, Tns1/2, TPTe1/2 |
8.3.11 Classical Cytosolic Protein Tyrosine Phosphatases
In humans, protein Tyr phosphatases can be categorized into 20 sets according to the presence, number, and position of each detected domain [683]. For example, PTPn5, PTPn7, and PTPn20 contain a single PTPase domain at their C-terminus. Subtype PTPn1 and PTPn2 also have a single PTPase domain, but at their N-terminus.50 The B41 domain responsible for targeting of cytoskeletal proteins to the membrane–cytoskeleton interfaces is found in PTPn3, PTPn4, PTPn13, PTPn14, and PTPn21 phosphatases.
Protein Tyr phosphatases counteract the reversible protein Tyr phosphorylation on many substrates carried out by protein Tyr kinases to regulate cell differentiation and proliferation (Tables 8.27 to 8.30).
Table 8.27
Partners and substrates of cytoplasmic protein Tyr phosphatases (Part 1; Sources: Wikipedia and BioGRID; ADAM: a disintegrin and metallopeptidase ATRX: α-thalassemia/mental retardation syndrome RAD54 homolog X-linked [DNA-dependent ATPase and helicase]; eEF: eukaryotic translation elongation factor; EGFR: epidermal growth factor receptor; GP: glycoprotein; GluRδ2: ionotropic glutamate receptor-δ subunit [GRID2]; GluN2a: ionotropic glutamate receptor, N-methyl D-aspartate subunit [GRIN2A]; IGF1R: insulin-like growth factor-1 receptor; InsR: insulin receptor; LPase: lipoprotein lipase; YWHAB: Tyr 3-monooxygenase/Trp 5-monooxygenase activation protein of the 14-3-3 protein family (14-3-3β/α); ZZEF1: zinc finger, ZZ-type with EF-hand domain-1 protein).
Gene | Expression | Partners and substrates |
---|---|---|
pattern | ||
PTPN1 | Ubiquitous | EGFR, InsR, IGF1R, IRS1 |
NTRK1–3, LTK, JaK2, TyK2, GSK3β | ||
GRB2, BCAR1 (CAS), CRK, STAT5a/b | ||
Cav1, Catenin-β1 | ||
PTPN2 | Hematopoietic cell | JaK1/3, STAT1/3 |
14-3-3σ | ||
PTPN3 | Neuron | CDC48, YWHAB, ADAM15, 14-3-3σ |
PTPN4 | Neuron | GluRδ2, GRIN2A |
eEF1α and γ, ATRX, ZZEF1, LPase | ||
PTPN5 | Neuron | Fyn, ERK1/2, P38MAPK |
Table 8.28
Partners and substrates of cytoplasmic protein Tyr phosphatases (Part 2; Sources: Wikipedia and BioGRID; Arfaptin: adpribosylation factor interacting protein; BCR: B-cell receptor; EGFR: epidermal growth factor receptor; EpoR: erythropoietin receptor; FCRL3: Fc receptor-like-3; Hox: homeobox transcription factor; InsR: insulin receptor; LAIR: leukocyte-associated immunoglobulin-like receptor; LCP: lymphocyte cytosolic protein (LCP2 alias: SLP76); LILRB: leukocyte immunoglobulin-like receptor-B; M6PRBP: mannose-6-phosphate receptor-binding protein [or perilipin-3]; PAG: phosphoprotein associated with glycosphingolipid microdomains [or CBP: CSK-binding protein]; PILRα: paired immunoglobin-like type 2 receptor-α; SIRP: signal regulatory protein; SLAMF: signaling lymphocytic activation molecule family protein).
Gene | Expression | Partners and substrates |
---|---|---|
pattern | ||
PTPN6 | Hematopoietic cell | EGFR, SstR2, InsR, EpoR, SCFR, CSF2Rb, |
Ubiquitous | IL4R, BCR, CD5, CD22, CD72, | |
SIRPA, Siglec2/3/11, FcRL3, PILRα, | ||
LIFR, LAIR1, LILRB1/2/4, SLAMF6, BLnk, | ||
IL6ST, TREML1, C6orf25, CXCR4, | ||
Ros1, Abl1, Lck, Lyn, SYK, ZAP70, FAK2, | ||
JaK1/2, TyK2, PKCδ, PI3KR1, NOS1, | ||
PTPNS1, SOS, Vav1, GRB2, GAB2, LCP2, | ||
CBL, PAG1, Cav1, Catenin-δ1, | ||
PECAM1, STAT5b, HoxA10 | ||
PTPN7 | Hematopoietic cell | ERK1/2, P38MAPK |
PTPN8(22) | B and T cells, | CSK, LCK, Fyn, ZAP70 |
Myeloid cell lines | GRB2, CBL | |
PTPN9 | Hematopoietic cell | M6PRBP, Arfaptin (ArfIP1), reticulon-3 |
Table 8.29
Partners and substrates of cytoplasmic protein Tyr phosphatases (Part 3; Sources: Wikipedia, BioGRID; CEACAM: carcinoembryonic antigen-related cell adhesion molecule; EFS: embryonal Fyn-associated substrate; EpoR: erythropoietin receptor; FCRL3: Fc receptor-like-3; FRS2: fibroblast growth factor receptor substrate; GHR: growth hormone receptor; GP: glycoprotein; IGF1R: insulin-like growth factor-1 receptor; InsR: insulin receptor; LAIR: leukocyte-associated immunoglobulin-like receptor; LILRB: leukocyte immunoglobulin-like receptor-B; PSTPIP: Pro–Ser–Thr phosphatase-interacting protein; SLAMF: signaling lymphocytic activation molecule family protein; SorbS2: sorbin and SH3 domain-containing protein-2 [a.k.a. Arg–Abl-interacting protein ArgBP2]; TGFβ1I1: transforming growth factor-β1-induced transcript-1 [a.k.a. 55-kDa androgen receptor coactivator ARA55 and hydrogen peroxide-inducible clone-5 protein HIC5]).
Gene | Expression | Partners and substrates |
---|---|---|
pattern | ||
PTPN11 | Hematopoietic cell | PDGFRβ, HER1/2/4, GHR, PrlR, IGF1R, InsR, |
Ubiquitous | EPHa2, SCFR, CSF2Rβ, CSF3R, EpoR, IL4R | |
GP130, CD33, PIlRα, LILRB4, MPZL1 | ||
SLAMF1/6, NK-cell CD244, FcGR2B, FcRL3 | ||
IRS1/2, FRS2, IL6ST, Siglec11, Cav1, C6orf25 | ||
Ros1, JaK1/2, FAK1/2, Fyn, SYK, TyK2 | ||
ERK, PI3KR1, PTPn6, PLCγ2 | ||
GAB1/2, GRB2, SHC, LAIR1, SIT1, CRKL, CBL | ||
SOS1, STAT3, STAT5a/b, SOCS3 | ||
SynCRIP, catenin-β1 | ||
PECAM1, CEACAM1, VE-cadherin, selectin-E | ||
PTPN12 | Ubiquitous | Abl, CSK, FAK1/2, LCK, JaK2 |
BCAR1 (CAS), CASL, GRB2, SHC, Sin, | ||
PSTPIP1, TGFβ1I1 | ||
Paxillin, leupaxin, gelsolin, WASP | ||
EFS, p190RhoGAP, ArgBP2 |
Table 8.30
Partners and substrates of cytoplasmic protein Tyr phosphatases (Part 4; Sources: Wikipedia and BioGRID; APC: adenomatous polyposis coli Ub ligase; KIF: kinesin-like protein; PSTPIP: Pro–Ser–Thr phosphatase-interacting protein).
Gene | Expression | Partners and substrates |
---|---|---|
pattern | ||
PTPN13 | Ubiquitous | TNFRSF6a, TNFSF6, PAK2, IκBα |
Catenin-β1, ephrin-B1, | ||
RhoGAP29, APC | ||
PTPN14 | Ubiquitous | Catenin-α1, -β1, and -γ |
Zona occludens-1 | ||
PTPN18 | Hematopoietic | CSK, TEC, Fyn |
progenitors | PSTPIP | |
Brain, colon | ||
PTPN21 | Hematopoietic cell | BMX, Src |
KIF1C | ||
PTPN22: see PTPN8 | ||
PTPN23 | Endothelial cell |
8.3.11.1 Non-Receptor Protein Tyrosine Phosphatase-1
Non-receptor protein Tyr phosphatase-1 (PTPn1 or PTPn1b) is an abundant enzyme with a broad tissue distribution. It is associated with the outer leaflet of the endoplasmic reticulum membrane [684]. Growth factor stimulation provokes production of a PTPn1 splice variant with a modified C-terminus. Phosphatase PTPn1 is encoded by the PTPN1 gene (N: non-receptor).
Phosphatase PTPn1 particularly inhibits signaling downstream of insulin and leptin receptors [684]. Phosphatase PTPn1 indeed dephosphorylates (inactivates) insulin receptor Tyr kinase (InsR) and possibly insulin receptor substrate proteins as well as both mediators JaK2 and STAT3 of the leptin pathway [685] (Tables 8.27 to 8.29). It also inhibits prolactin signaling by preventing nuclear translocation of dephosphorylated effectors STAT5a and STAT5b [686]. On the other hand, PTPn1 dephosphorylates (activates) Src kinase, particularly in platelets.
Phosphatase PTPn1 also regulates the insulin response element-mediated unfolded protein response in the endoplasmic reticulum. It reduces caveolin-1 phosphorylation (Tyr14), thus its docking capacity. It forms a complex with α-actinin and dephosphorylates (Tyr397; inactivates) FAK [684]. Among hyper(Tyr) phosphorylated proteins that link to PTPn1, there are regulators of cell motility and adhesion, such as cortactin, lipoma-preferred partner, tight junction protein zona occludens ZO1, and catenin-δ1, as well as docking protein Dok1 and p120RasGAP to lower cell motility and heighten proliferation [687].
Phosphatase PTPn1 also interacts with adaptor GRB2 and cytoskeletal component adaptor BCAR1 (CAS or p130CAS) as well as SUMo ligase Protein inhibitor of activated STAT PIAS1. It can associate with and dephosphorylate many receptor (PDGFRa and PDGFRb, InsR, IGFR1, and EGFR) and non-receptor (JaK2, TyK2, and Src) protein Tyr kinases [684]. It also binds to N- and E-cadherins, then dephosphorylates β-catenin to increase the strength of cadhesin–catenin linkage, thus intercellular adhesion.
Small RhoA GTPase inhibits PTPn1 enzyme. Transcription factor Yb1 contributes to the regulation of PTPn1 synthesis. Activity of PTPn1 is regulated by proteolysis, oxidation, phosphorylation, glutathionylation, and sumoylation [684]. Phosphatase PTPn1 is phosphorylated by PKB (Ser50) to impede its phosphatase activity, thus creating a positive feedback loop for insulin signaling [688].
8.3.11.2 Non-Receptor Protein Tyrosine Phosphatase-2
Non-receptor protein Tyr phosphatase-2 (PTPn2) that is encoded by the PTPN2 gene is also called T-cell protein Tyr phosphatase (TC-PTP). Three alternatively spliced variants of mRNA from the PTPN2 gene encode isoforms that differ at their non-catalytic C-termini: 48-kDa, long (PTPn2 L ; also called TC48 and TCPTPβ) and 45-kDa, short (PTPn2 S ; also termed TC45 and TCPTPα) form of PTPn2. Different C-termini determine substrate specificity and cellular location of the isoforms. PTPn2 L localizes to the endoplasmic reticulum, whereas under basal conditions PTPn2 S resides in the nucleus [689]. Phosphatase PTPn2 is mainly produced by hematopoietic cells.
Among PTPn2 substrates, there are epidermal growth factor receptor and adaptor SHC [689, 690]. Both PTPn2L and PTPn2S isoforms recognize phosphorylated EGFR. Long subtype PTPn2L can prevent inappropriate signaling by nascent receptor, whereas PTPn2S exits the nucleus in response to EGF and is recruited to plasmalemmal, EGFR-containing complexes. Splice variant PTPn2S hampers EGFR signaling after EGFR or integrin stimulation toward the PI3K–PKB axis [690]. Splice variant PTPn2S also precludes EGF-induced association of SHC with GRB2 [689].
In addition to STAT1, PTPn2 S binds to signal transducers and activators of transcription STAT3 and STAT5a/b to repress IL6 and prolactin pathways, respectively [691]. Both endoplasmic reticulum and nuclear isoforms dephosphorylate (inactivate) insulin receptor [692]. Phosphatase PTPn2 targets JaK1 and JaK3, whereas PTPn1 dephosphorylates (inactivates) JaK2 kinase.
8.3.11.3 Non-Receptor Protein Tyrosine Phosphatase-3
Non-receptor protein Tyr phosphatase-3 (PTPn3)51 is encoded by the PTPN3 gene. It is characterized by an N-terminus that is homologous to cytoskeletal-associated proteins of the band 4.1 superfamily (band 4.1, ezrin, radixin, moesin, merlin, and talin) [693]. Band 4.1 Domain and phosphatase segment in the C-terminus are separated by a central motif with one PDZ binding domain. Phosphatase PTPn3 is involved in thalamocortical connections.
Phosphatase PTPn3 interacts with 14-3-3β adaptor to regulate its activity [694] as well as metallopeptidase ADAM17 to prevent its action [695].52 Moreover, PTPn3 shifts activity of voltage-gated sodium channel Na V 1.5 toward hyperpolarized potentials in cardiomyocytes [696]. Phosphatase PTPn3 also dephosphorylates T-cell receptor TCRζ subunit [697].
8.3.11.4 Non-Receptor Protein Tyrosine Phosphatase-4
Non-receptor protein Tyr phosphatase-4 (PTPn4)53 is encoded by the PTPN4 gene. Like PTPn3, this testis-enriched phosphatase contains a C-terminal phosphatase domain and N-terminal motif homologous to band 4.1 superfamily of cytoskeletal-associated proteins. It is prominently expressed in cerebellar Purkinje and thalamus cells [698].
Phosphatase PTPn4 interacts with δ2 and ε subunits of the heteromeric glutamate receptor, the predominant excitatory neurotransmitter receptors in the brain [698]. Glutamate receptor GluRδ2 is selectively synthesized in cerebellar Purkinje cells. Phosphatase PTPn4 in thalamus cells enhances Fyn-mediated Tyr phosphorylation of N-methyl-D-aspartate receptor GluN2a (or GluRε1).
8.3.11.5 Non-Receptor Protein Tyrosine Phosphatase-5
Brain-specific non-receptor protein Tyr phosphatase-5 (PTPn5) is highly enriched within dopaminergic neurons (soma, axons, and dendrites) in the striatum, neocortex, amygdala and other related regions relatively to other areas of the central nervous system [699, 700].54 It operates in the rapid integration of adrenergic, dopaminergic, and glutamatergic signaling. Cytosolic PTPn5 is related to transmembrane PTPRr phosphatase. Both are mainly expressed in neurons.
Four major PTPn5 isoforms exist (20-, 38-, 46-, and 61-kDa PTPn5). However, only two isoforms — PTPn546 and PTPn561 — are active, as they contain a phosphatase domain [700].
Phosphatase PTPn5 localizes to postsynaptic densities, which are specialized parts of the cytoskeleton at neuronal synapses in striatal neurons, where it binds to and dephosphorylates non-receptor protein Tyr kinase Fyn (Tyr420) [701]. In addition, PTPn5 is a substrate and inactivator of extracellular signal-regulated kinases ERK1 and ERK2 [702].
Several post-translational modifications regulate PTPn5 activity, such as phosphorylation, ubiquitination and proteasomal degradation, and proteolysis.55 Phosphatase PTPn5 contributes to the regulation of endocytosis of NMDA-type glutamate receptors.
Phosphatase PTPn5 is phosphorylated by protein kinase-A and dephosphorylated by protein phosphatases PP1 and PP3 [700]. Phosphatase PTPn5 dephosphorylates NMDA-type glutamate receptors GluN2b, thereby causing NMDAR internalization. It also targets GluR2 subunit of AMPA-type glutamate receptors, MAPKs (ERK1, ERK2, P38MAPK, and JNK), in addtion to Fyn kinase [700].
8.3.11.6 Non-Receptor Protein Tyrosine Phosphatase-6
SH2 domain-containing, non-receptor protein Tyr phosphatase-6 (PTPn6)56 is encoded by the PTPN6 gene. Its N-terminus contains 2 tandem Src homolog-2 (SH2) binding domains. Multiple alternatively spliced transcript variants generate distinct isoforms.
Phosphatase PTPn6 is expressed primarily in hematopoietic cells, where it participates in multiple signaling pathways that regulate cell growth, differentiation, and proliferation, especially during hematopoiesis. However, it is ubiquitous and can be particularly detected in neurons and epithelial cells.
In cells, it resides in the cytoplasm and nucleus. In particular, in neurons, it translocates into the nucleus upon angiotensin-2 stimulation. Its post-translational modifications include phosphorylations on Ser and Tyr residues.
Phosphatase PTPn6 impedes signaling from receptor Tyr kinases, adhesion receptors, cytokine receptors, and immunoreceptors, in particular receptors Ros1 in the proximal segment of the epididymis [703], EGFR [704], SCFR [705], and EpoR [706], as well as Fc receptor-like-3, inhibitory leukocyte-associated immunoglobulin-like receptor LAIR1 [708],57 and leukocyte immunoglobulin-like receptors LILRb2 and LILRb4, adhesion molecules PECAM-1 and catenin-δ1, and sialic acid-binding Ig-like lectin Siglec-2.58
Phosphatase PTPn6 controls TCR signaling thresholds in both developing and mature T lymphocytes. It interacts with receptor and cytoplasmic Tyr kinases (Abl, FAK2, JaK2, LCK, Lyn, SYK, and TyK2) for activation or, most often, inactivation of protein kinase-Cδ [707], adaptor GRB2, Homeobox-A10 transcription factor, and scaffold signal-regulatory protein-α.
In cooperation with mitochondrial (or microtubule-associated) tumor suppressor MTuS159 that interacts with angiotensin-2 receptor AT2, PTPn6 upregulates ubiquitin conjugase variant UbE2V2 upon angiotensin-2 stimulation.
8.3.11.7 Non-Receptor Protein Tyrosine Phosphatase-7
Non-receptor protein Tyr phosphatase-7 (PTPn7),60 encoded by the PTPN7 gene, is predominantly expressed in hematopoietic cells. Its N-terminus contains a kinase interaction motif (KIM) used by PKA to phosphorylate PTPn7 and to tether ERK kinase. Two alternatively spliced transcript variants encode 2 isoforms.
Phosphatase PTPn7 can form a cholesterol-regulated complex with PP2 that has dual-specificity due to the combined activities of Ser/Thr phosphatase PP2 and Tyr phosphatase PTPn7, hence being able to dephosphorylate ERK1 and ERK2 [709]. Acute depletion of cholesterol causes the disassembly of the PP2–PTPn7 complex.61
Phosphatase PTPn7 is closely related to receptor PTPRr and cytoplasmic PTPn5 PTPs that target ERK1/2 and P38MAPKs. Its non-catalytic N-terminus can interact with MAPKs to suppress their activities. In addition to Ser/Thr phosphatases PP1 and PP2, dual-specificity MAPK phosphatases, PTPn7 also binds and dephosphorylates P38MAPK and ERK enzymes.62 Phosphatase PTPn7 regulates ERK1 and ERK2 nuclear translocation, rather than ERK activity [710].
8.3.11.8 Non-Receptor Protein Tyrosine Phosphatase-8
Non-receptor protein Tyr phosphatase-8 (PTPn8) that is encoded by the PTPN8 gene is also identified as cytoplasmic protein Tyr phosphatase-22 (PTPn22). Alternative splicing transcript variants generate 2 isoforms.
8.3.11.9 Non-Receptor Protein Tyrosine Phosphatase-9
Non-receptor protein Tyr phosphatase-9 (PTPn9)63 is encoded by the PTPN9 gene. It is expressed in some hematopoietic cell lineages such as neutrophils, where it is mainly associated with the cytoplasmic face of granules and secretory vesicles [711]. Its non-catalytic N-terminus negatively regulates the enzymatic activity of the C-terminal phosphatase domain.
The N-terminus of PTPn9 phosphatase is homologous to lipid-binding proteins involved in intracellular transport such as retinaldehyde-binding protein RlBP1 (also known as cellular retinaldehyde binding protein [CRalBP]) and members of the SEC14 cytosolic factor family. This domain can serve as a secretory vesicle targeting signal. The Sec14 domain is responsible for PTPn9 localization to the perinuclear region.
8.3.11.10 Non-Receptor Protein Tyrosine Phosphatase-11
Ubiquitous, Src homology-2 (SH2) domain-containing, non-receptor protein Tyr phosphatase-11 (PTPn11)65 is encoded by the gene PTPN11 in all hematopoietic cells as well as other cell types. Its N-terminus contains 2 tandem SH2 domains (NSH2 and CSH2) that are TyrP-binding sites. In its inactive state, its N-terminus attaches to PTP domain and blocks access of substrates to the active site (auto-inhibition).
Phosphatase PTPn11 interacts with receptors of hormones, such as insulin and growth hormone [713], growth factors, such as EGF, PDGF, and IGF1, cytokines ( SCFR and IL6Rβ subunit [a.k.a. glycoprotein GP130 and CD130]) [714], some immunoreceptors (e.g., leukocyte-associated immunoglobulin-like receptor LAIR1, or CD305), as well as insulin receptor substrate IRS1, Janus kinases JaK1 and JaK2 (but not JaK3) [715], transcription factors STAT5a and STAT5b [716], suppressor of cytokine signaling SOCS3, kinase FAK2, phospholipase-Cγ2 [717], adaptors GRB2-associated binders GAB1 and GAB2, CBL, and adhesion molecule PECAM1.
Mutations of the PTPN11 gene are the most frequent cause of juvenile myelomonocytic leukemia characterized by a selective hypersensitivity of hematopoietic progenitors to granulocyte–macrophage colony-stimulating factor (CSF2). In more than 75% of cases, mutually exclusive gain-of-function mutations appear in N-RAS, K-RAS, or PTPN11 or homozygous loss-of-function mutations in NF1 (RASGAP), i.e., mutations in genes that encode components of the Ras–ERK cascade. Protein PTPn11 also operates in signal transduction of many receptors involved in aging, such as GHR.
Phosphatase PTPn11 intervenes in embryogenesis, as it can regulate cell survival, differentiation, proliferation ,and migration using the ERK1/2 pathway that is transiently activated during early embryogenesis. Mutations in PTPN11 gene also cause Noonan syndrome often characterized by cardiac disease, craniofacial abnormalities, growth impairment, and a disturbance in IGF1 signaling.66 Whereas mutations associated with Noonan syndrome often generate gain of ERK1/2 function, Leopard syndrome also associated with PTPN11 mutations leads to loss of ERK1/2 function.
Neural crest cells (the fourth germ layer) participate in both heart and skull formation. Neural crest cell determination, proliferation, migration, and differentiation are controlled by sonic Hedgehog, Wnts, bone morphogenic proteins and fibroblast growth factors. Furthermore, PTPn11 is essential for normal migration of neural crest cells and differentiation into the diverse lineages of heart and skull [718].67
8.3.11.11 Non-Receptor Protein Tyrosine Phosphatase-12
Non-receptor protein Tyr phosphatase-12 (PTPn12),68 encoded by the PTPN12 gene, belongs to the PEST family of protein Tyr phosphatases with PTPn18, PTPn22, and PTPn23 phosphatases. Phosphatase PTPn12 resides in non- immunocytes, but abounds in hematopoietic cells.
Phosphorylated focal adhesion-associated proteins and dephosphorylation of corresponding phosphoproteins contribute to the regulation of the cytoskeleton dynamics and control of signals for cell adhesion, migration, growth, and survival. In non-hematopoietic cells, PTPn12 is a potent regulator of adhesion and migration.
Phosphatase PTPn12 prominently associates with cytoskeletal proteins (CAS and CAS-related proteins CAS family member-3 (CAS3) and CASL, paxillin and paxillin-related polypeptides TGFβ1-induced transcript-1 TGFβ1-169 and leupaxin) as well as kinases and adaptors (CSK, SHC, and GRB2).
Phosphatase PTPn12 dephosphorylates Abl kinase, as well as various cytoskeleton and cell-adhesion molecules, such as focal adhesion-associated adaptor BCAR1 (a.k.a. CAS and p130CAS), WASP, kinases FAK1 and FAK2 (Sect. 4.6), Pro–Ser–Thr phosphatase-interacting protein PSTPIP1, Vav GEF, RhoGAP35, and paxillin [719].
Phosphatase PTPn12 participates not only in cytoskeletal reorganization for cell adhesion and migration, but also inhibition of lymphocyte activation [720]. It dephosphorylates a subset of BCR- and TCR-regulated substrates, such as adaptors (e.g., SHC and BCAR1 [CAS]) and kinases (e.g., FAK and PYK2 that intervene with Lyn, SYK, and BTK in B-cell receptor signaling; Lck, Fyn, and ZAP70 for T-cell receptor signaling).
8.3.11.12 Non-Receptor Protein Tyrosine Phosphatase-13
Ubiquitous non-receptor protein Tyr phosphatase-13 (PTPn13),70 encoded by the PTPN13 gene, dephosphorylates TNFRSF6a receptor. Four alternatively spliced transcript variants exist. Like PTPn3 and PTPn4, PTPn13 belongs to the group of PTPs characterized by an N-terminal segment homologous to proteins of the band 4.1 superfamily, PDZ (PSD95, Disc large, zonula occludens) domains, and a PTPase catalytic motif.
Phosphatase PTPn13 interacts via its PDZ domain with PAK271 that is regulated by small GTPase Rho [721]. Both PTPn13 and PAK2 colocalize in lamellipodium-like structures, where they can contribute to the regulation of the actin cytoskeleton. As it interacts with GTPase-activating proteins, it can operate as a regulator of Rho signaling. Phosphatase PTPn13 also dephosphorylates IκBα agent.
8.3.11.13 Non-Receptor Protein Tyrosine Phosphatase-14
Non-receptor cytoskeletal-associated protein Tyr phosphatase-14 (PTPn14)72 is encoded by the PTPN14 gene. Like PTPn3, PTPn4, and PTPn13, it belongs to the group of PTPs characterized by an N-terminal segment homologous to proteins of the band 4.1 superfamily, PDZ domains, and a PTPase catalytic domain. Hence it is a member of the FERM (four-point-one, ezrin, radixin, moesin) family of PTPs. It localizes to adherens junctions, where it dephosphorylates β-catenin [722]. It is synthesized in many human organs, such as lung, kidney, skeletal muscle, and placenta. It is phosphorylated upon DNA damage, probably by ATMK or ATRK kinase.
8.3.11.14 Non-Receptor Protein Tyrosine Phosphatase-18
Non-receptor protein Tyr phosphatase-18 (PTPn18)73 is encoded by the PTPN18 gene. Like PTPn12 and PTPn22, it contains a PEST (proline-, glutamic acid-, serine-, and threonine-rich domain) motif. It is expressed in hematopoietic cells as well as other cell types, especially in brain and colon.
Like PTPn12, PTPn18 links to cytoskeleton-associated Pro–Ser–Thr phosphatase-interacting proteins PSTPIP1 and PSTPIP2 [723].74 It can be a positive and negative regulator of cytoskeletal rearrangement and inflammation, respectively. It can be phosphorylated by PKA or PKC as well as Fyn or TEC. It can associate with C-terminal Src kinase that inactivates Src kinases. This association can enable its recruitment in the vicinity of CSK targets, in particular Src kinases [720].
8.3.11.15 Non-Receptor Protein Tyrosine Phosphatase-20
Non-receptor protein Tyr phosphatase-20 (PTPn20)75 contains a single catalytic domain at its C-terminal half and an N-terminal half with a region rich in PEST motifs [724]. It is specifically expressed in mouse testicular germ cells that undergo meiosis. It is detected between week 2 and 3 after birth with the onset of meiosis.
In mammals, spermatogenesis happens in the seminiferous tubule, in which mitotic division of spermatogonia, meiosis of spermatocytes, and differentiation of spermatid to sperm occurs. Many enzymes are specifically produced before or at meiosis, such as Tyr kinases SCFR (Vol. 3 – Chap. 8. Receptor Kinases), Abl (Sect. 4.2), and Fer (Sect. 4.7), Ser/Thr kinases male germ cell-associated kinase (MAK), v-mos Moloney murine sarcoma viral oncogene homolog Mos (a MAP3K; Sect. 6.5.2), and TesK (Sect. 5.9.4), dual-specific Never in mitosis gene-A-related kinase Nek1, receptor protein Tyr phosphatase PTPRv, Ser/Thr phosphatase calcineurin-B subunit isoformβ1 (PP3r1), and dual-specific phosphatase CDC25, in addition to cytoplasmic protein Tyr phosphatase PTPn20 [724].
8.3.11.16 Non-Receptor Protein Tyrosine Phosphatase-21
Non-receptor protein Tyr phosphatase-21 (PTPn21)76 is encoded by the PTPN21 gene. Like PTPn3, PTPn4, and PTPn13, PTPn21 belongs to the group of PTPs characterized by an N-terminal segment homologous to proteins of the band 4.1 superfamily, PDZ domains, and a PTPase catalytic domain.
Phosphatase PTPn21 interacts with BMX kinase of the TEC kinase family. It localizes along actin filaments and to adhesion plaques. It can form distinct complexes with actin, Src kinase, and focal adhesion kinase to cause cell adhesion and migration [725].
8.3.11.17 Non-Receptor Protein Tyrosine Phosphatase-22
Non-receptor protein Tyr phosphatase-22 (PTPn22)77 is encoded by the PTPN22 gene (or PTPN8 gene). In humans, PTPN22 polymorphism is a significant risk factor for autoimmune diseases, such as type-1 diabetes, rheumatoid arthritis, juvenile idiopathic arthritis, myasthenia gravis, and systemic lupus erythematosus.
Phosphatase PTPn22 interacts with adaptors CBL and GRB2, as well as kinases CSK, Fyn, LCK, and ZAP70 [720]. It prevents T-cell activation, mainly by suppressing the activity of SRC family PTKs via CSK kinase.
8.3.11.18 Non-Receptor Protein Tyrosine Phosphatase-23
Non-receptor protein Tyr phosphatase-23 (PTPn23)78 is encoded by the PTPN23 gene. It possesses an N-terminal domain, a Tyr phosphatase motif, and a PEST sequence at its C-terminus. Phosphatase PTPn23 participates in sorting cargo proteins to endosomes.
Endothelial cells synthesize various transmembrane and cytoplasmic PTPs, such as PTPRb (VE-PTP), PTPRe, PTPRj, PTPRm, and PTPn23, in addition to RPTKs, such as FGFR, PDGFR, and VEGFR1 and -2, and NRTRKs, such as Src, JaK, and FAK. Endothelial cell migration during angiogenesis (Vol. 5 – Chap. 10. Vasculature Growth) is controlled via phosphorylation and dephosphorylation of different substrates by coordinated kinases and phosphatases. Phosphatase PTPn23 binds focal adhesion kinase [726]. Fibroblast growth factor FGF2 that stimulates endothelial cell migration precludes PTPn23–FAK attachment. On the other hand, Src kinase favors cell migration, as it phosphorylates FAK, BCAR1, and E-cadherin. In addition, Src phosphorylates (inactivates) PTPn23 [727]. Fibroblast growth factor controls Src–PTPn23 interaction, as it activates Src kinase.
8.3.12 PEST Family Phosphatases in Inflammation and Immunity
The PEST (proline-, glutamic acid-, serine- and threonine-rich) family of cytoplasmic protein Tyr phosphatases includes PTPn12, PTPn18, PTPn22, and PTPn23. T-cell activation results from binding of an antigen with a major histocompatibility complex molecule that is displayed to a T-cell antigen receptor. Class-1 and -2 MHCs interacts with CD8 and CD4, respectively, thereby building receptor complexes. These associations trigger phosphorylation initiated by Src family PTKs and amplified by SYK family PTKs. Molecules of the major histocompatibility complex are dispensable for B-cell antigen receptors and receptors recognizing Fc-receptor-binding part of immunoglobulins, but these receptors prime equivalent signalings.
Phosphatase PTPn12 is implicated with kinases of the Src family members, FAK1/2, and PTPn1 in cytoskeletal reorganization for leukocyte adhesion and migration as well as granule exocytosis and phagocytosis [720]. In addition, it hampers lymphocyte activation, as it can dephosphorylate TCR- and BCR-regulated substrates. Conversely, PTPn12 is downregulated during T-cell activation. Phosphorylation of PTPn12 by PKC and PKA impedes PTPn12 activity.
Phosphatase PTPn18 can contribute to inhibition of inflammation. It promotes cytoskeleton rearrangement. Kinases of the SRC and TEC families can phosphorylate PTPn18, thereby inactivating PTPn18 [720].
Phosphatase PTPn22 links to CSK to cooperatively inhibit activation of both CD4 + and CD8 + mature (effector and memory) T lymphocytes, but not naive T lymphocytes [720]. Phosphatase PTPn22 can also regulate BCR-triggered responses in immature B lymphocytes. Phosphorylation of PTPn22 by PKC reduces PTPn22 activity.
8.3.13 Dual-Specificity Protein Phosphatases
Dual-specificity phosphatases (DuSP) form a heterogeneous hyperfamily of protein phosphatases that can dephosphorylate SerP, ThrP, and TyrP residues within a given substrate.
These phosphatases constitute 7 families on the basis of sequence similarity: (1) mitogen-activated protein kinase (MAPK) phosphatases (MKP) that dephosphorylate (inactivate) MAPKs; (2) Slingshots; (3) protein Tyr phosphatases-4A that are also termed phosphatases of regenerating liver; (4) cell-division cycle-14 homolog phosphatases; (5) phosphatase and tensin homologs; (6) myotubularins; and (7) atypical dual-specific phosphatases.
8.3.13.1 Lipid and Protein Phosphatases – PTen and PTen-like Phosphatases
Plasmalemmal phosphatase and tensin homolog deleted on chromosome 10 (PTen; Sect. 2.10.1)79 possesses a tensin-like domain as well as a catalytic domain similar to that of dual-specificity protein phosphatases. However, cytoplasmic PTen preferentially dephosphorylates phosphoinositide substrates. It is called a tumor suppressor, as this phosphoinositide phosphatase restricts the activity of phosphatidylinositol 3-kinase by dephosphorylating PI(3,4,5)P3 to PI(4,5)P2, thereby impeding the PI3K–PKB pathway.
Phosphatase PTen is both a phosphatidylinositol 3-phosphatase and a protein phosphatase that is able to dephosphorylate SerP, ThrP, and TyrP, residues. It indeed dephosphorylates Tyr-phosphorylated focal adhesion kinases. Phosphatidylinositol 3-kinase interacts with FAK in PKB phosphorylation. Protein phosphatase-1δ is also located in focal adhesions and associates with focal adhesion kinases.
Phosphatase PTen interacts with adaptor NHERF80 and platelet-derived growth factor receptors [728]. Ubiquination regulates PTen stability and its nuclear location, where PTen favors chromosome integrity [729]. Polyubiquination degrades PTen, whereas nuclear monoubiquitination stabilizes PTen. PTen acts on chromatin and regulates expression of small GTPase Rad51 that reduces the incidence of spontaneous DNA double-strand breaks.
Phosphorylation represents a mode of regulation of PTen activity. Phosphatase PTen is a substrate of Src family protein Tyr kinases. It modulates the activity of the PI3K–PKB axis, whereas SH2 domain-containing protein Tyr phosphatase PTPn6 binds and dephosphorylates PTen. Casein kinase CK2 also targets PTen [730].
Five PTen-like phosphatases dephosphorylate D3-phosphorylated inositol phospholipids, hence contributing to reversible phosphorylation of inositol lipids that serves in cell signaling. Some myotubularin phosphatases also dephosphorylate D3-phosphorylated inositol phospholipids.
8.3.13.2 PTEN Superfamily
Members of the PTEN superfamily, PTen, tensins, and transmembrane phosphatases with tensin homology, are encoded by 5 genes (Table 8.31).
Table 8.31
Members of the PTEN superfamily (C1Ten: C1 domain-containing phosphatase and tensin homolog; MMAC: mutated in multiple advanced cancers; MxRA: matrix-remodeling associated protein; TenC: tensin-like C1 domain-containing phosphatase; Tns: tensin; TPIP: TPTE and PTen homologous inositol lipid phosphatase; TPTe: transmembrane phosphatase with tensin homology, or transmembrane phosphoinositide 3-phosphatase and tensin homolog).
Type | Other aliases |
---|---|
PTen | MMAC1, TEP1 |
Tns1 | MST091, MSTP091, MST122, MSTP122, MST127, MSTP127, MxRA6 |
Tns2 | C1Ten, TenC1 |
TPTe1 | TPTe, PTen2 |
TPTe2 | TPIP |
Tensin-1
Tensin-1 encoded by the TNS1 gene localizes to focal adhesions,81 more precisely fibrillar adhesions.82 Tensin-1 is involved in integrin removal from focal adhesions and transfer to fibrillar adhesions as well as in fibronectin fibrillogenesis.83 This protein binds to and crosslinks actin filaments. However, tensin-1 can reduce the actin polymerization rate by interacting with the barbed end of actin. Tensin has a protein Tyr phosphatase domain related to PTen, an Src homology-2 (SH2) motif, and a TyrP-binding sequence (PTB).
Platelet-derived growth factor, thrombin, angiotensin, among others, provoke Tyr phosphorylation of tensin-1. Both Abl and Src kinases phosphorylate tensin-1 [731]. It is a substrate of calpain-2, a focal adhesion peptidase involved in disassembly of focal adhesions. Therefore, tensin-1 links the extracellular matrix and the actin cytoskeleton to signal transduction.
Protein Ser/Thr phosphatase PP1 resides also in focal adhesions. Among the 3 predominant, widespread PP1 isoforms, PP1β (or PP1δ) can link to focal adhesion kinase. On the other hand, PP1α tethers to tensin [732].
Tensin-2
Tensin-2 is strongly produced in the heart, kidney, skeletal muscle, and liver. Three transcript variants generate 3 isoforms. Tensin-2 also localizes to focal adhesions. Both tensin-1 and -2 are able to promote cell migration [731]. Tensin-2 binds to Axl receptor Tyr kinase [733]. This intracellular phosphatase precludes PKB signaling.
Transmembrane Phosphatases with Tensin Homology
The human transmembrane phosphatase with tensin homology genes (TPTE1–TPTE2) encode PTen-related Tyr phosphatases TPTe1 and TPTe2. These 2 phosphatases are observed in secondary spermatocytes and/or prespermatids [734]. Several alternatively spliced isoforms of these 2 proteins possess a variable number of transmembrane domains among the 4 potential transmembrane domains. Phosphatase TPTe2 is a membrane-associated phosphatases with substrate specificity for the 3-position phosphate of inositol phospholipids.
8.3.13.3 Mitogen-Activated Protein Kinase Phosphatases
Non-dual-specificity mitogen-activated protein kinase phosphatases include protein Ser/Thr phosphatases PP2 and PPM1 as well as receptor-like PTPRr and cytoplasmic PTPn5 and PTPn7 protein Tyr phosphatases.
Dual-specificity MAPK phosphatases (DuSP/MKP) dephosphorylate (inactivate) both ThrP and TyrP on activated MAPKs, i.e., extracellular-signal-regulated kinases, Jun N-terminal kinases, and P38MAPKs (Table 8.32; Chap. 6), thereby antagonizing MAPK signaling and contributing to the setting of the magnitude and duration of MAPK activation. They are involved in development, metabolism, immunity, and cellular stress responses.
Table 8.32
Types of mitogen-activated protein kinase phosphatases and their specific substrate with order of potency (Sources: [735–737]; KAP: kinesin-associated protein). Substrate specificity of MKPs can differ in vivo from that in vitro. Dual-specificity MKPs constitute a structural superfamily of 11 proteins within the hyperfamily of dual-specificity protein phosphatases. This subset encompasses 10 enzymes and DuSP24 pseudophosphatase.
Type | Substrates (potency order) |
---|---|
MKP1 (DuSP1) | ERK < P38MAPK ∼ JNK |
MKP2 (DuSP4) | P38MAPK < ERK ∼ JNK |
MKP3 (DuSP6) | P38MAPK ∼ JNK < ERK |
MKP4 (DuSP9) | JNK < P38MAPK < ERK |
MKP5 (DuSP10) | ERK < P38MAPK ∼ JNK |
MKP6 (DuSP14) | ERK, JNK |
MKP7 (DuSP16) | ERK < P38MAPK ∼ JNK |
MKP8 (DuSP26) | ERK, JNK, P38MAPK, PKB, KAP3 |
DuSP2 | JNK < P38MAPK ∼ ERK |
(in vivo, preferentially JNK) | |
DuSP3 | ERK, JNK, P38MAPK, STAT5 |
DuSP5 | ERK |
DuSP7 | P38MAPK ∼ JNK < ERK |
DuSP8 | ERK < P38MAPK ∼ JNK |
DuSP18 | JNK |
DuSP19 | JNK |
DuSP22 | ERK, JNK, P38MAPK, ERα, STAT3 |
DuSP23 | ERK, JNK, P38MAPK |
According to sequence similarity, gene structure, substrate specificity, subcellular location, and transcriptional regulation, the MKP superfamily can be subdivided into 3 families [738, 739]: (1) family 1 that comprises 4 inducible, nuclear MKPs (DuSP1, DuSP2, DuSP4, and DuSP5); (2) family 2 with 3 related, cytoplasmic, ERK-specific MKPs (DuSP6, DuSP7, and DuSP9); and (3) family 3 also with 3 MKPs (DuSP8, DuSP10, and DuSP16). that preferentially inactivate stress-activated MAPKs, i.e., P38MAPKs and JNKs.
Except DuSP5 that targets ERK1 and ERK2, subfamily-1 MKPs display a rather broad specificity for ERK, JNK, and P38MAPKs. All of these DuSPs are encoded by inducible genes that are rapidly upregulated in response to mitogenes and cell stresses.
Pseudophosphatase mitogen-activated protein kinase (MAPK) SerP (S)/ThrP (T)/TyrP (Y)-interacting (X) protein (MKSTYX), or STYX-like protein (STYXL1), that is characterized by a substitution of Ser for Cys in the catalytic domain is included in MKP subfamily on the basis of domain structure [736].
Numerous MKPs have overlapping substrate specificities. These enzymes interact specifically with their substrates via a kinase-interaction motif (KIM) located within the non-catalytic, N-terminal domain of the protein. Binding of MAPKs to MKPs often primes activation of the C-terminal catalytic domain, thereby ensuring substrate specificity.
Four types of DuSP/MKP proteins can be defined according to their structure [741]. Classical MKPs possess a DuSP domain and a MAPK-binding domain (MKB), whereas atypical DuSPs that still function as MKP (e.g., DuSP3, DuSP14, and DuSP22) are much smaller proteins that lack a MAPK-binding domain. Type-1 DuSP/MKPs are characterized by a single PTP domain between their C- and N-termini. They include DuSP3, DuSP14, DuSP15, and DuSP22. Type-2 DuSP/MKPs contain a PTP and a MKB domain. They encompass DuSP1, DuSP2, DuSP4 to DuSP7, and DuSP9. Type-3 DuSP/MKP represented by a single element DuSP10 possesses a unique N-terminal NT domain in addition to MKB and PTP motifs. Type-4 DuSP/MKPs, such as DuSP8 and DuSP16, are constituted by NT, MKB, PTP, and PEST (proline-, glutamine-, serine-, threonine-rich) sequences.
Therefore, type-2 DuSP/MKPs are split into MKP subfamilies 1 and 2, whereas type-3 and -4 DuSP/MKPs constitute the MKP subfamily 3.
The MKP phosphatases possess an N-terminal rhodanese or CDC25 homology-2 domain (CH2) with kinase-interacting motifs that confer MAPK substrate specificity for one or more MAPKs among ERKs, JNKs, and P38MAPKs. Members of the MKP subfamily are listed in Tables 8.33 and 8.34. Many MKPs are inducible and have a low expression level in unstressed cells. Their expression rapidly heightens upon stimulation by growth factors or cell stresses. Their production kinetics and magnitude can depend on cell type and context.
Table 8.33
The DuSP hyperfamily of phosphatases with its members of the MKP superfamily (Part 1; Sources: [736, 739, 740]; VH1: virus-encoded protein homolog, i.e., vaccinia virus VH1 gene product). The set of MAPK phosphatases also include protein Ser/Thr phosphatases PP2 and PPM1 as well as receptor-like protein Tyr phosphatase PTPRr and cytoplasmic types PTPn5 and PTPn7. Various synonyms exist for some of the DuSP genes or proteins. The MKP list takes into account Human Genome Organisation (HUGO) nomenclature. Mitogen-activated protein kinase phosphatases are classified into 2 main families according to intracellular location and transcriptional regulation. Family-1 MKPs are primarily located in the nucleus and encoded by immediate-early genes. They include MKP1, MKP2, DuSP2, and DuSP5. The expression of the nuclear MKPs is induced shortly after cell stimulation by growth factors and stresses. Family-2 MKPs are located either primarily in the cytoplasm or in both the cytosol and nucleus. They are characterized by slower kinetics. Mitogen-activated protein kinase phosphatases, which are specific for MAPKs, could also serve as anchors for MAPKs and control their intracellular location.
Name | Alias | Subcellular | Substrates | Stimuli |
---|---|---|---|---|
localization | ||||
DuSP1 | PTPn10, MKP1, | Nuclear | ERK, JNK, | Hypoxia, LPS, |
CL100, VH1 | P38MAPK | heat shock | ||
Oxidative stress | ||||
DuSP2 | PAC1 | Nuclear | ERK, P38MAPK | Growth factors, |
> JNK | heat shock, LPS | |||
DuSP4 | MKP2, VH2, | Nuclear | ERK > JNK | Growth factors, |
TYP, STY8 | > P38MAPK | Other mitogens | ||
DuSP5 | DuSP, VH3, | Nuclear | ERK | Growth factors, |
B23 | IL2, heat shock | |||
DuSP6 | MKP3, PYST1, | Cytosolic | ERK > JNK | |
VH6 | = P38MAPK | |||
DuSP7 | MKPx, PYST2, | Cytosolic | ERK > P38MAPK | |
B59 | ||||
DuSP8 | VH5, VH8, | Nuclear, | JNK > P38MAPK | |
HB5, C11ORF81 | cytosolic | > ERK | ||
DuSP9 | MKP4, PYST3 | Nuclear, | ERK > P38MAPK | |
cytosolic | = JNK |
Name | Alias | Subcellular | Substrates | Stimuli |
---|---|---|---|---|
localization | ||||
DuSP10 | MKP5 | Nuclear, | JNK = P38MAPK | Peptidoglycans, |
cytosolic | > ERK | LPS | ||
DuSP14 | MKP6, MKPL | Nuclear, | ERK, JNK, | |
cytosolic | P38MAPK | |||
DuSP16 | MKP7, MKPm | Cytosolic | JNK2/3, | |
P38MAPKαβ | ||||
DuSP18 | Nuclear, | JNK | ||
cytosolic | ||||
DuSP22 | JKAP, JSP1, | JNK | ||
VHx | ||||
DuSP26 | MKP8, SKRP3, | Nuclear, | ERK, | |
NEAP | cytosolic | P38MAPK | ||
STYXL1 | DuSP24, MKSTYX |
The MKP enzymes bear post-translational modification. As the catalytic cleft possesses a cysteine residue, MKPs can undergo reversible oxidation (inactivation). Certain MKPs are inducible, others are stabilized or destabilized by phosphorylation. Phosphorylation of MKPs can lead to their stabilization by attenuating ubiquitination and subsequent degradation. Isozymes MKP1 to MKP3 and MKP7 are regulated by phosphorylation.
In fact, MKPs can be phosphorylated by their substrates: DuSP1 is phosphorylated by ERK (Ser359 and Ser364) and DuSP16 by P38MAPK (Ser446) [736]. Phosphorylation by ERK stabilizes MKP1 and increases its half-life, whereas it elicits MKP3 degradation [735]. However, the interaction of MKP3 with its specific substrate ERK2 enhances its activity. Furthermore, MKPs can be epigenetically regulated via promoter methylation or chromatin modification. Upon Toll-like receptor stimulation, histones associated with DuSP1 are acetylated.
The MAPK enzymes target numerous substrates, such as transcription factors of the ATF–CREB and AP1 family, kinases MAPKAPK2 and RSK, and controllers of mRNA stability and translation.
MKPs in Cell Metabolism
Upon insulin stimulation, liganded insulin receptor triggers the PI3K–PKB pathway for glucose uptake. This pathway possesses upstream mediators, insulin receptor substrates. The latter serves as a negative feedback node.
Phosphatase DuSP1 (MKP1) regulates multiple MAPK pathways in the nucleus, thereby controling the expression of genes involved in fatty acid metabolism and energy expenditure. As cytosolic MAPKs are not subjected to inducible, nuclear MKP1, negative feedback exerted by phosphorylation of IRS by MAPK kinases does not operate.
MKPs in Development
Members of the subfamily-2, cytoplasmic, ERK-specific MKPs (DuSP6, DuSP7, and DuSP9) inactivates mainly ERK1 and ERK2. Phosphatase DuSP6 is an inhibitor of fibroblast growth factor signaling. Phosphatase DuSP9 intervenes in placental development and function [738].
MKPs in Stress Response
Members of the MKP subfamily 3 (DuSP8, DuSP10, and DuSP16) target stress-activated MAPKs (JNKs and P38MAPKs). In addition, DuSP1 promotes cell survival, as it attenuates effects of stress-activated MAPK pathways. In response to oxidative stress, the P53 pathway upregulates DuSP2 [738].
MKPs in Immunity
The MKP phosphatases can elicit synthesis of cytokines and chemokines. Activation of ERK results from TLR2 stimulation and leads to the production of anti-inflammatory IL10, whereas P38MAPK activation is caused by excitated TLR4, TLR5, or TLR9 to provoke IL12 synthesis.
Phosphatases DuSP1, DuSP2, and DuSP10 regulate immune function. Activated B and T lymphocytes, mastocytes, eosinophils, macrophages, and dendritic cells, are characterized by high concentrations of nuclear MKPs (DuSP1, DuSP2, DuSP4, and DuSP5 [MKP subfamily 1]). Upon activation by a set of TLR ligands, DuSP1, DuSP2, and DuSP16 are the most strongly induced MKPs in macrophages [741] (Table 8.35).
DuSP type | Cell types |
---|---|
DuSP1 | Macrophages, mastocytes, microglial cells, fibroblasts |
DuSP2 | B and T cells, neutrophils, mastocytes, macrophages |
DuSP5 | T cells, fibroblasts |
DuSP10 | Macrophages, T cells |
Phosphatase DuSP1 controls macrophage function, inflammatory response to TLR signaling, and both innate and adaptive immune responses by inactivating P38MAPK and JNK [736]. In macrophages, lipopolysaccharides signal via Toll-like receptors that recruit MyD88 and TRIF adaptors to launch an early response using P38MAPKs and JNKs characterized by the production of pro-inflammatory TNF cytokine. This reaction is followed by synthesis of MKP1 that then prevents P38MAPK and JNK activities to avoid overproduction of cytokines, hence endotoxic shock. Later, when MKP1 level declines, P38MAPKs and JNKs promote the synthesis of anti-inflammatory IL10 [738].
Among inducible, nuclear MKPs of the subfamily 1, DuSP2 is the most closely associated with immunocytes. Phosphatase DuSP2 not only impedes directly ERK and P38MAPK signaling, but also operates in JNK activity. In fact, it favors immune response via crosstalk between JNK and ERK pathways [738].
Phosphatase DuSP10 modulates gene expression in innate immunocytes. It precludes inflammation by inactivating JNK kinase. Whereas DuSP10 mainly operates on JNK signaling in immune cells, DuSP1 that influences the activity of all 3 MAPK types predominantly acts via the P38MAPK pathway [738].
DuSP1 – MKP1
Dual-specificity phosphatase-1 (DuSP1)84 links to ERK1, ERK2, JNK1 to JNK3, and P38MAPKα (i.e., MAPK1, MAPK3, MAPK8–MAPK10, and MAPK14 [251]. Its other partners include CDC28 protein kinase regulatory subunit CKS1b, S-phase kinase-associated protein SKP2, and ubiquitin-C.
Phosphatase DuSP1 inhibits immune function, participates in metabolic homeostasis, and mediates resistance to cellular stress [739]. Activity of MKP1 is elicited by TLR stimulation via both myeloid differentiation factor MyD88, TIR domain-containing adaptor inducing Ifnβ(TRIF), and P38MAPK, to avoid excessive response to lipopolysaccharides of bacteria, especially by macrophages [742]. In macrophages, DuSP1 expression is synergistically induced by TLR and IL10 or glucocorticoid receptor signaling [741]. Phosphatase DuSP1 acts primarily on P38MAPK, thereby limiting the activity of CREB and AP1 transcription factors and controlling the expression of a set of LPS target genes. In microglia, endocannabinoid anandamide upregulates DuSP1 via CB2, hence dampening MAPK activation.
DuSP2
Dual-specificity phosphatase-2 (DuSP2) is also named PAC1.85 It is predominantly expressed in hematopoietic cells. It is located in the nucleus. It inactivates ERK1 and ERK2. Phosphatase DuSP2 is a positive regulator of inflammatory response [739].
Phosphatase DuSP2 is highly inducible in macrophages, mastocytes, eosinophils, and B and T lymphocytes by activated receptors. In mastocytes and macrophages, DuSP2 controlc JNK–ERK crosstalk [741]. Expression of DuSP2 is induced in macrophages by TLR ligands and in mastocytes by FcεR1 ligation. Protein DuSP2 associates with MAPKs and blocks JNK-mediated inhibition of ERK activation.
DuSP4 – MKP2
Dual-specificity phosphatase-4 (DuSP4)86 is widespread. It localizes to the nucleus. Two alternatively spliced transcript variants encode distinct isoforms.
Phosphatase DuSP4 interacts with MAPK3 (or ERK1), MAPK8 (or JNK1), and MAPK14 (or P38MAPKα) as well as cytokine suppressive anti-inflammatory drug-binding protein CSBP2 that itself connects to growth arrest and DNA-damage-inducible molecule GADD45α, MAP2K3, and MAP2K6 [251].
DuSP5
Dual-specificity phosphatase-5 (DuSP5)87 is produced in various tissues, but with high level in pancreas and brain. It lodges in the nucleus. It inactivates ERK1 [251].
Phosphatase DuSP5 is encoded by the vascular-specific Dusp1 gene. It is expressed in angioblasts and in the vasculature, where it counteracts Ser/Thr sucrose non-fermenting-related kinase SNRK1. Kinase SNRK1 operates in migration, maintenance, and differentiation of angioblasts and favors endothelial cell migration. It tags arterial vasculature in association with Notch signaling [743], whereas the Rho pathway favors venous differentiation of microvasculature. Enzymes DuSP5 and SNRK1 function together to control angioblast population density [744]. In addition, DuSP5 impedes apoptosis of endothelial cells.
DuSP6 – MKP3
Dual-specificity phosphatase-6 (DuSP6) targets ERK1, ERK2, and P38MAPKα, in addition to MyoD family inhibitor and testis expressed protein-11 (TEx11) [251]. It is also identified as MKP3 and PYST1. The Dusp6 gene is expressed in various tissues with the highest levels in heart and pancreas. Unlike most other members of the group of typical DuSPs, it resides in the cytoplasm. Two transcript variants encode different isoforms.
Phosphatase DuSP6 is negative feedback regulator of ERK2 downstream from FGFR signaling. Fibroblast growth factors are secreted glycoproteins that contribute to morphogenesis by initiating the FGFR–Ras–MAPK cascade. Dual-specificity phosphatase DuSP6, sonic Hedgehog, Sproutys, and FGF-binding protein, the Similar expression to FGFs (Sef), operate in coordination as feedback attenuators of the Ras–MAPK pathway to adjust FGF signaling to optimal level in embryogenesis. Whereas Sef and Sproutys impedes the Ras–MAPK axis at multiple nodes, DuSP6 restrains this signaling by dephosphorylating a single component, ERK.
At least in some animal species, DuSP6 is synthesized in cardiac progenitor cells and attenuates the FGF–ERK signaling by dephosphorylating ERK1/2. Inhibitor of DusP1 and DusP688 reversibly represses these phosphatases that are directly activated by ERK binding. As this inhibitor interferes with ERK-mediated activation, it does not disturb DuSP6 basal activity, but precludes ERK-stimulated DuSP6 enzymatic activity that thus remains at a low level and unresponsive to additional stimulus. Transient DuSP6 inhibition in zebrafish embryos elevates FGF signaling and expands the pool of cardiac progenitor cells at the expense of hematopoietic or endothelial cell lineages [745].
DuSP7 (MKPx)
Dual-specificity phosphatase-7 (DuSP7)89 abounds in leukocytes of patients with acute myeloid or lymphoid leukemia. There is 2 splice variants: DuSP7L and DuSP7S. Isoform DuSP7S may function as an inhibitor of DuSP7L [746].
Enzyme DuSP7 possesses a nuclear export sequence that confines the protein to the cytoplasm [747]. In vivo, DuSP7 binds with high efficiency and dephosphorylates extracellular signal-regulated kinases ERK1 and ERK2.
Protein DuSP7 has a broad tissue distribution (brain, heart, lung, kidney, liver, skeletal muscle, pancreas, and placenta) [747]. Two splice isoforms have been described: DuSP7L and DuSP7S that lacks the catalytic domain.
DuSP8
Dual-specificity phosphatase-8 (DuSP8)90 is expressed predominantly in the adult brain, heart, and skeletal muscle. It abounds in the central and peripheral nervous system. It inactivates mitogen-activated protein kinase [748].
Olfactory stimuli primes expression of several immediate early genes, among which the DUSP8 gene in certain brain regions of the set of odor-processing nervous structures [749].
DuSP9 – MKP4
Dual-specificity phosphatase-9 (DuSP9)91 is highly synthesized in the developing liver and placenta, but at low level in other organs. It thus has a developmentally regulated expression. It is indeed essential for placental development and function [739]. It is also produced in the lung, kidney, and adipose tissue [750].
Phosphatase MKP4 has a high specificity for extracellular signal-regulated kinases ERK1 and ERK2, but a low one for P38MPAKα and Jun N-terminal kinases [750]. It possesses a nuclear export sequence that confines the protein to the cytoplasm.
DuSP10 – MKP5
Dual-specificity phosphatase-10 (DuSP10), or MAPK phosphatase MKP5, interacts with MAPK8, MAPK9, and MAPK10 (JNK1–JNK3) as well as MAPK14 (P38MAPKα) [251].
It functions in innate and adaptive immunity, primarily by regulating the JNK pathway in innate and adaptive immune effector cells [751]. It limits the cytokine production in CD4 + and CD8 + effector T cells. On the other hand, it promotes T-cell proliferation upon activation. In macrophages, TLR ligands induce DuSP10 production to impede JNK activity, thereby constraining the production of cytokines and reducing costimulation of T cells. In T lymphocytes, DuSP10 is constitutively expressed and inhibits early JNK activation after TCR ligation [741].
DuSP14
Phosphatase DuSP14 interacts with T-cell costimulatory factor CD28 [736].92 It can also target ERK and JNK and, via these kinases, interleukin-2. It may then inhibit costimulatory signaling in T lymphocytes.
In pancreatic β cells, DuSP14 production rises in response to glucagon-like peptide GLP1, a growth and differentiation factor for these cells, to launch a negative feedback [737]. Activated dopamine D1 receptor can provoke the synthesis of DuSP14 phosphatase.
DuSP16 – MKP7
Dual-specificity phosphatase-16 (DuSP16), or MKP7, interacts not only with ERK2 (MAPK1), MAPK8 to MAPK10 (JNK1–JNK3), and MAPK14 (P38MAPKα), but also with scaffold proteins MAPK8IP1 and MAPK8IP2 (a.k.a. JNK-interacting proteins JIP1 and JIP2).
DuSP24 – STYXL1
Dual-specificity phosphatase-24, or MAPK phosphatase-like inhibitor Ser/Thr/Tyr (STY)-interacting-like protein STYXL1,93 is a catalytically inactive dual-specificity phosphatase as a result of a naturally occurring replacement of the phosphatase catalytic cysteine by serine.
Among 5 potential isoforms, DuSP24 is the single isoform that encodes both the CDC25-like domain and dual-specificity phosphatase segment. It may antagonize cell survival [752]. It is widespread.
DuSP26 – MKP8
Dual-specificity phosphatase-26 (DuSP26 or DuSP24), or mitogen-activated protein kinase phosphatase MKP8,94 targets P38MAPK and prevents P38MAPK-mediated apoptosis.
8.3.13.4 Slingshot Phosphatases
The slingshot (Ssh) family of phosphatases contains 3 members (Ssh1–Ssh3) encoded by 3 genes (SSH1L–SSH3L). Each Ssh has long and short variants. They have distinct subcellular location [483] (Table 8.36).
They dephosphorylate proteins of the actin-depolymerization factor–cofilin family. Mammalian slingshots can dephosphorylate SerP and ThrP residues. They possess the PTP catalytic domain as well as 14-3-3-binding motifs, a C-terminal filamentous actin-binding site, and a SH3 binding sequence. Phosphorylated (Ser) slingshot phosphatases bind to 14-3-3 proteins for cytoplasmic sequestration (inactivation).
Table 8.36
Slingshot properties (Source: [483]). Isoform Ssh3 is less effective than the others in dephosphorylating phosphoADF and phospho-cofilin.
Ssh Type | Distribution | Localization | Regulators | Substrates |
---|---|---|---|---|
Ssh1 | Ubiquitous | Lamellipodia | 14-3-3 | Cofilin, ADF, LIMK1 |
Ssh2 | Ubiquitous | Lamellipodia | Calcineurin | Cofilin, ADF |
Ssh3 | Ubiquitous | Membrane | PI3K | Cofilin, ADF |
protrusion |
The 3 slingshots are widespread. However, they have distinct subcellular locations, and hence related but distinct functions in the regulation of actin polymerization. Slingshot phosphatases dephosphorylate ADFP and cofilinP to impede actin filament assembly caused LIM domain-containing kinase LIMK1 and testis-specific kinase TesK1. Moreover, slingshot Ssh1L dephosphorylates LIMK1 [736].
Cofilin is a ubiquitous actin-binding molecule required for the reorganization of actin filaments. Cofilin dephosphorylation enables its depolymerizing activity during cell migration. In fact, 2 distinct families of phosphatases dephosphorylate cofilin (Ser3): slingshot phosphatases and haloacid dehalogenase phosphatase chronophin (Sect. 8.3.16.3).
Slingshot phosphatases are regulated by pathways that involve Ca
influx (e.g., Ca
-mobilizing agents ATP and histamine), cAMP and PI3K [483]. Calcium-regulated PP3 dephosphorylates (activates) Ssh1, whereas PAK4 inhibits Ssh1. Insulin-primed actin reorganization occurs via PI3K. Slingshot activity is also directly stimulated by Factin binding.


During healing of wounded skin, keratinocytes migrate. Integrins β4 regulate the laminin matrix assembly through which keratinocytes move. Whereas in neurons, small GTPase Rac1 signals via p21-activated kinase to phosphorylate (inactivate) cofilin, in keratinocytes, α6β4 integrin works via Rac1 to maintain active cofilin via slingshot phosphatases [753].
As they control cofilin activity, slingshot phosphatases are implicated in mitosis, growth cone motility and morphology, and neurite extension, as well as regulation of membrane protrusion [483]. During metaphase of the cell division, they participate in cortical actin regulation; during anaphase and telophase in the cleavage furrow; and during late telophase, in the midbody (a set of bundles of microtubules derived from the mitotic spindle at the end of cytokinesis, just prior to the complete separation of the daughter cells).
8.3.13.5 Protein Tyrosine Phosphatases-4A
Members of the protein Tyr phosphatase-4A family (PTP4a1–PTP4a3) are also called phosphatases of regenerating liver (PRL1–PRL3). They possess the conserved PTP catalytic domain and a C-terminal CAAX box that allows post-translational farnesylation and localization to membranes. They have a specific tissue distribution. Like other PTPs, PTP4as can be inactivated by reversible oxidation.
8.3.13.6 Cell-Division Cycle-14 Phosphatase Homologs
Phosphatases of the CDC14 family specifically dephosphorylate cyclin-dependent kinases. Four CDC14 phosphatases exist: CDC14a and CDC14b as well as kinase-associated phosphatase (KAP), or cyclin-dependent kinase inhibitor CIP3, and protein Tyr phosphatase domain-containing protein-1 (PTPDC1 or PTP9Q22). They are all related to class-3 cysteine-based CDC25 Tyr phosphatases that regulate the initiation of mitosis and DNA-damage checkpoint control.
Phosphatase CIP3 dephosphorylates cyclin-dependent kinase CDK2, hence hindering cell cycle progression. Mammalian CDC14 phosphatases contribute to centrosome maturation, spindle stability, cytokinesis, and cell cycle progression. They are primarily regulated by sequestration in the nucleolus during interphase [736].
8.3.13.7 Myotubularins
Myotubularins (myotubular myopathy-associated gene product [MTM])95 and myotubularin-related proteins (Sect. 2.10.2; Table 8.37) form a family of protein Tyr phosphatases. They use inositol phospholipids rather than phosphoproteins as substrates. These lipid phosphatases dephosphorylate phosphatidylinositol 3-phosphate and phosphatidylinositol (3,5)-bisphosphate. Myotubularin can hydrolyze SerP and TyrP substrates due to the presence of the DSP catalytic motif, but its activity is relatively inefficient.
Table 8.37
Superfamily of myotubularins (myotubular myopathy-associated gene products [MTM] and myotubularin-related phosphatases [MTMR]; SBF: SET binding factor). Both SBF1 and SBF2 are approved symbol for MTMR5 and MTMR13 (3PAP: 3-phosphatase adaptor protein; CNM: X-linked centronuclear myopathy [XLCNM; a.k.a. myotubular myopathy] protein; MTMx: X-linked recessive myotubular myopathy [XLMTM) protein; CMT4b: Charcot-Marie-Tooth disease type-4B protein; CRA: cisplatin resistance-associated protein; EDTP: egg-derived tyrosine phosphatase; FAN: Fanconi-associated nuclease; FYVEDuSP: FYVE domain-containing dual-specificity protein phosphatase; PIP3AP: phosphatidylinositol 3-phosphate 3-phosphatase adaptor; lipSTYX: lipid-selective Ser/Thr/Tyr-interacting (STYX) protein-like protein; ZFYVE: zinc finger FYVE domain-containing protein). The abnormal Mtmr14 gene produces the JUMPY phenotype in flies (a muscle defect and progressive loss of muscle control with shaky and slower motions, hence the name JUMPY).
Type | Other alias(es) |
---|---|
MTM1 | CNM, MTMx |
MTMR1 | |
MTMR2 | CMT4b1 |
MTMR3 | ZFYVE10, FYVEDuSP1 |
MTMR4 | ZFYVE11, FYVEDuSP2 |
MTMR5 | SBF1 |
MTMR6 | |
MTMR7 | |
MTMR8 | |
MTMR9 | MTMR8, lipSTYX, C8ORF9 |
MTMR10 | |
MTMR11 | CRA |
MTMR12 | PIP3AP, 3PAP |
MTMR13 | SBF2, CMT4b2 |
MTMR14 | C3ORF29, EDTP, Jumpy |
MTMR15 | FAN1 |
Myotubularins commonly contain motifs assigned to between-protein or protein–lipid interactions. Most myotubularins indeed possess PH and FYVE (Fab1p, YOTB, Vac1p, and EEA1) domains that link to phosphoinositide lipids and/or PDZ domains that connect to protein. In any case, they have an N-terminal PH–GRAM (pleckstrin homology–glucosyl transferase, Rab-like GTPase activator, and myotubularin domain) region that binds particular lipids and recruits proteins to the plasma membrane and a C-terminal coiled-coil domain. The PTP domain contains a SET-interacting domain (SID sequence) that is conserved in myotubularins [754]. The PH-G domain is a phosphoinositide-binding site for all 3 monophosphoinositides as well as PI(3,5)P2.
Among the 12 identified members of the myotubularin family, a subgroup of catalytically inactive members serve as adaptors for the active members. Nearly half the myotubularins are indeed catalytically inactive ( pseudophosphatase).96
The prototypical family member MTM1 is encoded by a gene on chromosome-X.97 Other members include myotubularin-related proteins MTMR1 to MTMR9 as well as MTMR13.98 Members of the MTM family can heteromerize. Both MTM1 and MTMR2 can interact with MTMR12; MTMR6 and MTMR7 with MTMR9; MTMR2 with MTMR5 and MTMR13 [754]. Hetero-oligomerization enhances enzymatic activity.
Myotubularin-related proteins participate in lipid-mediated signaling. Several myotubularins, such as MTMR1, MTMR6 to MTMR8, and MTMR12, promote cell survival. Lipid PI(5)P actually regulates a PI(3,4,5)P3-specific 5-phosphatase that controls PKB activation. Protein MTMR2 promotes the formation of adherens junctions. Protein MTMR6 binds to and inhibits Ca
-activatedKCa3.1 channels.

Myotubularins and Endocytosis
Myotubularins regulate endocytosis. Endocytic membrane trafficking depends on the synthesis of phosphatidylinositol 3-phosphate and phosphatidylinositol (3,5)-phosphate. Phosphoinositide PI3P resides in sorting endosomes, early and late endosome. Class-3 PI3K corresponds to a single kinase (PI3KC3 with catalytic PI3Kc3 subunit) that is responsible for the synthesis of its specific substrate phosphatidylinositol 3-phosphate.99 In humans, it is encoded by the PIK3C3 gene. Endosomal synthesis of PI3P is initiated with the activation of Rab5 and Rab7 GTPases on early and late endosomes, respectively. Phosphoinositide PI3P governs the endosomal recruitment of membrane-trafficking FYVE- and PX-domain-containing regulators, such as early endosome antigen EEA1 and hepatocyte growth factor-regulated Tyr kinase substrate Hrs. Kinase PIP5K3 (or PIKfyve) generates PI(3,5)P2 from PtdIns3P. The conversion of PI3P into PI(3,5)P2 occurs on multivesicular late endosomes.
Myotubularin substrates PI3P and PI(3,5)P2 are regulators of the endocytic pathway. Myotubularins antagonize PIK3C3 and PIP5K3, as MTM1 and MTMR2 utilize both PI3P and PI(3,5)P2 as substrates. Hydrolysis of PI(3,5)P2 produces PI5P that activates myotubularins. Myotubularins can repress accumulation of substrate lipids at inappropriate compartments. Under certain circumstances, the cell needs to limit PI3P concentration on the secretory pathway to avoid recruitment of endosomal proteins [754].
Myotubularins MTM1 and MTMR2 are associated with early and late endosomes, respectively, although MMT1 is recruited at low level in late endosomes. Enzyme MTM1 lowers the endosomal pool of PI3P, but not its overall cellular mass that resides elsewhere in the cell, presumably at lower concentrations [754]. On the other hand, myotubularins do not extract EEA1 from endosomes.
As myotubularins impede endosome formation, it prevents receptor internalization. MTMR2 translocates to EGFR-containing endosomes after EGF stimulation to block receptor degradation via late endosomes [755].
8.3.13.8 Atypical Dual-Specific Phosphatases
Atypical dual-specificity phosphatases have a low molecular mass, as they lack the N-terminal CDC25 homology-2 domain common to mitogen-activated protein kinase phosphatases. However, some atypical DuSPs dephosphorylate MAPK enzymes (Sect. 8.3.13.3). Sixteen atypical DuSPs have been identified (Table 8.38).100 All DuSPs contain the conserved catalytic domain. Among these atypical dual-specificity phosphatases, some are pseudophosphatases (Table 8.39)
Table 8.38
Atypical dual-specificity phosphatases (Source: [736]; HUGO: Human Genome Organisation; BEDP: branching enzyme-interacting DuSP; Cap: mRNA-capping enzyme; DuPD: DuSP and proisomerase domain-containing protein; EPM; epilepsy, progressive myoclonus; GKAP: glucokinase-associated phosphatase; HCE: human corneal epithelial cell phosphatase; JKAP: JNK pathway-associated phosphatase; JSP: JNK-stimulating phosphatase; LD: Lafora disease protein [or laforin]; LDP: low-molecular-mass DuSP; lmwDSP: low-molecular-weight DuSP; mDSP: muscle-restricted DuSP; MKPL: MKP1-like protein Tyr phosphatase; MOSP: myelin- and oligodendrocyte-specific protein phosphatase; PTPmt: protein Tyr phosphatase localized to the mitochondrion; PIR: phosphatase that interacts with RNA–ribonucleoprotein complex; PLIP: PTen-like phosphoinositide [PI(5)P] phosphatase; RNGTT: RNA guanylatetransferase and 5′-phosphatase; SKRP: stress-activated protein kinase pathway-regulating phosphatase; STYX: Ser/Thr/Tyr-interacting protein; tmDP: testis- and skeletal muscle-specific DuSP; VHR: vaccinia virus open reading frame H1 (VH1)-related; VHX: VH1-related phosphatase-X; VHY: VH1-like member-Y; VHZ: VH1-related phosphatase-Z).
Type | Alias(es) | Subcellular localization |
---|---|---|
DuSP3 | VHR | Nucleolus |
DuSP11 | PIR1 | Nucleus |
DuSP12 | DuSP1, yVH1, GKAP | Cytoplasm, nucleus |
DuSP13a | LDP1, tmDP | |
DuSP13b | BEDP, mDSP, SKRP4, | Cytoplasm |
tsDSP6 | ||
DuSP14 | MKP6, MKPL | Cytoplasm (T lymphocytes) |
DuSP15 | VHY | Cytoplasm (plasma membrane) |
DuSP18 | DuSP20, lmwDSP20 | Cytoplasm, nucleus, mitochondrion |
DuSP19 | DuSP17, SKRP1, LDP2, | Cytoplasm |
lmwDSP1, lmwDSP3, tsDSP1 | ||
DuSP21 | lmwDSP21 | Cytoplasm, nucleus, mitochondrion |
DuSP22 | lmwDSP2, JKAP, JSP1, | Nucleus and cytoplasm |
VHX, tsDSP2, VHX | (enriched in Golgi body) | |
DuSP23 | DuSP25, LDP3, | Cytoplasm |
VHZ | ||
DuSP26 | DuSP24, LDP4, | Cytoplasm (perinuclear region, |
MKP8, NATA1, SKRP3 | Golgi body), nucleus | |
DuSP27 | DuPD1, FMDSP, STYXL2 | Cytoplasm |
DuSP28 | DuSP26, VHP | |
EPM2a | Laforin, LD | Cytoplasm |
PTPmt1 | DuSP23, PLIP, MOSP | Mitochondria |
RNGTT | Cap1a, HCE1 | Nucleus |
STYX |
Table 8.39
Subtrates of atypical dual-specificity phosphatases (Source: [737]; GSK: glycogen synthase kinase; PI(5)P: phosphatidylinositol 5-phosphate). Substrates of atypical DuSPs include not only proteins phosphorylated on Ser, Thr, and Tyr, but also phosphatidylglycerol phosphate (PGP), mRNA capping structure, and glycogen.
Type | Substrates |
---|---|
DuSP11 | RNA 5′triphosphate |
DuSP12 | Glucokinase |
DuSP27 | Pseudophosphatase |
Laforin | Glycogen, GSK3β |
PTPmt1 | PGP, PI(5)P |
RNGTT | 5′Cap (RNA phosphatase) |
STYX | Pseudophosphatase |
Laforin
The unique dual-specificity phosphatase laforin with a carbohydrate-binding domain (CBM20) is encoded by the EPM2A gene.101 The EPM2B gene encodes ubiquitin ligase malin, or NCL1,102 HT2a,103 and Lin41 (NHL) repeat-containing protein-1 (NHLRC1), that ubiquitinates laforin for proteasomal degradation. Laforin phosphatase dephosphorylates glycogen to maintain a normal pool of cellular glycogen.104
In addition to a DuSP catalytic domain, laforin possesses a carbohydrate-binding module-20 (or starch-binding domain) in its N-terminus that is a glycogen-binding site. Laforin binds glycogen-targeting regulatory subunit-5 of protein phosphatase-1 (PP1r5), or protein targeting to glycogen (PTG), that recruits glycogen synthase [736]. Protein phosphatase-1 subunit PP1r5 also impedes the activity of glycogen degradation enzymes phosphorylase and phosphorylase kinase [737]. Laforin and malin form a complex that ubiquitinates PP1r5 for proteasomal degradation, thereby precluding glycogen accumulation, especially in neurons that do not synthesize phosphorylase and phosphorylase kinase involved in glycogen hydrolysis. Laforin may serve as a substrate adaptor that recruits proteins in glycogen particles for ubiquitination by malin and subsequent degradation by the proteasome. In addition, malin ubiquitinates laforin (autoregulation). Interaction between laforin and malin relies on laforin phosphorylation by AMPK. Moreover, AMPK phosphorylates PP1r5 (Ser8 and Ser268) to accelerate its ubiquitination by the laforin–malin complex [737].
STYX
The Ser (S)/Thr (T)/Tyr (Y)-interacting (X) protein (STYX)105 is a pseudophosphatase.106 Like its related pseudophosphatases, MAPK Ser (S)/Thr (T)/Tyr (Y)-interacting protein (MKSTYX or STYXL1), Dusp27 (or STYXL2), and MTMR9 (or lipid-selective STYX-like protein [lipSTYX]), STYX functions as an adaptor for phosphorylated targets that operate in the differentiation of male germ cells [756]. Protein STYX is predominantly produced not only in the testis, but also in the myocardium and skeletal muscle; it is widespread.
Protein STYX interacts with the phosphorylated, 24-kDa, RNA-binding, Ca
-regulated, heat-stable protein CRHSP24. The latter is substrate of PP3 phosphatase [737].

RNA Phosphatase RNGTT
Like DuSP11, RNGTT phosphatase possesses an N-terminal phosphatase domain that contains the P-loop motif, but lacks the catalitically involved Asp residue of the Cys-based PTPs. This RNA triphosphatase removes the γ-phosphate from the 5′ end of nascent mRNA to promote the formation of unmethylated 5′ cap structure, which facilitates transcript translation and subsequent mRNA processing.
Mitochondrial Protein Tyr Phosphatase PTPmt1
Mitochondrial atypical dual-specificity phosphatases include DuSP18, DuSP21, and PTPmt1. Mitochondrial protein Tyr phosphatase PTPmt1 localizes to the mitochondrion inner membrane; more precisely, it is anchored to the matrix face of the inner membrane. It colocalizes with components of the respiratory chain.
Its preferred substrate is PI(5)P in vitro. It dephosphorylates phosphatidylglycerol phosphate, which is similar PI(5)P that exists only in very low concentrations in mitochondria, to phosphatidylglycerol, an intermediate in the cardiolipin synthesis [757].
It is also called myelin- and oligodendrocyte-specific protein (MOSP), as it is detected in oligodendrocytes and myelin of the central nervous system, where it can serve as a late marker.107
In pancreatic β cells, this mitochondrial atypical dual-specificity phosphatase attenuates ATP production and insulin secretion under both basal and glucose-stimulated conditions [737].
DuSP3
Phosphatase DuSP3 preferentially targets diphosphorylated TyrP and SerP–ThrP peptides rather than monophosphorylated compounds and TyrP in monophosphorylated substrates rather than SerP or ThrP. Unlike some other DuSPs, the DUSP3 gene is not an early-response gene.
Ubiquitous DuSP3 intervenes during the cell cycle as well as several signaling pathways involved in the immune response. Regulation of DuSP3 function is achieved by phosphorylation. In T lymphocytes, DuSP3 is phosphorylated by ZAP70 to enable antigen signaling from T-cell receptors.
Kinases ERK1, ERK2, and JNK, as well as EGFR and STAT5 are DuSP3 substrates [736]. Once phosphorylated (Tyr138) by ZAP70 or TyK2 kinase, DuSP3 targets STAT5 especially in cells stimulated by Ifnα and Ifnβ [737].108
When DuSP3 expression is reduced, JNK and ERK kinases are hyperactivated and the cell cycle stops during the G1–S and G2–M transitions. In addition, cells undergo senescence [737].
DuSP11
DuSP12
Dual-specificity phosphatase-12 (DuSP12)109 dephosphorylates glucokinase, thereby enhancing its activity. It also interacts with pleiotrophin, methylCpG-binding domain protein MBD6, and prenylated Rab acceptor RabAC1 [736]. It possesses a N-terminal DUSP domain and a Cys-rich C-terminus that can bind zinc.
Phosphatase DuSP12 acts as a ribosome assembly factor that regulates ribosome stalk assembly, in particular in humans [758]. It interacts with heat shock protein HSP70 using its Zn-binding domain together with the HSP70 ATPase domain to enhance cell survival.
DuSP13
Two isoforms — DuSP13a and DuSP13b — are encoded by alternative open reading frames of the single DUSP13 gene [736]. Isoform DuSP13a is highly expressed in the testis (hence the alias tmDP for testis and skeletal muscle-specific dual-specificity phosphatase); DuSP13b is only produced in the muscle (hence the alias mDSP for muscle-restricted dual-specificity phosphatase). Protein DuSP13 interacts with coiled-coil domain-containing protein CCDC85b [251].110
DuSP15
Phosphatase DuSP15 is highly expressed in testis, but is also synthesized at low levels in other organs, such as the brain, spinal cord, and thyroid [736]. This myristoylated atypical dual-specificity phosphatase is characterized by the addition of myristic acid to Gly residue that enables its recruitment to the plasma membrane [737].
DuSP18
Mitochondrial DuSP18 (or lmwDuSP20) is strongly produced in the brain, liver, testis, and ovary, but is also synthesized in most tissues. In cells, it can be restricted to mitochondria, from which it is released during apoptosis. It may then dephosphorylate JNK, but not ERK and P38MAPK [736].
Unlike PTPmt1 that possesses an N-terminal mitochondrial localization signal, DuSP18 contains an internal mitochondrial localization signal that enables its localization in the mitochondrial inner membrane. Unlike PTPmt1 that is anchored to the matrix face of the inner membrane, it is located at the external edge that faces the intermembrane space [737].
DuSP19
DuSP21
DuSP22
Two DuSP22 variants can be found, a ubiquitous and a testis- and liver-restricted isoform. DuSP22 is required for full JNK1 activation in response to cytokines via MAP2K4 and MAP2K7 [736]. It also interacts with ERK2 [251]. Like DuSP15, DuSP22 is myristoylated to be recruited to the plasma membrane [737].
Protein DuSP22 is also involved in the regulation of certain transcription factors, such as STAT3 and estrogen receptor-α. It indeed dephosphorylates ERα (Ser118) [737]. It also dephosphorylates STAT3, especially during interleukin-6 stimulation.
DuSP23
Phosphatase DuSP23 is detected in the cytosol. It is widespread with high expression in the heart, spleen, liver, and testis [736]. It is produced in different hematopoietic cell lines.
DuSP26
Phosphatase DuSP26, also called DuSP24 and DuSP28,112 It has its highest expression in the brain, heart, adrenal glands, and skeletal muscles [736]. It localizes mainly in the nucleus. Its synthesis rises upon nerve growth factor stimulation.
Substrates of DuSP26 include MAPKs and PKB (Ser473) [737]. Phosphatase DuSP26 impedes cell growth in response to epidermal growth factor, as it lowers EGFR production. In addition, DuSP26 participates in intracellular transport and cell adhesion, since it binds microtubule-associated nanomotor KIF3 that carries β-catenin and N-cadherin to the plasma membrane [737].
DuSP27
Protein DuSP27 is also labeled dual-specificity phosphatase and proisomerase domain-containing DUPD1, but refers to another open reading frame that encodes a pseudophosphatase [737]. It is produced in adipose tissue, skeletal muscles, and liver [736].
Protein DuSP27 can dephosphorylate SerP and ThrP peptides, but is more efficient on TyrP peptides. It processes particularly phosphorylated substrates in which 2 phosphorylated residues are separated by 2 amino acids instead of 1 such as MAPK enzymes.
DuPD1
Atypical dual-specificity phosphatase and proisomerase domain-containing protein-1, in fact, does not contain the proisomerase sequence [737].
8.3.14 Infraclass-2 Protein Tyrosine Phosphatase
Soluble acid phosphatase-1 (AcP1), or low-molecular-weight protein phosphotyrosine phosphatases (lmwPTP; ∼ 20 kDa)113 generated by the highly polymorphic ACP1 gene have a sequence homology with other classes of PTPs, the active site sequence that contains Cys and Arg residues involved in enzyme catalysis found in all PTPs [759].
In both vascular smooth muscle and endothelial cells, low-molecular-weight protein Tyr phosphatases include 2 active isoforms – AcP1IF1 and AcP1IF2 – that operate in cell growth and migration [761].114
Both isoforms AcP1IF1 and AcP1IF2 inhibit PDGF-induced protein synthesis and cell migration in smooth myocytes. On the other hand, both isoforms enhance lysophosphatidic acid-stimulated migration of endothelial cells, without change in DNA synthesis. In addtion, high concentrations of glucose suppress AcP1 production.
Phosphatase AcP1 targets phosphorylated platelet-derived growth factor receptor [760]. It also dephosphorylates insulin receptor. Isoform IF1 is more active than isoform IF2 on the insulin receptor.
8.3.15 Infraclass-3 PTP Cell-Division Cycle-25 Phosphatases
Cell-division cycle dual-specificity phosphatases CDC25 remove inhibitory phosphate on specific Thr and Tyr residues in cyclin-dependent kinases, hence controlling progression through different phases of the cell cycle. Phosphatases of the CDC25 infraclass include 3 isoforms (CDC25a–CDC25c) that are encoded by 3 genes (Cdc25A–Cdc25C).
The CDC25 isozymes have both different and redundant specificities and regulations. Phosphatase CDC25a intervenes at the G1–S transition. Paralog CDC25b is activated during S phase and activates the CDK1–CcnB complex in the cytoplasm. Active CDK1–CcnB complex then phosphorylates (activates) CDC25c, thereby generating a positive feedback for entry into mitosis [762]. Moreover, CDC25 contributes to G2 arrest caused by DNA damage or unreplicated DNA. All isoforms are also targets and regulators of the G2–M checkpoint mechanism that is initiated in response to DNA injury.
The expression and activity of CDC25 phosphatases is finely regulated by multiple processes, such as post-translational modifications, interactions with regulatory partners, control of their intracellular localization, and cell cycle-regulated degradation [763].
The CDC25 phosphatases interact with many substances, such as kinases CDK1, Src, Polo-like kinases PlK1 and PlK3, checkpoint kinase ChK1, P38α MAPK, cell cycle mediators, such as cyclin-B1, peptidyl-isomerase Pin1 that regulates CKI1b expression, and proliferating cell nuclear antigen (PCNA), an auxiliary processivity factor for DNA polymerase-δ, as well as 14-3-3 protein family members tyrosine 3-monooxygenase/tryptophan 5-monooxygenase activation protein YWHAα (or 14-3-3α; encoded by the YWHAA gene), YWHAε (14-3-3ε; encoded by the YWHAE gene), and YWHAη (14-3-3η; encoded by the YWHAH gene), and ubiquitin ligase NEDD4 [251].
Proteolysis of cyclin-B is a major mechanism that inactivates cyclin-dependent kinase CDK1 to promote exit from mitosis. Inhibitory phosphorylation of CDK1 (Thr14 and Tyr15) by Wee1 and PKMYT1 that are removed by CDC25 phosphatases not only repress CDK1 during S- and G2 phases, but also in G1 phase to avoid reversal to M phase. Downregulation of CDK1 activates Wee1 and PKMYT1 kinases and simultaneously inhibits CDC25 phosphatase during the M–G1 transition [764]. Therefore, a reciprocal action of CDK1 exist on its kinases and phosphatases ( feedback loop). Conversely, during the G2–M transition, Wee1 and PKMYT1 are phosphorylated (inactivated) when CDK1 activity rises, as CDK activity stimulates its activator CDC25 and inhibits its inhibitors Wee1 and PKMYT1 kinases.
The CDC25 phosphatases are not only rate-limiting activators of cyclin- dependent kinases, but also targets of the ChK1–ChK2-mediated checkpoint pathway. Each CDC25 isoform exerts specific functions. Isoform CDC25a regulates both G1–S and G2–M transitions. During embryogenesis, it intervenes in cell proliferation after the blastocyst stage. Isoform CDC25b is dispensable in embryogenesis, but indispensable in meiotic progression of oocytes. In addition, CDC25a and CDC25b regulate different stages of mitosis. In fact, CDC25b activates CDK1–cyclin-B at the centrosome during prophase, whereas CDC25a operates for subsequent full activation of the nuclear CDK1–cyclin-B complex [765]. Isoform CDC25c is dispensable for both mitosis and meiosis, although it is highly regulated during these processes.
8.3.15.1 CDC25a Phosphatase
Dual-specificity protein phosphatase CDC25a regulates not only the cell cycle progression, but also mitogen-activated protein kinase pathway. Isoform CDC25a actually receives signals from mitogens that influences its stability, localization, and activity.
Phosphatase CDC25a has a non-redundant activity during the cell cycle. It indeed acts during the G1 phase and G1–S transition, with a peak activity that precedes that of CDC25b in prophase, as it activates the CDK2–cyclin-E and CDK2–cyclin-A complexes, a function that has not been observed with other members [766]. Furthermore, in G2 phase, CDC25a activates both CDK1/2–cyclin-A and CDK1–cyclin-B complexes, whereas CDC25b only activates CDK1–cyclin-B [767].
8.3.15.2 CDC25b Phosphatase
Phosphatase CDC25b intervenes in the control of the activity of the CDK1–CcnB complex at the entry into mitosis and together with Polo-like kinase PLK1 in the regulation of the resumption of cell cycle progression after DNA damage-dependent checkpoint arrest in G2. Activity of PLK1 is necessary for the relocation of CDC25b from the cytoplasm to the nucleus at the G2–M transition [769].
8.3.15.3 CDC25c Phosphatase
Activity of CDC25c is precluded by sequestration by 14-3-3ε and 14-3-3γ, especially to complete S phase and G2 checkpoint [770].
8.3.16 Infraclass Aspartate-Based Protein Tyrosine Phosphatases
8.3.16.1 Eye Absent Phosphatases
Eye absent phosphatases (EyA) regulate organogenesis, especially developing kidney, branchial arches, eye, and ear. They are implicated in morphogenesis of thymus, parathyroid, and thyroid that are derived from the pharyngeal region, i.e., cranial neural crest that yields mesenchymal cells of the pharyngeal region [771].
Four mammalian EYA genes encode proteins that contain a N-terminal transactivation domain and a highly conserved C-terminal Eya domain for protein–protein interactions (Table 8.40). The EYA genes are coexpressed with Pax and Six genes. Four EyA1 transcript variants encode 3 distinct isoforms. The EyA phosphatases can act as transcriptional activator.
Table 8.40
Family of eye absent homologs (EyA; BOR: autosomal dominant branchiootorenal syndrome protein; CMD: dilated cardiomyopathy; DfNA10: autosomal dominant, progressive, sensorineural, non-syndromic deafness [hearing loss]).
Type | Aliases |
---|---|
EyA1 | BOR |
EyA2 | EAb1 |
EyA3 | |
EyA4 | CMD1J, DFNA10 |
8.3.16.2 Haloacid Dehalogenases
Haloacid dehalogenases (HAD) possess a highly conserved catalytic domain and 3 characteristic conserved sequence motifs. They use an unconventional catalytic mechanism whereby a phospho-aspartate intermediate transiently forms following nucleophilic processing on the substrate phosphate group.
Soluble nucleotidases belong to the haloacid dehalogenase superfamily. Nucleotidase catalyzes the hydrolysis of a nucleotide into a nucleoside (e.g., AMP to adenosine and GMP to guanosine).
P-type ATPases constitute a superclass of ubiquitous ATP-driven pumps involved in active transport across membranes of charged substrates. They can be grouped into 5 major families according to substrate specificity: type-1 ATPases are heavy metal pumps that are decomposed into 2 subtypes, the type 1A for K + import and type 1B for transport of cations (Cu + , Ag + , Cu
, Zn
, Cd
, Pb
, and Co
; type-2 ATPases Ca
pumps (types 2A and -2B) as well as Na + –K + – and H + –K + pumps (type 2C); type-3 ATPases H + – and Mg
pumps; type-4 ATPases are phospholipid transporting pumps, and type-5 ubiquitous ATPases that have no allocated substrate specificity.







P-type ATPases have several functions: action potentials in heart and nervous system, secretion and reabsorption of solutes in kidneys, acidification in stomach, nutrient absorption in intestine, relaxation of muscles, and Ca
-dependent signal transduction.

The family of haloacid dehalogenase-like hydrolase domain-containing enzymes includes HDHD1a, HDHD2, and HDHD3 that interact with thyroid receptor-interacting protein TRIP13. Subtype HDHD1A116 that can bind to RNA. It can then function as an RNA-binding factor involved in RNA processing or transport. It can also represent an atypical transcription regulatory factor.
8.3.16.3 Chronophin
Chronophin is a phosphatase of the haloacid dehalogenase superfamily that carries out 2 tasks. Chronophin works with LIMK kinase on actin regulators cofilin and ADF to control cofilinP and ADFP turnover, hence the dynamics of the actin cytoskeleton. Chronophin also acts as a pyridoxal phosphatase, as it removes a phosphate from pyridoxal phosphate (also known as vitamin-B6). Chronophin is an unusual phosphatase, it has a large domain that covers the active site with its magnesium ion to help phosphate removal [772].
Chronophin is widespread. It is particulalrly abundant in brain, heart, skeletal muscle, liver, and kidney. It localizes to actin-rich ruffles and membrane protrusions. Chronophin possesses several interaction motifs to link to PI3K and PLC enzymes.
Cofilin is a key regulator of actin cytoskeletal dynamics, the activity of which is controlled by phosphorylation of a single serine residue (Ser3). Cofilin and related actin depolymerizing factor family proteins are phosphorylated (inactivated). Dephosphorylation re-activates cofilin and ADF. Cofilin phosphorylation is regulated by several phosphatases, such as slingshot (Sect. 8.3.13.4) and chronophin. Elevated chronophin activity is associated with lowered cellular Factin content.
Reorganization of actin at cell leading edge during cell displacement requires the coordinated action of both actin-polymerizing and -depolymerizing (severing) factors. Branched actin filaments are created at the leading edge by the actin nucleator ARP2–ARP3 complex and actin filament crosslinkers filamin-A1 and -A2. Actin depolymerizing proteins, such as members of the cofilin–actin depolymerizing factor family, disassemble Factin in the cell rear to recycle actin monomers to the leading edge. Cofilin-dependent Factin depolymerizing activity then contributes to actin polymerization. Cofilin-depolymerized Factin fragments are preferred substrates for the ARP2–ARP3 complex [483]. Cofilin bears phosphorylation–dephosphorylation cycles by LIMK (Sect. 5.9.3) and TesK kinases (Sect. 5.9.4) that prevent cofilin binding to Factin, hence stabilizing Factin, and phosphatases Ssh and chronophin that stimulate Factin depolymerization and subsequent actin nucleation for directional motility.
During mitosis, chronophin relocalizes from cytoplasm, where it resides in prophase and metaphase to cell poles in anaphase and telophase and to cleavage furrow, actomyosin contractile ring, and midbody in late telophase [483].
References
1.
Ezkurdia I, Del Pozo A, Frankish A, Rodriguez JM, Harrow J, Ashman K, Valencia A, Tress ML (2012) Comparative proteomics reveals a significant bias toward alternative protein isoforms with conserved structure and function. Molecular Biology and Evolution(mbe.oxfordjournals.org/content/early/2012/03/22/molbev.mss100.abstract)
2.
Chavent G (2010) Nonlinear Least Squares for Inverse Problems, Theoretical Foundations and Step-by-Step Guide for Applications. Springer, New York
3.
Bensoussan A (1971) Filtrage optimal des systèmes linéaires [Optimal filtering of linear systems] Dunod, Paris
4.
Bertoglio C, Moireau P, Gerbeau JF (2012) Sequential parameter estimation for fluid-structure problems. Application to hemodynamics. International Journal for Numerical Methods in Biomedical Engineering 28:434–455MathSciNet
5.
Lombardi D, Iollo A, Colin T, Saut O (2009) Inverse problems in tumor growth modelling (communication at CEMRACS summer school)
6.
Lagaert JB (2011) Modélisation de la croissance tumorale [Tumor Growth Modeling], PhD thesis, Bordeaux University
7.
van Meer G, Voelker DR, Feigenson GW (2008) Membrane lipids: where they are and how they behave. Nature Reviews – Molecular Cell Biology 9:112–124
8.
D’Arrigo P, Servi S (2010) Synthesis of lysophospholipids. Molecules 15:1354–1377
9.
Voet D, Voet JG (2011) Signal Transduction (Chap 19). Biochemistry (4th edition), Wiley, Hoboken, New Jersey
10.
Berridge MJ (2009) Module 2: Cell Signalling Pathways. Cell Signalling Biology. Biochemical Journal’s Signal Knowledge Environment Portland Press Ltd., London, UK (www.biochemj.org/csb/002/csb002.pdf)
11.
Delon C, Manifava M, Wood E, Thompson D, Krugmann S, Pyne S, Ktistakis NT (2004) Sphingosine kinase 1 is an intracellular effector of phosphatidic acid. Journal of Biological Chemistry 279:44763–44774
12.
Carricaburu V, Lamia KA, Lo E, Favereaux L, Payrastre B, Cantley LC, Rameh LE (2003) The phosphatidylinositol (PI)-5-phosphate 4-kinase type II enzyme controls insulin signaling by regulating PI-3,4,5-trisphosphate degradation. Proceedings of the National Academy of Sciences of the United States of America 100:9867–9872ADS
13.
Maag D, Maxwell MJ, Hardesty DA, Boucher KL, Choudhari N, Hanno AG, Ma JF, Snowman AS, Pietropaoli JW, Xu R, Storm PB, Saiardi A, Snyder SH, Resnick AC (2011) Inositol polyphosphate multikinase is a physiologic PI3-kinase that activates Akt/PKB. Proceedings of the National Academy of Sciences of the United States of America 108: 1391–1396ADS
14.
Weirich CS, Erzberger JP, Flick JS, Berger JM, Thorner J, Weis K (2006) Activation of the DExD/H-box protein Dbp5 by the nuclear-pore protein Gle1 and its coactivator InsP6 is required for mRNA export. Nature – Cell Biology 8:668–676
15.
Alcázar-Román AR, Tran EJ, Guo S, Wente SR (2006) Inositol hexakisphosphate and Gle1 activate the DEAD-box protein Dbp5 for nuclear mRNA export. Nature – Cell Biology 8: 711–716
16.
Macbeth MR, Schubert HL, VanDemark AP, Lingam AT, Hill CP, Bass BL (2005) Inositol hexakisphosphate is bound in the ADAR2 core and required for RNA editing. Science 309:1534–1539ADS
17.
Huang YH, Grasis JA, Miller AT, Xu R, Soonthornvacharin S, Andreotti AH, Tsoukas CD, Cooke MP, Sauer K (2007) Positive regulation of Itk PH domain function by soluble IP4. Science 316:886–889ADS
18.
Shen X, Xiao H, Ranallo R, Wu WH, Wudagger C (2003) Modulation of ATP-dependent chromatin-remodeling complexes by inositol polyphosphates. Science 299:112–114ADS
19.
Mulugu S, Bai W, Fridy PC, Bastidas RJ, Otto JC, Dollins DE, Haystead TA, Ribeiro AA, York JD (2007) A conserved family of enzymes that phosphorylate inositol hexakisphosphate. Science 316:106–109ADS
20.
Lee YS, Mulugu S, York JD, O’Shea EK (2007) Regulation of a cyclin-CDK-CDK inhibitor complex by inositol pyrophosphates. Science 316:109–112ADS
21.
Saiardi A, Bhandari R, Resnick AC, Snowman AM, Snyder SH (2004) Phosphorylation of proteins by inositol pyrophosphates. Science 306:2101–2105ADS
22.
Chakraborty A, Koldobskiy MA, Sixt KM, Juluri KR, Mustafa AK, Snowman AM, van Rossum DB, Patterson RL, Snyder SH (2008) HSP90 regulates cell survival via inositol hexakisphosphate kinase-2. Proceedings of the National Academy of Sciences of the United States of America 105:1134-1139ADS
23.
Szado T, Vanderheyden V, Parys JB, De Smedt H, Rietdorf K, Kotelevets L, Chastre E, Khan F, Landegren U, Söderberg O, Bootman MD, Roderick HL (2008) Phosphorylation of inositol 1,4,5-trisphosphate receptors by protein kinase B/Akt inhibits Ca2+ release and apoptosis. Proceedings of the National Academy of Sciences of the United States of America 105: 2427–2432ADS
24.
Otto JC, Kelly P, Chiou ST, York JD (2007) Alterations in an inositol phosphate code through synergistic activation of a G protein and inositol phosphate kinases. Proceedings of the National Academy of Sciences of the United States of America 104:15653–15658ADS
25.
Zhang C, Majerus PW, Wilson MP (2012) Regulation of inositol 1,3,4-trisphosphate 5/6-kinase (ITPK1) by reversible lysine acetylation. Proceedings of the National Academy of Sciences of the United States of America 109:2290–2295ADS
26.
Ohnishi T, Ohba H, Seo KC, Im J, Sato Y, Iwayama Y, Furuichi T, Chung SK, Yoshikawa T (2007) Spatial expression patterns and biochemical properties distinguish a second myo-inositol monophosphatase IMPA2 from IMPA1. Journal of Biological Chemistry 282: 637–646
27.
Berggard T, Szczepankiewicz O, Thulin E, Linse S (2002) Myo-inositol monophosphatase is an activated target of calbindin D28k. Journal of Biological Chemistry 277:41954–41959
28.
Irvine RF (2002) Nuclear lipid signaling. Science Signaling 150:re13
29.
Schouten A, Agianian B, Westerman J, Kroon J, Wirtz KWA, Gros P (2002) Structure of apo-phosphatidylinositol transfer protein α provides insight into membrane association. EMBO Journal 21:2117–2121
30.
Woodcock EA, Kistler PM, Ju YK (2009) Phosphoinositide signalling and cardiac arrhythmias. Cardiovascular Research 82:286–295
31.
Jao CY, Roth M, Welti R, Salic A (2009) Metabolic labeling and direct imaging of choline phospholipids in vivo. Proceedings of the National Academy of Sciences of the United States of America 106:15332–15337ADS
32.
Breslow DK, Collins SR, Bodenmiller B, Aebersold R, Simons K, Shevchenko A, Ejsing CS, Weissman JS (2010) Orm family proteins mediate sphingolipid homeostasis. Nature 463:1048–1053ADS
33.
Pavoine C, Pecker F (2009) Sphingomyelinases: their regulation and roles in cardiovascular pathophysiology. Cardiovascular Research 82:175–183
34.
Cogolludo A, Moreno L, Frazziano G, Moral-Sanz J, Menendez C, Castañeda J, González C, Villamor E, Perez-Vizcaino F (2009) Activation of neutral sphingomyelinase is involved in acute hypoxic pulmonary vasoconstriction. Cardiovascular Research 82:296–302
35.
Karliner JS (2009) Sphingosine kinase regulation and cardioprotection. Cardiovascular Research 82:184–192
36.
Means CK, Brown JH (2009) Sphingosine-1-phosphate receptor signalling in the heart. Cardiovascular Research 82:193–200
37.
Gellings Lowe N, Swaney JS, Moreno KM, Sabbadini RA (2009) Sphingosine-1-phosphate and sphingosine kinase are critical for transforming growth factor-beta-stimulated collagen production by cardiac fibroblasts. Cardiovascular Research 82:303–312
38.
Sanchez T, Skoura A, Wu MT, Casserly B, Harrington EO, Hla T (2007) Induction of vascular permeability by the sphingosine 1-phosphate receptor-2 (S1P2R) and its downstream effectors ROCK and PTEN. Arteriosclerosis, Thrombosis, and Vascular Biology 27:1312–1318
39.
Sattler K, Levkau B (2009) Sphingosine-1-phosphate as a mediator of high-density lipoprotein effects in cardiovascular protection. Cardiovascular Research 82:201–211
40.
Igarashi J, Michel T (2009) Sphingosine-1-phosphate and modulation of vascular tone. Cardiovascular Research 82:212-220
41.
Frias MA, James RW, Gerber-Wicht C, Lang U (2009) Native and reconstituted HDL activate Stat3 in ventricular cardiomyocytes via ERK1/2: Role of sphingosine-1-phosphate. Cardiovascular Research 82:313–323
42.
Ke Y, Lei M, Solaro RJ (2008) Regulation of cardiac excitation and contraction by p21 activated kinase-1. Progress in Biophysics and Molecular Biology 98:238–250
43.
Jenkins CM, Cedars A, Gross RW (2009) Eicosanoid signalling pathways in the heart. Cardiovascular Research 82:240–249
44.
Zhao J, O’Donnell VB, Balzar S, St Croix CM, Trudeau JB, Wenzel SE (2011) 15-Lipoxygenase 1 interacts with phosphatidylethanolamine-binding protein to regulate MAPK signaling in human airway epithelial cells. Proceedings of the National Academy of Sciences of the United States of America 108:14246–14251ADS
45.
Jacobs ER, Zeldin DC (2001) The lung HETEs (and EETs) up. American Journal of Physiology – Heart and Circulatory Physiology 280:H1–H10
46.
Watanabe K (2011) Recent reports about enzymes related to the synthesis of prostaglandin (PG) F2 (PGF2α and 9α, 11β-PGF2). Journal of Biochemistry 150:593–596
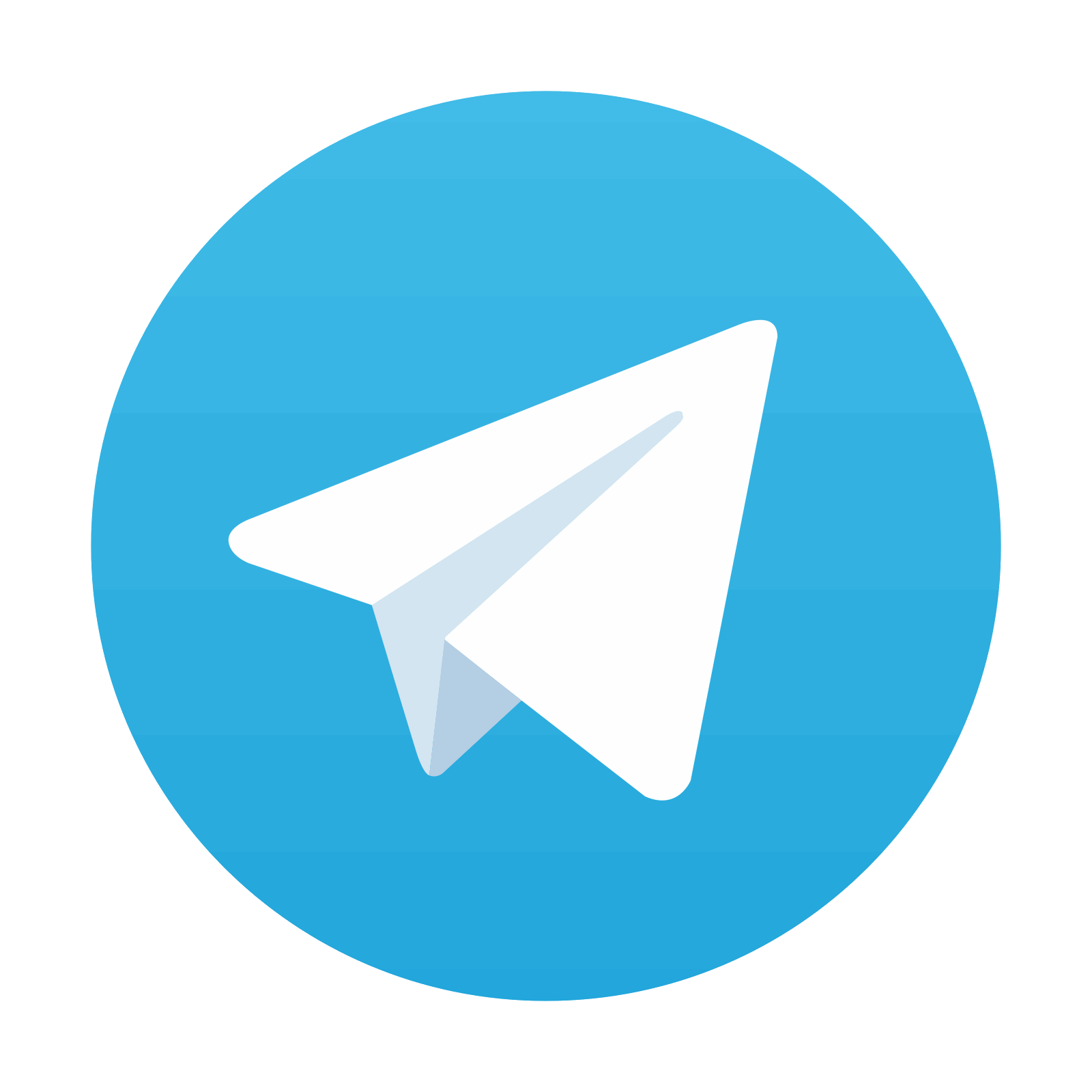
Stay updated, free articles. Join our Telegram channel

Full access? Get Clinical Tree
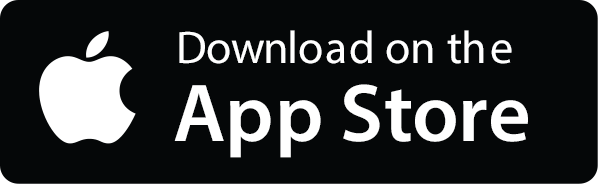
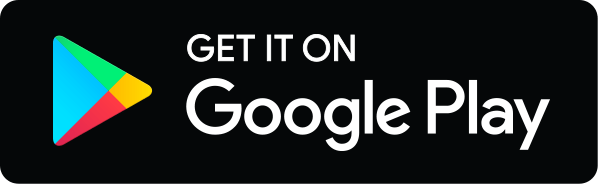
