(1)
Project-team INRIA-UPMC-CNRS REO Laboratoire Jacques-Louis Lions, CNRS UMR 7598, Université Pierre et Marie Curie, Place Jussieu 4, Paris Cedex 05, France
Abstract
Protein tyrosine kinases (PTK), i.e., enzymes that catalyze the phosphorylation of Tyr residues of proteins. are mainly associated with growth factor signaling. They actually modulate multiple cellular events, such as differentiation, growth, metabolism, and apoptosis. On the other hand, protein serine/ threonine kinases are principally related to second messengers, such as cyclic nucleotides cAMP (Sect. 11.1) and cGMP (Sect. 11.2), lipidic and related mediators diacylglycerol and inositol trisphosphate (Chap. 2), and calmodulin (Sect. 11.5.3).
Protein tyrosine kinases (PTK), i.e., enzymes that catalyze the phosphorylation of Tyr residues of proteins. are mainly associated with growth factor signaling. They actually modulate multiple cellular events, such as differentiation, growth, metabolism, and apoptosis. On the other hand, protein serine/ threonine kinases are principally related to second messengers, such as cyclic nucleotides cAMP (Sect. 11.1) and cGMP (Sect. 11.2), lipidic and related mediators diacylglycerol and inositol trisphosphate (Chap. 2), and calmodulin (Sect. 11.5.3).
Protein kinase activity is strongly regulated by phosphorylation, either by the kinase itself (autophosphorylation), or kinase-bound activators or inhibitors. Because enzymes can have different phosphorylation sites, phosphorylation and dephosphorylation either regulate the enzyme activity or directly launch enzyme activation or inhibition.
4.1 Receptor and Cytosolic Protein Tyrosine Kinases
The class of about 100 detected protein Tyr kinases includes 2 main subclasses (Tables 4.1 and 4.2): (1) transmembrane (plasmalemmal) receptor tyrosine kinases (RTK; Vol. 3 – Chap. 8. Receptor Kinases) that can be grouped into 19 families and (2) intracellular non-receptor tyrosine kinases (NRTK) that can be subdivided into 10 families.
Table 4.1
Human protein tyrosine kinases. (Part 1) Receptor and cytoplasmic (non-receptor) families (BMX: bone marrow Tyr kinase gene in chromosome-X product; BRK: breast tumor kinase; BTK: Bruton Tyr kinase; CSK: C-terminal Src Tyr kinase; InsRR: insulin receptor-related receptor; ITK: interleukin-2-inducible T-cell kinase; LTK: leukocyte Tyr kinase; MATK: megakaryocyte-associated Tyr kinase; HGFR: hepatocyte growth factor receptor [a.k.a. mesenchymal–epithelial transition factor (MET)]; MST1R: macrophage stimulating-1 factor receptor; Smrs: src-related kinase lacking C-terminal regulatory Tyr and N-terminal myristylation; TEC: family of NRTKs in T and B lymphocytes and hepatocytes; TNK: Tyr kinase inhitor of nuclear factor-κB).
Receptor families | Non-receptor families |
---|---|
Apoptosis-associated Tyr kinases | Abelson kinases |
(Aatyk1–Aatyk3) | (Abl1–Abl2) |
Anaplasic lymphoma kinase | Activated CDC42-associated kinases |
(ALK, LTK) | (ACK1–ACK2 or TNK2–TNK1) |
Adhesion-related kinases | SRC Tyr kinases |
(Axl, Eyk, Mer, Tyro3, Rek) | (CSK, MATK) |
Discoidin domain kinases | Focal adhesion kinases |
(DDR1–DDR2) | (FAK1, FAK2) |
EGF receptors | Feline sarcoma oncogene kinases |
(HER1–HER4) | (FeR, FeS) |
EPH receptors | Fyn-related kinases |
(EPHa1–a10; EPHb1–b6; EPHx) | (BRK, FRK, Smrs) |
FGF receptors | Janus kinases |
(FGFR1–FGFR4) | (JaK1–JaK3, TyK2) |
Insulin and IGF receptors | SrcA (FGR, Fyn, Src, Yes1) |
(InsR, InsRR, IGF1R) | SrcB (BLK, HCK, LCK, Lyn) |
MET | Spleen Tyr kinases |
(HGFR, MST1R) | (SYK, ZAP70) |
Muscle-specific kinase | TEC kinases |
(MUSK) | (BMX, BTK, ITK, TEC) |
Table 4.2
Human protein tyrosine kinases. (Part 2) Receptors (TEK: Tyr endothelial kinase; TIE: Tyr kinase with Ig and EGF homology receptor (angiopoietin receptor); TRK: neurotrophin receptor).
Receptor PTK families (Cont.) | Members |
---|---|
PDGF receptors | PDGFRα–PDGFRβ |
CSF1R, FLT3, SCFR | |
Protein Tyr kinase-7 | PTK7 |
Rearranged during transfection | ReT |
Receptor Tyr kinase-like orphan receptor | ROR1–ROR2 |
Ros UR2 sarcoma virus oncogene homolog-1 | Ros1 |
Related to receptor Tyr kinase | RYK |
Angiopoietin receptors | TEK, TIE |
Tropomyosin receptor kinase (TRK) | NTRK1–NTRK3 |
VEGF Receptors | VEGFR1–VEGFR3 |
Receptor protein Tyr kinases contain an extracellular ligand-binding domain, transmembrane region, and intracellular cytoplasmic kinase domain. The RTK subclass with 58 members is constituted by growth factor receptors (e.g., epidermal [EGFR], fibroblast [FGFR], insulin-like [IGFR], platelet-derived [PDGF] growth factor receptor). Non-receptor protein Tyr kinases recruited in signaling pathways following ligand binding to receptor comprise 32 known kinases that include Janus, SRC, and TEC kinase families (Tables 4.3 and 4.4).
Table 4.3
Partners and substrates of cytoplasmic protein Tyr kinases (Part 1; Sources: multiple).
Type | Expression | Partners and substrates |
---|---|---|
pattern | ||
Abl1/2 | Ubiquitous | NTRK1, EPHb2, BCR, TOR, ATMK, PAK2, |
GPX1, Rad9a, Rad51, RB, BrCa1, TeRF1, | ||
NCK1, DOK1, PAG1, BCAR1, CASL, CBL, | ||
CRKL, SHC, GRB2/10, PSTPIP1, ArgBP2, | ||
Vav1, RFX1, HDM2, | ||
P73, spectrin-α1 | ||
ACK1/2 | Ubiquitous | EGFR, Src, Fyn, CDC42 |
ALX, GRB2, NCK, DblGEF, RasGRF1 | ||
BrK | Epithelial cell | EGFR, PI3K, PTen, PKB |
FRK | CRK2, KhdRBS1, STAP2, SHB, paxillin | |
BTK | B and mast cells | TNFSF6, PI(4)P5K, PLCγ2 |
PI(3,4,5)P3 | ||
CSK | Hematopoietic | InsR, IGF1R, IRS1 |
cell | FAK, PKA, G protein, PTP, paxillin | |
CBP, DOK, G3BP, SIT, caveolin-1 | ||
FAK1/2 | Ubiquitous | Src, PI3K |
GRB2, SHC, BCAR1, PtdIns, paxillin | ||
FeR, | Granulocyte | EGFR |
FeS | Macrophage | Dynamin-1–3, catenin, cortactin, |
E-cadherin, TMF1 |
Table 4.4
Partners and substrates of cytoplasmic protein Tyr kinases (Part 2; Sources: multiple; HSP: heparan sulfate proteoglycan [a.k.a. CD44 and phagocytic glycoprotein PGP1]).
Type | Expression | Partners and substrates |
---|---|---|
pattern | ||
JaK1–3, | Ubiquitous | Cytokine receptors |
TyK2 | STATs | |
CIS, SOCS, SSI | ||
Src | Ubiquitous | GluN2a, HSP, EPHb2, β3AR, EGFR, |
PDGFR, CSF1R, AR | ||
ERα and -β, NR1b1, HNF1a, | ||
cRaf, PKCζ, PLD2, FAK2, | ||
ARNT, AHR, dystroglycan, KhdRBS1, | ||
PDE6γ, STAT1/3, SRE, DDEF1, NCoA6 | ||
GRB2, Dab2, EPS8, BCAR1, SHB, WASP, | ||
RICS, P120RasGAP, GNB2L1, ND2, | ||
Mucin-1 | ||
SYK, | Hematopoietic | EpoR, CSF3R, Fc |
ZAP70 | cells | BTK, FGR, Fyn, LCK, Lyn, Src, FAK1/2, |
PKCα, PRKD1, PTP6, PLCγ1/2 | ||
Cortactin, paxillin, and tubulin | ||
Vav, GRB2, SHC, CRKL, SLAP, TRAF6, | ||
BCAP, BAnk, BLnk, LCP2, | ||
LAB, LAT, LAX | ||
TEC | T lymphocyte | LCK, PI3K, PLCγ1 |
B lymphocyte (BTK) | EEA1 |
Src homology SH2 domains of intracellular protein Tyr kinases can keep these enzymes in an auto-inhibited conformation. However, SH2 domain of intracellular protein Tyr kinases can enhance substrate recognition and catalytic activity of FeS and Abl kinases that lack this between-domain auto-inhibitory interaction [180]. SH2 domain is involved in substrate recruitment and couples substrate recognition to kinase activation. Moreover, SH2 domain interaction with the kinase domain stabilizes the active kinase conformation.
4.2 Family of Abl Kinases
Viral Abelson murine leukemia oncogene homolog is expressed with 2 paralogs — Abl1 and Abl2 —, which represent the mammalian members of the Abelson family of non-receptor protein Tyr kinases. Kinases Abl1 and Abl2 (or Arg) have redundant functions. Ubiquitous Abl1 and Abl2 can heterodimerize.
Several types of post-translational modifications enable the control of Abl catalysis, subcellular localization, and stability. Binding partners yield additional regulation of Abl activity, substrate specificity, and signaling.
Each Abl subtype contains an SH3–SH2–TK (SH: Src homology; TK: Tyr kinase) domain cassette that confers autoregulated kinase activity. This cassette is coupled to an actin-binding and -bundling domain that couples phosphoregulation to actin filament reorganization.
Kinase Abl11 is a cytosolic and nuclear protein Tyr kinase that contributes to cell differentiation, division, and adhesion, as well as stress response. Protein Abl2 is detected exclusively in the cytoplasm.
Kinase Abl1 possesses nuclear localization signals and a DNA-binding domain used to mediate DNA-damage repair. On the other hand, Abl2 has additional binding motifs for actin and microtubules that enable cytoskeletal remodeling.
4.2.1 Abl1 Kinase (Abl)
Kinase Abl1 (a.k.a. JTK7 and P150) possesses multiple domains, such as SH1 (Tyr kinase), SH2, and SH3 domains, as well as a large C-terminus that contains proline-rich sequences, DNA- and actin-binding motifs, and nuclear localization and export signals.
Messenger RNA ABL1 can be alternatively spliced at the level of the N-terminus to generate Abl1a and Abl1b isoforms [181]. Kinase Abl1 operates in the regulation of cytoskeletal dynamics and cell proliferation and survival. Altered Abl1 exhibits constitutive Tyr kinase activity and provokes leukemias in humans.
In adult humans, the highest Abl1 expression levels are observed in cartilage, adipocytes, and ciliated epithelium. In human fetuses, its highest concentrations are detected in muscle, osteoblasts, endothelial cells, and neovasculature at sites of endochondral ossification in the umbilical cord stroma [181].
Kinase Abl1 regulates pro- or anti-apototic response according to cell type. Activated by DNA damage or oxidative stress, it accumulates in the nucleus to prime a pro-apoptotic response in fibroblasts [181]. It can also be activated in DNA repair that depends on DNA-dependent protein kinase (DNA-PK; Sect. 5.5.2) and ataxia telangiectasia mutated kinase (ATMK; Sect. 5.5.1) as well as G1–S checkpoint.
Enzyme Abl1 undergoes phosphorylation by CDK1. It associates with retinoblastoma protein. It phosphorylates RNA polymerase-2. It can cause cell cycle arrest in response to cell stress. It then activates the stress-activated protein kinase pathway.
In T lymphocytes, upon TCR activation, Abl1 phosphorylates LAT2 adaptor and ZAP703 kinase [181]. Kinase Abl1 intervenes in proliferation of stimulated splenic B lymphocytes as well as that of fibroblasts in response to platelet-derived growth factor. It interacts with B-cell coreceptor CD19 on follicular dendritic cells and B lymphocytes.
Kinase Abl1 is activated by stimulated receptors of growth factors (PDGFR and EGFR), ephrins (EPHb2), and semaphorin-6D, as well as RPTK MuSK (Vol. 3 – Chap. 8. Receptor Kinases) and integrin engagement to reorganize the cytoskeleton [181]. It is also activated by DNA damage and oxidative stress. On the other hand, in addition to auto-inhibition due to intramolecular interactions and folding, Abl1 is inhibited by phosphatidylinositol (4,5)-bisphosphate as well as dephosphorylation by PTPn12 (Sect. 8.3.11.11). Moreover, it is degraded by ubiquitin-dependent proteasome.
Protein Abl1 can heterodimerize with Abl2. It also interacts with adaptor CRK, docking proteins DOK1 and DOK2, Abl interactors AbI1 and AbI2, CDK5 and Abl enzyme substrate CABLES1, Ras and Rab interactor RIn1, proline/serine/threonine phosphatase-interacting protein PSTPIP1, phospholipase-Cγ, anti-oxidant enzyme peroxiredoxin-1, Gactin, and WAVe1 [181].
Kinase Abl1 stimulates the activity of the RNA-binding protein heterogeneous nuclear ribonucleoprotein hnRNPe2 that prevents the translation of the mRNA of the transcription factorC/EBPα, which is involved in leukocyte differentiation. MicroRNA-328 is a double-duty molecule that acts as both a RNA silencer to prevent protein synthesis and competitive inhibitor of translation-inhibiting hnRNPe2 to promote protein synthesis [182].4
4.2.2 Abl2 Kinase (Arg)
Abelson-related kinase Abl2 is another cytoplasmic kinase that shares structural and sequence homology with Abl1. Kinase Abl2 contains Tyr kinase domains (SH1–SH3) and a C-terminus with proline-rich sequences and binding domains for Factin and tubulin [181].
Kinase Abl2 contributes to linkage between plasmalemmal receptors (T-cell receptors, MuSK, EPHb2, and platelet-derived growth factor receptor, as well as integrins) and cytoskeleton and/or cell proliferation signaling. It can be identified in actin cytoskeletal structures and focal adhesions, at least, under certain conditions. In fact, Abl2 is not detected in stress fibers. It is specifically required for integrin-mediated adhesion to laminin-1 and semaphorin7A. Abl Kinases target adaptor P130CAS (or BCAR1) and structurally related docking protein CASL5 that both interact with FAK1 in focal adhesions. Adaptor CRK associates with both Abl1 and Abl2 kinases. Other potential Abl partners that are components of the actin cytoskeleton and focal adhesions include FAK, paxillin, vinculin, talin, and tensin. In addition, target SH3 domain-binding protein SH3BP1 has GTPase-activating protein activity for Rac GTPases involved in the regulation of the actin cytoskeleton [183].
Kinase Abl2 is activated by autophosphorylation as well as phosphorylation by Abl1 and Src kinases [181]. Association of Abl2 with Ras effector Rin1 can also stimulate Abl2. Kinase Abl2 interacts with adaptors CRK and Arg (Abl2)-interacting protein ArgBP2,6 pro-apoptotic protein Siva-1, and ubiquitin for degradation.
4.2.3 Abl-Binding Proteins
Binding partners of Abl1 and Abl2 kinases such as ArgBP27 are substrates of both enzymes. Protein ArgBP2 is widespread in human organs and extremely abundant in the heart. In epithelial cells, ArgBP2 and Abl are located near stress fibers and in the nucleus. Protein ArgBP2 then links Abl kinases to the actin cytoskeleton. This adaptor assembles signaling complexes in stress fibers. In cardiomyocytes, ArgBP2 is located in Z discs of sarcomeres, where it can be targeted by signaling mediators [183].
4.3 ACK Kinases
Activated CDC42-associated kinases ACK1 and ACK28 are intracellular protein Tyr kinases. Small GTPase CDC42GTP binds to the CDC42–Rac interactive-binding domain (CRIB) of ACK in a GTP-dependent manner. Kinase ACK indeed possesses a Tyr kinase core, an SH3 domain, a CDC42-binding region, a RALT homology region,9 and a proline-rich region.
Ubiquitous ACK is highly synthesized in the brain, thymus, and spleen [184]. Activation of integrins as well as EGFR and PDGFR receptors leads to ACK recruitment and its Tyr phosphorylation (activation).
The ACK1 splice variant is activated from chondroitin sulfate proteoglycan. It can regulate cell motility. Isoform ACK2 can regulate cell spreading, motility, and integrin-mediated adhesion [185]. Kinase ACK2 represses focal adhesion complex organization.
Both ACK1 and ACK2 can associate with clathrin to regulate receptor-mediated endocytosis [185]. Epidermal growth factor activates ACK1 that, once phosphorylated, activates RhoGEF21 agent. Activated RhoGEF21 subsequently stimulates Rho GTPases. It contributes to cytoskeletal rearrangements. Kinase ACK2 supports phosphorylation of sorting nexin SNx9 (or SH3PX1) by epidermal growth factor receptor, thereby promoting EGFR degradation.
Phosphorylated ACK1 binds to GRB2 and NCK adaptors. In addition, ACK1 phosphorylates RasGRF1 (a GEF) that targets hRash, kRas, and nRas. Isoform ACK2 binds to Src kinase and ALX (adaptor in lymphocytes of unknown function [X]) adaptor.10
4.4 BrK Kinase (Protein Tyr Kinase-6; FRK Subfamily)
Protein Tyr kinase-6 (PTK6), or breast tumor kinase (BrK), contains an SH2, an SH3, and a catalytic domain. A splice variant comprises a SH3 domain and a short C-terminus.
In mammary epithelial cells, PTK6 enhances mitogenic responses to epidermal growth factor [186]. Expression of PTK6 in breast carcinoma cells causes cell proliferation owing to an elevated EGF-dependent phosphorylation of EGFR-related receptor HER3 and potentiated recruitment of phosphoinositide 3-kinase to HER3 receptor. Protein PTK6 phosphorylates KH domain-containing, RNA-binding signal transduction-associated protein KhdRBS1 (or Sam68) and related proteins to prevent its binding to RNA, thereby impeding the ability of KhdRBS1 to increase expression from Rev-responsive element-containing genes.
Kinase PTK6 regulates protein kinase-B. It also phosphorylates paxillin and promotes Rac1 activation via CRK2, hence assisting in chemotaxis. Protein PTK6 can form complexes with EGFR (HER1), HER3, signal-transducing adaptor protein STAP2, and paxillin, in addition to KhdRBS1 and PKB [186]. Its binding partners also include insulin receptor substrate IRS4 in resting and insulin-like growth factor-1-stimulated cells [187].
4.5 CSK Kinase
Carboxy-terminal Src Tyr kinase (CSK) phosphorylates a regulatory Tyr residue at the C-terminus of kinases of the SRC family, thereby inactivating Src kinases. Kinase CSK is especially produced in hematopoietic cells [188]. It blocks signaling downstream from T-cell receptors mediated by SRC family Tyr kinases.
Protein kinase-A phosphorylates (Ser364) CSK, thereby heightening its kinase activity (2–4-fold) [188]. Activity of CSK is also moderately enhanced (2-fold) by Gβγ subunit. Kinase CSK also forms a complex with the RasGAP-binding protein GTPase-activating protein (SH3 domain)-binding protein G3BP. In addition, CSK interacts with receptors of insulin and insulin-like growth factor-1, insulin receptor substrate-1, focal adhesion kinase, protein Tyr phosphatase, paxillin, palmitoylated transmembrane adaptor phosphoprotein associated with glycosphingolipid microdomain PAG1, or CSK-binding protein (CBP) in membrane rafts, caveolin-1, leukocyte-specific protein Tyr kinase-interacting molecule (LIME), SHP2 (PTPn11)-interacting transmembrane adaptor (SIT), and members of the Downstream of Tyr kinase docking protein (DOK) family.
The DOK proteins participate in the regulation of signaling mediated by receptor and non-receptor kinases. Protein DOK1 precludes cell proliferation initiated by growth factors, as it tethers different signaling components to the cell membrane. It attenuates platelet-derived growth factor action by recruiting CSK to active Src kinases. Moreover, DOK1 impedes PDGF-induced activation of the Ras–MAPK cascade by acting on RasGAP and other DOK1-interacting proteins [189]. Adaptor DOK3 rapidly bears Tyr phosphorylation in response to immunoreceptor stimulation and subsequently recruits inhibitors SHIP phosphatase and CSK kinase [190].
4.6 Focal Adhesion Kinases
Focal adhesion kinases —- FAK1,11 and FAK2 —12 are encoded by the Fak1 and PTK2B genes. Four transcript variants encode 4 isoforms. These cytosolic protein Tyr kinases localize to focal adhesions that create attachments with the extracellular matrix or with other cells. Cells synthesize FAK-related non-kinase (FRNK) from an independent transcript that corresponds to the FAK C-terminus (and hence lacks the kinase activity and autophosphorylation site) to prevent FAK activation.
The primary FAK function is the transmission of signals emitted by integrins. Kinase FAK also participates in growth factor receptor-mediated signaling. It transduces signals to regulate cell survival, proliferation, adhesion, migration, and death. Its activity is modulated by phosphorylation and dephosphorylation. Kinase FAK is phosphorylated in response to integrin engagement, growth factor stimulation, and mitogenic neuropeptides.
Focal adhesion kinase is a scaffold and Tyr kinase that binds to itself and cellular partners through its four-point-one, ezrin, radixin, moesin (FERM) domain.13 Regulators convert auto-inhibited autocatalytic Tyr kinase FAK into its active state by binding to its FERM domain.14 Interactors of FAK support this protein in its coordination of diverse cellular functions in which it is involved.
Partners of FAK that bind to the FERM domain comprise PI(4,5)P2 generated by phosphatidylinositol 4-phosphate 5-kinase PI(4)P5K1γ,15 epidermal (EGFR), hepatocyte (HGFR), platelet-derived (PDGF), and vascular endothelial (VEGF) growth factor receptors, protein Tyr phosphatase PTPn21,16 ezrin, BMX kinase,17 actin-related protein ARP3, receptor for activated kinase RACK1, insulin receptor substrate IRS1, and MAPK8-interacting protein MAPK8IP3,18 in addition to β subunits of integrins [191].
Neural Wiskott-Aldrich syndrome protein, an effector of CDC42 GTPase, is a substrate of FAK enzyme. Together with ARP3, RACK1, and phosphodiesterase PDE4d5, FAK contributes to the assembly of nascent integrin-based adhesions.
Merlin, related to the ERM (ezrin, radixin, and moesin) proteins, is phosphorylated (inactivated) by P21-activated kinase activated upon integrin engagement. Conversely, cadherin engagement inactivates PAK kinase. Merlin can accumulate in the nucleus, where it binds to and inhibits the ubiquitin ligase CRL4–DCAF1 complex (CRL4DCAF1),19 hence the expression of numerous genes [192].
Phosphorylation of specific residues of FAK regulate distinct cellular processes. Phosphorylation (Tyr861 and Tyr925) regulates cell migration of endothelial cells and proliferation, respectively [193] (Table 4.5). Focal adhesion kinase is activated by multiple pro-angiogenic growth factors, such as VEGF, EGF, and FGF2. Enzyme FAK integrates integrin and growth factor signaling to promote cell motility. Kinase FAK not only operates as a kinase, but also as a scaffold, as it yields TyrP-binding sites for signaling mediators.
Table 4.5
Structural features and binding sites of focal adhesion kinases and partners (Sources: [191, 194]; FAT: focal adhesion-targeting domain; FERM: band 4.1 [four], ezrin, radixin, and moesin homology N-terminal domain; Pro: proline-rich Src-homology 3 (SH3) binding motif; TK: tyrosine kinase domain). Focal adhesion kinase associates with adaptors, structural proteins, kinases and phosphatases, as well as small GTPase regulators. Src-mediated phosphorylation of FAK (Tyr925) creates a binding site for GRB2 adaptor to prime the extracellular signal-regulated (ERK) pathway. The FERM domain of FAK, in particular, binds to cytoskeleton- and membrane-linked proteins and phospholipids, mainly at adhesion sites, where it modulates cortical actin and nascent adhesions by binding to the ARP2–ARP3 complex and the molecular scaffold RACK1, in addition to optimal signaling from growth factor receptors (EGFR, HGFR, and PDGFR), but also in the nucleus, where it connects to P53 and its regulator DM2.
Domain | Partners (pathways) |
---|---|
N-terminus | |
FERM | FAK, EGFR, HGFR, PDGFR, VEGFR, BMX, PI3K, |
ezrin, β-integrin, ARP3, RACK1, IRS1, | |
MAPK8IP3, PI(4,5)P2, P53, DM2 | |
Pro1 | GRB7, PLCγ |
Central domain | |
TK | Src, PTen, PTPn12, SHC |
(Tyr397 autophosphorylation site) | |
(FAK–Src–CAS complex–JNK) | |
C-terminus | |
Pro2 | CAS |
Pro3 | ASAP1 (ArfGAP), RhoGAP26, GRB2 |
FAT | paxillin, talin, |
(indirectly integrin, APAP1, Rho(Arh)GEF6/7) |
Focal adhesion kinases mediate several integrin signaling pathways. They can perform autophosphorylation on a Tyr residue. Autophosphorylation of FAK creates a binding site for kinases of the SRC family. This complex allows Tyr phosphorylation of focal adhesion-associated proteins. The FAK kinases interact with Src and PI3K kinases, GRB2 adaptor, integrin signaling mediator CRK-associated substrate CAS (or BCAR1), and paxillin.
Hence, activated FAK controls cell adhesion and motility [195]. Tyrosine-phosphorylated FAK promotes interactions with certain proteins, which allows connections to the Rac and Rho GTPases and extracellular signal-regulated kinase isoform ERK2 cascade. It acts on the assembly and maturation of focal contacts. Activity of the FAK–Src axis promotes phosphorylation of phosphatidylinositols. Kinase Src causes disassembly of focal contacts, as it activates calpain and extracellular matrix metalloproteinases; it also regulates cadherin-mediated cellular junctions. Signaaling vi FAK to Rho GTPases regulates changes in actin and microtubules in cell protrusions of migrating cells.
Mechanical signals from the extracellular matrix sent to fibroblasts are sensed by integrin-based cell–matrix adhesions and transmitted by several types of molecules, among which focal adhesion kinases. The latter actually contributes to the activation of cardiac fibroblasts subjected to mechanical stress [196]. Mechanical stimuli provoke proliferation of cardiac fibroblasts and their differentiation into myofibroblasts and raise the production of matrix components, such as MMP2 and collagen. Enzyme FAK works via target of rapamycin.
Focal adhesion kinase FAK2 is involved in Ca + + -induced regulation of ion channels and activation of the MAPK signaling. Four transcript variants generate 2 different isoforms. It serves as a signaling mediator between neurotransmitters and neuropeptide-activated receptors that cause calcium influx on the one hand and signals that regulate neuronal activity on the other. In response to increased intracellular calcium concentration, nicotinic acetylcholine receptor activation, membrane depolarization, or protein kinase-C activation, FAK2 undergoes rapid Tyr phosphorylation (activation).
In vascular smooth muscle cells, focal adhesion kinase and its inhibitor FAK-related non-kinase (FRNK) regulate cell spreading and migration during vasculo- and angiogenesis as well as vascular remodeling [193].20 Phosphorylation of FRNK on Tyr residues leads to inhibition of VSMC spreading and migration.
In cardiomyocytes, hypoxia not only activates SRC family kinases Src and Fyn and the Ras–MAPK pathway to target stress-activated protein kinase and P38MAPK, but also focal adhesion kinase and paxillin [197]. Hypoxia-induced FAK activation leads to association with adaptors SHC and GRB2 and cytoplasmic Tyr kinase Src. Furthermore, hypoxia causes subcellular translocation of FAK from perinuclear sites to focal adhesions.
4.7 FeS and FeR Kinases (FPS/FES Subfamily)
The mammalian FPS/FES (fujinami poultry sarcoma/feline sarcoma)21 gene encodes protein Tyr kinase FeS that is specifically expressed during hematopoiesis, principally in cells of the granulocyte-macrophage lineage. Another Tyr kinase, antigenically related to FeS, is encoded by FER gene (FeS-related gene). Hence, FeR is considered as a member of the FPS/FES family of non-receptor protein Tyr kinases [198].22
Cytoplasmic FeS and FeR kinases have an N-terminal FCH domain23 for possible microfilament association, 3 regions that potentially regulate oligomerization, a central Src homology-2 domain for binding to phosphorylated (Tyr P -containing) proteins, and a C-terminal catalytic domain [199]. The FCH domain is implicated in the regulation of cytoskeletal rearrangement and vesicular transport. It can bind to microtubules and its SH3 domain can link to actin-reorganizing Wiskott-Aldrich syndrome proteins.24
Kinases FeS and FeR are dispensable for hematopoiesis, as they have redundant activities with other kinases. They are mainly involved in the regulation of inflammation, particularly mastocyte migration and leukocyte diapedesis, and innate immunity. They are actually involved in between-cell and cell–matrix interactions, possibly via rearrangement of the cytoskeleton and crosstalks between integrins of focal adhesions, cell adhesion molecules of adherens junctions, and receptors for growth factors and cytokines [199].
Kinases FeS and FeR participate in signaling downstream from receptors for cytokines (e.g., gmCSF [CSF2], EpoR, IL3R–IL6R, and IL11R), growth factors (e.g., PDGF), and immunoglobin-G. Kinases FeS and FeR can also be activated downstream from IgE receptors on mastocytes and glycoprotein GP6 collagen receptor on platelets [199]. They control cytoskeletal rearrangements and signaling that accompany receptor–ligand, cell–matrix, and cell–cell interactions. They participate in the regulation of inflammation and innate immunity. Kinase FeS indeed abounds in macrophages and neutrophils.
Cell migration requires cycles of actin polymerization and depolymerization. These cycles rely on coordinated interactions between the plasma membrane and actin regulators. Cortactin is an activator of actin polymerization mediated by the actin-related protein ARP2–ARP3 complex. Cortactin thus contributes to cytoskeletal reorganization during cell migration and vesicular transport. Cortactin localizes in lamellipodia and endosomes at the leading edge of moving cells. Tyrosine phosphorylation of cortactin inhibits its actin crosslinking activity and promotes its proteolytic degradation by calpain. Tyrosine phosphorylation of cortactin after engagement of PDGF and FcεR1 receptors is caused, at least partly, by FeR kinase.
Phosphatidic acid can be produced by phospholipase-D for cell proliferation, survival, and migration. Phosphatidic acid binds to the Tyr kinase FeR and enhances its ability to phosphorylate cortactin [200]. Kinase FeR favors lamellipodium formation and cell migration. This effect depends on PLD activity and phosphatidic acid–FeR interaction.
Enzyme FeR regulates intercellular adhesion and transmits signals initiated by growth factor receptors from the plasma membrane to the cytoskeleton. Kinase FeR is a cell volume-sensitive kinase that acts downstream from Fyn that targets α-, β-, and δ1 catenins [201]. It also phosphorylates TATA element modulatory factor TMF1 that can bind TATA element in RNA polymerase-2 promoters. Nuclear kinase FeR can modulate the suppressive activity of TMF during cell growth and differentiation [202].
In fibroblasts, FeR reduces cell adhesion to matrix, as it phosphorylates docking protein FAK-associated CRK-associated substrate CAS125 by activated protein Tyr phosphatase and increased Tyr phosphorylation of adherens junction proteins catenin-δ126 and β-catenin [199]. Conversely, protein Tyr phosphatases that dephosphorylate PTK substrates in adherens junctions and focal adhesions include at least plasmalemmal Tyr phosphatases PTPRa and PTPRs as well as cytoplamic Tyr phosphatases PTPn1, PTPn11, and PTPn12.
4.8 Fyn-Related Kinase
Like Tyr kinases Weel and Abl1, Fyn-related kinase27 is a nuclear protein. It is composed of SH2 and SH3 domains at the N-terminus and Tyr residues within the kinase domain and near the C-terminus. It is expressed primarily in epithelial cells.
It operates during G1 and S phases of the cell cycle to suppress cell proliferation. Kinase FRK interacts with retinoblastoma protein [203]. It also forms a signaling pathway with SH2-domain-containing adaptor SHB.28 This signaling axis involves focal adhesion kinase and insulin receptor substrates IRS1 and IRS2. In endothelial cells, SHB both promotes apoptosis under anti-angiogenic condition, but also participates in mitogenicity, spreading, and tubular morphogenesis [204]. The FRK–SHB pathway regulates cell apoptosis, proliferation, and differentiation.
The FRK family of Tyr kinases includes BrK, FRK, and SRMS [205]. Several FRK family kinases preclude the Ras pathway primed by activated receptor Tyr kinases. They phosphorylate KH-domain-containing, RNA-binding, signal transduction associated protein KhdRBS1 (or Sam68) and adaptor signal-transducing adaptor protein STAP2 (or BKS). Kinase FRK phosphorylates PTen, thereby avoiding PTen anchorage to Ub ligase NEDD4-1 and degradation [206]. As a positive regulator of PTen, FRK suppresses PKB signaling.
4.9 Janus Kinases
Janus kinases29 (JaK) are activated by cytokine receptors (Table 4.6). Intracellular Janus kinases are involved in the JaK–STAT pathway (STAT: signal transducer and activator of transcription).
Table 4.6
Activation of JaKs and STATs by cytokines (Source: [207]). Type-1 and -2 cytokine classification is related to 3D structure. Type-1 cytokine possesses either a short ( ∼ 15 amino acids) or long ( ∼ 25 amino acids) chain. Cytokines IL5 and M-CSF (or CSF1) bind as dimers, whereas other type-1 cytokines bind as monomers. Type-2 cytokines have different structures (βc, γc: common cytokine receptor β and γ chain; CNTF: ciliary neurotrophic factor; CT1: cardiotrophin-1; gCSF: granulocyte colony-stimulating factor; gmCSF: granulocyte–monocyte colony-stimulating factor; IL: interleukin; mCSF: macrophage colony-stimulating factor; OsM: oncostatin-M; LIF: leukemia-inhibitory factor; SCF: stem cell factor; TSLP: thymic stromal lymphopoietin; TyK2: tyrosine kinase-2).
Cytokines | JaKs | STATs |
---|---|---|
Type-1 cytokines | ||
Short-chain cytokines that share γc | ||
IL2/7/9/15/21 | JaK1/3 | STAT3/5a/5b |
IL4 | JaK1/3 | STAT6 |
Short-chain cytokines that share βc | ||
IL3/5, gmCSF (CSF2) | JaK2 | STAT5a/5b |
Short-chain cytokines; receptors with Tyr kinase domain | ||
mCSF (CSF1) | JaK1, TyK2 | STAT1/3/5a/5b |
SCF | STAT5a/5b | |
Other short-chain cytokines | ||
IL13 | JaK1/2, TyK2 | STAT6 |
TSLP | STAT5a/5b | |
Long-chain cytokines that share GP130 | ||
IL6/11, OsM, CNtF, | JaK1/2, TyK2 | STAT3 |
LIF, CT1 | ||
Other long-chain cytokines | ||
IL12 | JaK2, TyK2 | STAT4 |
Growth hormone | JaK2 | STAT3/5a/5b |
Prolactin, Epo, Tpo | JaK2 | STAT5a/5b |
Leptin | JaK2 | STAT3 |
gCSF (CSF3) | JaK1/2, TyK2 | STAT1/3/5a/5b |
Type-2 cytokines | ||
Ifnα/β | JaK1, TyK2 | STAT1/2/4 |
Ifnγ | JaK1/2 | STAT1 |
IL10 | JaK1, TyK2 | STAT3 |
IL20, IL22 | STAT3 |
The Janus kinase family includes 4 members (JaK1–JaK3 and Tyr kinase TyK2). Kinase JaK3 is strictly expressed in hematopoietic cells, whereas the other members of the JaK family (JaK1, JaK2, and TyK2) are ubiquitous.
Kinases JaK1 and JaK2 are involved in interferon-2 (interferon-γ) signaling (Vol. 2 – Chap. 3. Growth Factors). Kinases JaK1 and TyK2 associate with interferon-1. Kinase JaK2 is also an effector of prolactin receptor among others. Kinase Jak3 connects exclusively to a single cytokine receptor subunit (γ C ) and predominantly promotes lymphopoiesis, whereas the other members of the Janus kinase family interact with many different cytokine receptor subunits.30
4.9.1 Canonical and Non-Canonical Pathways of JaK Activation
Kinase JaK2 possesses a basal activity in the absence of dimerization and transautophosphorylation. Upon cytokine-induced receptor dimerization (canonical pathway), activated JaK that is pre-associated via receptor subunits dimerizes, transautophosphorylates (Tyr1007 and Tyr1008), and acquires high activity, as its enzymatic efficiency with respect to ATP increases by at least 4 orders of magnitude and its substrate recognition spectrum broadens. Besides autophosphorylation, JaK phosphorylates cytoplasmic domain of receptors that can then serve as docking sites for scaffold and signaling proteins such as signal transducers and activators of transcription.
In addition, JaKs can be activated in non-canonical pathways. These signaling cascades entail [208]: (1) G-protein-coupled receptors, such as AT1 angiotensin-2 receptor that operates via PKCδ, NADPH oxidase, and possibly FAK2 and Src kinases to activate JaK2; Gq/11-protein-coupled angiotensin receptor Mas1 (a proto-oncogene product) that responds to angiotensin-3 and angiotensin(1 − − 7) (but not angiotensin-2);31 B2 bradykinin receptor, CCK2 cholecystokinin receptor; opioid receptors; platelet-activating factor receptor; and CCR2 and CXCR4 chemokine receptors; (2) oxidative stress, as hydrogen peroxide can indirectly stimulate JaK2, but oxidative stress attenuates or inhibits JaK activity downstream from cytokine stimulation; (3) hyperglycemia that involves PKC and subsequent activation of NADPH oxidase and generation of ROS that inhibit Tyr phosphatase PTPn6 (or SHP1); and (4) possibly hyperosmolarity.
4.9.2 Signal Transducers and Activators of Transcription
The family of signal transducers and activators of transcription (STAT) includes 7 members (STAT1–STAT4, STAT5a–STAT5b, and STAT6). These transcription factors are phosphorylated (activated) by Tyr kinases (RTKs and NRTKs, such as JaKs, Src, and Abl) in response to many cytokines, growth factors, and hormones. Their phosphorylation leads to STAT homo- or heterodimerization. These DNA-binding proteins particularly mediate interferon-dependent gene expression. However, phosphorylated STAT dimers as well as unphosphorylated STATs can stimulate gene expression, but using distinct mechanisms [209]. In the absence of Tyr phosphorylation, STAT1 is able to activate the expression of a gene that encodes 20S proteasome subunit-β9 (PsmB9).32 Unphosphorylated STAT3 binds to unphosphorylated NFκB, hence competing with IκB. The unphosphorylated STAT3–NFκB complex then accumulates in the nucleus to activate target genes.
4.9.3 Other Regulation of JaK Activity
Oxidants such as nitric oxide can reversibly inhibit JaK kinases. PKCδ phosphorylates (inactivates) JaK2 kinase. Cytokine-induced JaK2 phosphorylation (Tyr570) and autophosphorylation at Tyr913 result from a negative feedback [208]. On the other hand, autophosphorylation at Tyr813 enhances JaK2 catalytic activity by creating a docking site for adaptor SH2B1 that selectively improves JaK2 activity. In addition, JaK2 nitration (Tyr1007 and Tyr1008) prevents its activity.
Therefore, phosphorylation of some target amino acid residues and nitration are post-translational inhibitory modification. On the other hand, phosphorylation of other target amino acid residues and interaction with adaptor proteins represent stimulatory controls. In leptin and insulin signaling, insulin receptor substrates IRS1 and IRS2 are recruited to the SH2B1–JaK2 complex. Their subsequent phosphorylation by JaK2 promotes their association with PI3K for PKB activation [208].
4.9.4 Janus Kinases in Heart
In cardiomyocytes, JaK1 and JaK2 are predominant members of the JaK family. Numerous members of the type-1 cytokine receptors33 that activate JaK1 and/or JaK2, hence STAT3, protect heart from acute and chronic oxidative stress owing to 2 intracellular signaling cascades. These pathways that are activated by most of these cytokines and rely on JaK1 and JaK2 kinases include: (1) reperfusion injury salvage kinase (RISK) pathway and (2) JaK–STAT signaling [208]. The RISK pathway involves activation of phosphatidylinositol 3-kinase and extracellular signal-regulated protein kinases ERK1 and ERK2, hence restraining mitochondrial permeability transition pore. Signaling from the JaK–STAT axis entails activation of transcription factor STAT3, upregulation of cyclooxygenase-2 and nitric oxide synthase-2 that also inhibits mitochondrial permeability transition pore, vascular endothelial growth factor, anti-oxidants manganese superoxide dismutase and metallothioneins MT134 and MT2 (or MT2a), as well as matrix metalloproteases for repair and scar formation [208]. Whereas auto- and paracrine action of IL6 initiates heart preconditioning, the JaK–STAT pathway ensures delayed preconditioning, the so-called second window of protection (SWOP).
Although Janus kinases such as JaK2 may be activated by acute oxidative stress to prime an anti-oxidative stress program, they can also be inhibited by oxidative stress in cardiomyocytes, especially JaK1 kinase.
4.9.5 Inhibitors of the JaK–STAT Pathway
Cytokines bind to their cognate plasmalemmal receptor to trigger the JaK–STAT pathway. However, various molecules restrain the signaling cascade [210]. Inhibitors of JaK–STAT signaling include: (1) cytokine-inducible SH2-containing proteins (CIS), (2) suppressors of cytokine signaling (SOCS), and (3) STAT-induced STAT inhibitors (SSI). Many JaK–STAT inhibitors (CIS, SOCS1–SOCS7) are expressed in mammalian cells stimulated by cytokines, such as ciliary neurotrophic factor, erythropoietin, growth hormone, granulocyte (CSF3)and granulocyte– macrophage (CSF2) colony-stimulating factors, interferon-α and -γ, interleukins, leptin leukemia inhibitory factor (LIF or cholinergic differentiation factor [CDF]), prolactin, and tumor-necrosis factor-α.
Suppressors of cytokine signaling can bind to receptors, especially insulin and IGF1 receptors. Cytokine-inducible SH2-containing protein impedes signaling from IL2, IL3, and erythropoietin. Agents SOCS1, SSI1, and JaK-binding protein (JAB) bind to and hamper JaK kinases. Protein SOCS1 inhibits IL1, IL4, IL6, and Ifnγ signaling [211]. It also suppresses Toll-like receptor TLR4 signaling [212]. Interactions between SOCS1 and TLR are mediated via Ifnα and -β [213]. Protein SOCS3 is expressed in response to IL2 in T cells and blood lymphocytes [214]. It interacts with IL2 receptor complex and phosphorylates JaK1 and IL2Rβ. It also inhibits growth hormone signaling by binding to GH receptor, then interacting with and hindering receptor-bound JaK2. Both SOCS1 and SOCS3 impede effects of the IL6 family of cytokines, as they suppress STAT3 phosphorylation, whereas CIS or SOCS2 do not hamper this signaling pathway. Factors SOCS1 and SOCS3 regulate IL6 and Ifnγ signaling. Protein SOCS3 prevents Ifnγ response in cells stimulated by IL6 [215].
Obesity is associated with an increase in SOCS1 and SOCS3 in liver, muscle, and, to a lesser extent, adipose tissue [216]. Phosphorylation of insulin receptor is partly impaired and that of insulin receptor substrates IRS1 and IRS2 is almost completely suppressed.35 Although both SOCS1 and SOCS3 bind to insulin receptor in an insulin-dependent manner, they do not affect insulin-dependent insulin receptor autophosphorylation. Therefore, SOCS1 and SOCS3 link insulin resistance to cytokine signaling, as they inhibit insulin signaling. Moreover, SOCS1 and SOCS6 inhibit insulin-dependent activation of ERK1, ERK2, and PKB in vivo [217]. The SOCS proteins contribute to the pathogenesis of type-2 diabetes.
4.10 MATK Kinase or CSK Homologous Kinase (CSK Subfamily)
Megakaryocyte-associated tyrosine kinase (MATK) of the CSK subfamily is also designated as CSK homologous kinase (CHK).36 Kinases CSK and MATK not only share significant sequence identity, but also can inactivate SRC family kinases by phosphorylating their regulatory Tyr in the C-terminus [218]. In addition, MATK can also inhibit SRC family kinases via a non-catalytic mechanism by binding to active SRC family kinases to form stable MATK–SFK complexes.
Kinase MATK contains Src homology binding domains (SH2 and SH3), a kinase sequence, and a unique N-terminus, but lacks myristylation signals, negative regulatory phosphorylation site, and autophosphorylation motif. Therefore, it is not regulated by Tyr phosphorylation. It interacts with many partners via its SH2 domain, whereas the SH3 domain governs its subcellular localization. Its recruitment to the plasma membrane is associated with inhibition of Lyn kinase.
Enzyme MATK can be detected in the cytosol, at the plasma membrane, as well as in the nucleus. However, it lacks the N-terminal fatty acid acylation domain for membrane anchoring. However, it needs to be positioned in proximity to SRC family kinases to phosphorylate these kinases that reside at the plasma membrane, endosomes, and perinuclear regions, using its SH2 domain to bind to specific phosphorylated Tyr residues as well as SH3 motif.
Kinase MATK abounds in megakaryocytes and brain cells. In human resting monocytes, its expression is induced upon stimulation by interleukins-3 and -4. In megakaryoblasts, its production rises upon stem cell factor excitation.
Three alternatively spliced transcript variants generate different isoforms. The conserved lysine that binds ATP corresponds to Lys221 in P52MATK and Lys262 in P56MATK [218]. Isoform P52MATK abounds in neurons, astrocytes, and oligodendrocytes, especially in the hippocampus, substantia nigra, and cortex. Expression of P56MATK is specific to hematopoietic cells.
Kinase MATK contributes to the regulation of megakaryocytopoiesis. In hematopoietic cells, it phosphorylates (inactivates) SRC family kinases. It hence inhibits T-cell proliferation. In addition to suppression of cell growth and proliferation, MATK is also implicated in the regulation of chromosome movement during mitosis [218].
4.11 SRMS Kinase (FRK Subfamily)
Non-receptor Tyr kinase Src-related kinase lacking regulatory and myristylation sites SRMS (or SRM) has SH2, SH2′, and SH3 domains and a Tyr residue for autophosphorylation in the kinase domain, but lacks N-terminal glycine for myristylation and a C-terminal Tyr that, once phosphorylated, suppresses kinase activity [221]. The expression of 2 transcripts is ubiquitous, but depends on cell type and developmental stage.
4.12 Kinases of the SRC Family
The SRC family (Src stands for sarcoma) of intracellular protein Tyr kinases are signaling proteins associated with cell adhesion, growth, proliferation, differentiation, survival, and migration, especially during angiogenesis (Vol. 5 – Chap. 10. Vasculature Growth) as well as regulation of ion channels in neuronal cells. Constitutive activation and overexpression of SRC family kinases (SFK) cause many types of cancers, such as breast and colon carcinomas.
The activity of SRC family kinases is controlled mainly by phosphorylation of 2 conserved tyrosines: (1) autophosphorylation site (YA) in the activation loop and (2) regulatory tyrosine located near the C-terminal tail (YT). The latter site is targeted by CSK and MATK kinases. Autophosphorylation at (YA) causes full activation, whereas phosphorylation at YT inactivates tha enzyme. The SRC family members have regions with similar sequences, the so-called Src homology (SH) domains: the SH1 domain is a catalytic sequence, whereas SH2 and SH3 domains are protein-binding motifs. The SH2 domain usually binds phosphotyrosine-containing proteins and SH3 interacts with cytoskeletal proteins. Upon YT phosphorylation, SFKs adopt an inactive conformation stabilized by 2 major intramolecular interactions: (1) binding of phosphorylated YT to the SH2 domain and (2) binding of a segment linking SH2 and kinase domains (SH2–kinase linker) to the SH3 domain.
The SRC family of Tyr kinases includes include many members, which are reversibly coupled to the inner leaflet of the plasma membrane: Src, Yes, FGR, Fyn, LCK, Lyn, BLK, and HCK enzymes. Cytoplasmic kinases of the SRC family are either ubiquitous (e.g., Fyn, Src, and Yes) or have a restricted expression pattern (BLK, FGR, HCK, LCK, and Lyn).
The SRC family can be decomposed into subfamilies: (1) the SRCA subfamily that comprises FGR, Fyn, Src, and Yes; (2) the SRCB subfamily that consists of BLK, HCK, LCK, and Lyn; and (3) the FRK subfamily. The TEC family constitutes a subset of Src kinases that is composed of BMX, BTK, ITK, TEC, and TXK kinases. Kinases of the TEC family possess a pleckstrin- and TEC-homology domain.37
Cytosolic Src kinase is often self-restrained to an inactive conformation. The balance between its constitutively active Tyr kinases (CSK and MATK) and phosphatases (e.g., PTPRa) in both basal and stimulated states is partially responsible for the state of Src activation.
Src kinase becomes activated during the transit from the perinuclear region to the plasmalemma, which requires the actin cytoskeleton and RhoB-associated endosomes.38 Disruptions in actin filaments inhibit both the membrane translocation and activity of Src [223]. The active Src colocalizes with RhoB in the perinuclear region. Fibronectin is a main Src-activating extracellular stimulus. Src- and RhoB-containing structures are associated with Src-promoted, polymerized actin.
4.12.1 SRC Family Kinases during Hypoxia
Kinases of the SRC family are highly expressed in pulmonary arteries, where they are implicated in vascular smooth muscle cell contraction. Hypoxic pulmonary vasoconstriction corresponds to an acute, regional, adaptive process that directs blood flow away from poorly ventilated regions of the lung to support the ventilation–perfusion matching. In isolated pulmonary arteries, hypoxic pulmonary vasoconstriction is a biphasic process characterized by a first stage with a transient elevation in intracellular calcium level and a second sustained phase with a gradually rising then stabilized intracellular calcium level. Hypoxia hastens Src kinases that triggers RoCK kinase activation (Sect. 5.2.14) that mediates Ca + + sensitization during the second phase of vasoconstriction [224]. Subsequent inhibition of myosin light-chain phosphatase via phosphorylation of PP1r12a by RoCK kinase leads to increased phosphorylation of myosin light chain MLC20.39 In cultured pulmonary artery smooth muscle cells, hypoxia not only enhances phosphorylation of both PP1r12a and MLC20, but also causes translocation of RoCK kinase from the nucleus to the cytoplasm.
Both Src and Fyn kinases are activated during hypoxia in cardiomyocytes [225, 226]. Hypoxia also increases production of reactive oxygen species and subsequent Src phosphorylation to promote the synthesis of hypoxia-inducible factor-1α (Sect. 10.9.2) and plasminogene activator inhibitor-1 in rodent aortic smooth muscle cells [227].
Engagement of B-cell receptor immediately activates receptor-associated kinases of the SRC family (BLK, Fyn, Lyn, and LCK). This response primes phosphorylation of cellular substrates. Triggered signaling cascades are composed of effectors Ras GTPase-activating protein, phosphatidylinositol 3-kinase, phospholipases PLCγ1 and PLCγ2, and extracellular signal-regulated protein kinase. Three distinct sites allow the interaction of these kinases with effectors. The unique N-terminal domain of Lyn mediates association with PLCγ2, MAPK, and RasGAP; Src homology SH3 domain with PI3K; and SH2 domain with a relatively small proportion of PI3K, PLCγ2, MAPK, and RasGAP [228]. Kinases BLK, Lyn, and Fyn differ in their ability to bind MAPK and PI3K kinases. Therefore, they preferentially bind and subsequently phosphorylate their effectors.
4.12.2 BLK Kinase (SRCB Subfamily)
B-lymphoid Tyr kinase, also designated as B-lymphocyte specific Tyr kinase,40 is specifically expressed in the B-cell lineage [230]. In humans, 2 BLK transcripts arise from the transcription of gene Blk by 2 distinct promoters that can be regulated by different transacting factors [231]. Kinase BLK interacts with ubiquitin ligase to be degraded.
4.12.3 BMX Kinase (TEC Family)
Bone marrow Tyr kinase gene in chromosome-X product (BMX)41 is characterized by an N-terminal pleckstrin homology domain, Src homology SH3 and SH2 segments, and a catalytic kinase motif. It belongs to the TEC family that also includes TEC, TXK, ITK, and BTK, which are marked by an N-terminal TEC homology domain located downstream from the PH domain.
Kinase BMX mediates activation of Rho GTPase by Gα12 and -13 upon stimulation by hormones and neurotransmitters. It interacts with P21-activating Ser/Thr kinase PAK1 [232]. The latter maps small GTPases Rho, CDC42, and Rac1 onto cytoskeleton organization and nuclear signaling on the one hand, and associates with FAK and non-receptor protein Tyr phosphatase PTPN21 to increase STAT3 activation, and RUN and FYVE domain-containing protein RUFY1 that is involved in vesicular transport (early endosomes) on the other.
Kinase BMX operates in endothelial cells and lymphocytes, as well as cardiomyocytes [233]. It indeed participates in pressure overload-induced hypertrophic growth. Tumor-necrosis factor can provoke angiogenesis. This factor activates BMX specifically via TNFR2 [234]. Kinase BMX forms pre-existing complex with TNFR2 (independently from TNF). Activated BMX then mediates TNF-induced migration of endothelial cells and tube formation.
In endothelial cells, the endocannabinoid anandamide connects to cannabinoid receptor-1 (CB1R) and G-protein-coupled receptor GPR55 to initiate distinct signaling pathways, whether integrins are inactive or not, respectively [235]. Signaling primed by CB1R includes Gi-protein-mediated activation of spleen Tyr kinase to translocate NFκB and inhibit phosphoinositide 3-kinase. Yet, PI3K operates in GPR55-triggered signaling. On the other hand, integrin aggregation provokes CB1R splitting from integrins and subsequent SYK inactivation. Therefore, SYK cannot further inhibit GPR55-triggered signaling. Consequently, anandamide generates Ca + + influx from the endoplasmic reticulum (ER) via the GPR55–PI3K–BMX–PLC pathway and activates nuclear factor of activated T-cells.
In lymphocytes, TLR2, TLR4, and α5β1 integrin signal via MyD88 and focal adhesion kinase [236]. Toll-like receptors recruit adaptors, such as Mal, MyD88, TRIF, and TRAM to activate 2 distinct pathways, the TIRAP–Mal–MyD88–NFκB–AP142 or TRIF–TRAM–IRF axes.43 Kinase BMX is involved in crosstalk between the integrin–FAK and MyD88 pathways. In macrophages, BMX regulates IL6 production caused by Toll-like receptors independently of P38MAPK and NFκB [237]. On the other hand, BTK intervenes in production of inflammatory cytokine tumor-necrosis factor-α, but not IL6.
4.12.4 BTK Kinases (TEC Family)
Bruton Tyr kinase (BTK),44 a member of the TEC family of cytoplasmic protein Tyr kinases, contributes to B-cell maturation as well as mastocyte activation via IgE receptors. The Btk gene is located on the X chromosome. Kinase BTK is constitutively associated with phosphatidylinositol 4-phosphate 5-kinase that synthesizes PI(4,5)P2 [238]. The BTK–PI(4)P5K complex localizes to membrane rafts, where BTK binds phosphatidylinositol (3,4,5)-trisphosphate, then phosphorylates phospholipase-Cγ2.
Kinase BTK is a dual-function regulator of apoptosis, as it promotes stress-induced apoptosis but prevents TNFSF6-activated apoptosis in B cells [239]. When B lymphocytes are exposed to reactive oxygen species, BTK lowers the anti-apoptotic activity of transcription factor STAT3. On the other hand, BTK associates with TNFRSF6a death receptor and impairs its interaction with Fas (TNFRSF6a)-associated protein with death domain FADD that helps TNFRSF6a to recruit and activate caspase-8, thereby precluding the assembly of the death-inducing signaling complex.
4.12.5 FGR Kinase (SRCA Subfamily)
FGR (viral feline Gardner-Rasheed sarcoma oncogene homolog) kinase is a member of the SRCA subfamily of protein Tyr kinases. Protein FGR contains in its N-terminus sites for myristylation and palmitylation. Its catalytic domain as well as SH2 and SH3 motifs are involved in protein–protein interactions.
Leukocytes express 3 different subfamilies of Tyr kinases: (1) SRC, (2) SYK, and (3) FAK family kinases. In leukocytes of the myeloid lineage, detected SRC family kinases include FGR, HCK, and Lyn. Enzymes SYK and FAK2 are predominant members of their respective subfamilies. On the other hand, in leukocytes of the lymphoid lineage, ZAP70 and FAK are the main members. Kinases of the SRC family FGR, HCK, and Lyn, as well as SYK, are activated in stimulated granulocytes (mainly FGR and Lyn that relocalize to the actin cytoskeleton) and macrophages.45 Resulting signaling events increase the number and affinity of cell adhesion molecules, especially integrins (Table 4.7).
Table 4.7
Integrins produced by phagocytes and their ligands (Source: [240]; CR3: complement receptor-3; C3bi: fragment of complement component C3; GP: glycoprotein; HW: high-molecular-weight kininogen; ICAM: intercellular adhesion molecule; LFA: lymphocyte function-associated antigen; Mac1: macrophage-1 antigen; NIF: neutrophil inhibitory factor; VCAM: vascular cell adhesion molecule; VLA: very late antigen). Phagocytes are the leukocytes that ingest harmful foreign particles, from pathogens to dying cells. They are called professional or non-professional whether they phagocytize effectively or not. Professional phagocytes include neutrophils, monocytes, macrophages, and dendritic and mast cells that have surface receptors to detect foreign bodies. Non-professional phagocytes comprise lymphocytes, epithelial and endothelial cells, fibroblasts, and mesenchymal cells. Phagocytes are recruited by chemoattractants (cytokines, chemokines, clotting peptides, complement components, and invading microbe products) released by invaders or phagocytes already at work. Chemotaxis of phagocytes implies crossing of the endothelial barrier for circulating cells and displacement through a matrix of transmigrated leukocytes and tissue-resident phagocytes, hence transient cell adhesion. Contact with foreign bodies trigger a chemical attack with reactive oxygen species and possibly delayed antigen presentation to lymphocytes close to working site (macrophages) or remotely in lymph nodes (dendritic cells). Extracellular killing relies on interferon-γ released by CD4 + and CD8 + T lymphocytes, natural killer (NK) and NKT cells, B lymphocytes, monocytes, macrophages, and dendritic cells to stimulate macrophages to produce and deliver toxic level of nitric oxide.
Integrins | Other Alias | Ligands |
---|---|---|
β1 Integrins | ||
α4β1 | CD49d–CD29, VLA4 | Fibronectin, VCAM1 |
α5β1 | CD49e–CD29, VLA5 | Fibronectin |
α6β1 | CD49f–CD29, VLA6 | Laminin |
β2 Integrins | ||
αLβ2 | CD11a–CD18, LFA1 | ICAM1, ICAM2, ICAM3 |
αMβ2 | CD11b–CD18, | ICAM1, ICAM2, fibrinogen, |
CR3, | factor X, HW, β-glucan, | |
Mac1 | heparin, NIF, C3bi, elastase, | |
oligodeoxynucleotides | ||
αXβ2 | CD11c–CD18, | Fibrinogen, C3bi, |
GP150–95 | lipopolysaccharides | |
αDβ2 | CD11d–CD18 | ICAM3 |
β3 Integrins | ||
α v β3 | CD51–CD61 | Vitronectin, entactin |
Kinase FGR, as well as HCK and Lyn, are dispensable for development of myeloid cells as well as most of their functions, but they are indispensable for integrin-mediated signaling [240]. Integrin-mediated adhesion is a potent costimulus for neutrophil activation. Integrin clustering consecutive to bound ligands primes signaling that culminates in actin cytoskeletal rearrangement and neutrophil migration, degranulation (i.e., release of granule constituents, such as hydrolytic enzymes, metal-binding proteins, and peroxidases), and oxidative burst (i.e., rapid release of reactive oxygen species after assembly of NADPH oxidase subunits at the plasma membrane).
4.12.6 Fyn Kinase (SRCA Subfamily)
Kinase Fyn, a member of the SRCA subfamily of protein Tyr kinases, is primarily located on the cytoplasmic leaflet of the plasma membrane. It phosphorylates various substrates to regulate their activity and/or to generate a binding site for signaling effectors. Protein Fyn participates in signaling initiated by T- and B-cell receptors, excited integrins, growth factors, activated platelet, and gated ion channels [241]. Alternatively spliced variants exist. Most tissues express FynB, whereas T lymphocytes synthesize FynT.
Activated Fyn autophosphorylates (Tyr417). Kinase Fyn binds to P85 subunit of phosphatidylinositol 3-kinase and Fyn-binding protein (FyB). Kinase CSK phosphorylates (inactivates) Fyn (Tyr528). Transmembrane protein Tyr phosphatase receptors PTPRa, PTPRc, and PTPRf as well as protein Tyr phosphatase PTPn6 dephosphorylate Fyn (Tyr528) [241]. Phosphatase PTPn5 also dephosphorylates Fyn (Tyr417). Depalmitoylated Fyn is released from membrane, hence hindering Fyn-mediated phosphorylation of membrane-bound substrates.
Protein Fyn interacts with [241]: (1) receptor protein Tyr kinases;46 (2) immunocyte receptors;47 (3) interleukin receptors (IL2R–IL5R and IL7R); (4) Tyr kinases (BTK, FAK, JAK2, and PTK2b); (5) Ser/Thr kinases (PKCδ and PKCη); (6) Tyr phosphatases (endoplasmic reticulum phosphatase StEP61 as well as PTPn11 and PTPRa); (7) adaptors and scaffolds;48 (8) cell surface and/or GPI-linked proteins;49 (9) ion channels;50 (10) cytokeletal and adhesion proteins;51 (11) GTPase-activating proteins ( RhoGAP32, RhoGAP35 and RasA1 [a RasGAP]); and (12) other proteins ( CBL and Itch ubiquitin ligases, PI3K, Rapsyn, KH domain-containing, RNA-binding, signal transduction-associated proteins KhdRBS1 and KhdRBS2, BCL3, and TNFSF6).
Among these partners, Fyn particularly targets regulators of the cytoskeletal structure in both hematopoietic and non-hematopoietic cells [242]. Fyn Kinase binds to Wiskott-Aldrich syndrome protein. Members of the WASP family associate with numerous signaling molecules to depolymerize actin directly and/or serve as adaptors or scaffolds for these mediators to regulate the cytoskeleton dynamics.
4.12.7 Hemopoietic Cell Kinase (SRCB Subfamily)
Hemopoietic cell kinase (HCK) is predominantly expressed in hemopoietic cells. It can help to couple Fc receptor to activation of the respiratory burst (or oxidative burst), i.e., the rapid release of reactive oxygen species. In addition, it can intervene in neutrophil migration and degranulation. Two alternatively spliced variants exist with different subcellular locations.
Kinase HCK interacts with Breakpoint cluster region protein (BCR) [243], actin-binding proteins WASP, WASP-interacting protein (WIP), phagocytosis promotor Engulfment and cell motility ElMo1 [244] adaptor/Ub ligase CBL, GTPase-activating proteins RasGAPs RasA1 and RasA3 [245], RapGEF1 [246], granulocyte colony-stimulating factor receptor [247], and adamlysin ADAM15 [248].52
4.12.8 ITK Kinase (TEC Family)
Intracellular Tyr kinase or IL2-inducible T-cell kinase (ITK) is expressed in T lymphocytes.53 It is produced in T, NK, and mast cells. Kinase ITK pertains to the TEC family, the member of which are involved in signaling emitted by cytokine receptors, immunoreceptors, and other lymphoid cell surface receptors. Adhesion molecule CD2 on the surface of T lymphocytes and natural killer cells and costimulator CD28, a constitutively expressed B7 receptor on naive T lymphocytes provokes phosphorylation (activation) of ITK by LCK [249].
Kinase ITK interacts with CD2, kinases Fyn and Src, phospholipase-Cγ1, suppressor of cytokine signaling protein SOCS1, as well as adaptors GRB2, CBL, and lymphocyte cytosolic protein LCP2,54 and linker of activated T cells (LAT),55 in addition to DNA-binding protein KhdRBS1 (or Sam68) and heterogeneous nuclear ribonucleoprotein hnRNPk, Wiskott-Aldrich syndrome protein that activates the ARP2–ARP3 complex for actin nucleation, karyopherin-α2 (KpnA2) involved in nuclear transport of proteins, and peptidylprolyl isomerase-A (PPIa; a.k.a. cyclophilin-A) that accelerates protein folding [250, 251].
4.12.9 Leukocyte-Specific Cytosolic Kinase (SRCB Subfamily)
Leukocyte-specific cytoplasmic protein Tyr kinase (LCK), a member of the SRCB subfamily, is observed in lymphocytes, where it phosphorylates signaling mediators. It associates with the cytoplasmic tails of CD4 and CD8 coreceptors on T helper and cytotoxic T cells, respectively, to assist signaling from T-cell receptors.
Kinase LCK phosphorylates (activates) kinase ZAP70 that, in turn, phosphorylates transmembrane adaptor linker of activated T cells (LAT) that is a docking site for various proteins, such as SHC, GRB2, SOS, PI3K, and PLC. The corresponding cascade provokes Ca + + influx and activation of MAPK module to activate NFAT, NFκB, and AP1 transcription factors.
Protein LCK phosphorylates numerous substrates, such as SCFR, CD3, and IL2R receptors, CD44 that participates in lymphocyte activation, CD48, cell-surface antigens, such as B-cell membrane-spanning 4-domain MS4A1 and thymocyte protein THY1, TNFRSF6a receptor and its TNFSF6 ligand, cytotoxic T-lymphocyte-associated antigen-4, TCR-interacting molecule TRAT1, Notch1, membrane enzymes, such as Axl and PTPRC, kinases ZAP70, ITK, FAK2, PI3K, PI4K-α, and PKCθ, protein Tyr phosphatase PTPn6, PTPn11 (SHP2)-interacting transmembrane adaptor SIT1, cRaf kinase, RasGAP RasA1, RhoGAP17, Vav1 GEF, adpribosylation factor-related protein ArfRP1, CSK-binding protein PAG1, Src-associated phosphoprotein ScAP1, phospholipase-C, KhdRBS1, CRK- associated substrate-related protein NEDD9 (or CASL), adamlysins ADAM10 and ADAM15, paxillin, PECAM1, catenin-δ1, and Lnk, LCP2, and SHC adaptors, CBL adaptor and Ub ligase, UbE3a ubiquitin ligase, and sequestosome Sqstm1 [251].
4.12.10 Lyn Kinase (SRCB Subfamily)
Kinase Lyn (viral yes-1 Yamaguchi sarcoma-related oncogene homolog) belongs to the SRCB subfamily of protein Tyr kinases. It is mainly expressed in hematopoietic and nervous cells.
Kinase Lyn can be recruited with G-protein subunit Gi to plasmalemmal nanoclusters supported by CD59 (a.k.a. protectin and membrane inhibitor of reactive lysis [MIRL]), a complement regulatory protein. Once in this nanocluster, Lyn can transiently activate phospholipase-Cγ that produces inositol trisphosphate from phosphatidylinositol bisphosphate.
Upon B-cell receptor activation, Lyn is rapidly phosphorylated. Activated Lyn triggers a cascade of signaling events that starts with phosphorylation of immunoreceptor Tyr-based activation motifs (ITAM) of receptors to recruit and stimulate effectors, such as SYK, PLCγ2, and PI3K. Other interacting kinases include TyK2, TEC, BTK, FAK1 and FAK2, JaK2, ERK1, CK2, S6K, and CDK1, -2, and -4 [251].
Kinase Lyn also transmits inhibitory signals via phosphorylation of immunoreceptor Tyr-based inhibitory motifs (ITIM) in regulators, such as inhibitor for B-cell receptor CD22, pair of Ig-like receptors PIR-B, FcαR, and FCγR2b1 to recruit and stimulate phosphatases, such as SHIP1 and PTPn6 (SHP1; immunotolerance) [252].
Lyn Kinase also interacts with erythropoietin receptor, cytokine receptors SCFR (Kit or CD117), CSF1R and CSF3R, glycoprotein receptor for collagen GP6 on platelet, cell adhesion molecule CD24 that resides on most B lymphocytes, integral membrane protein CD36 that binds many ligands, mucin-1, PECAM1, membrane neutral metallo-endopeptidase neprilysin, PDE4a, protein Tyr phosphatase receptor PTPRc, Tyr phosphatase PTP6, inhibitory PP1r8 and PP1r15a subunits of protein phosphatase-1, inositol polyphosphate-5-phosphatase Inpp5d (SHIP1), Src kinase-associated phosphoproteins ScAP1 and ScAP2, adaptor lymphocyte cytosolic protein LCP2, cytotoxic T-lymphocyte antigen CTLA4, PML-RARA regulated adaptor molecule PRAM1, CAS-Br-M ecotropic retroviral transforming sequence (CBLC), docking protein DOK1, adaptors Gab2 and Gab3, SHC, breast cancer anti-estrogen resistance BCAR1 (or p130CAS), neural precursor cell expressed, developmentally downregulated molecule NEDD9, B-cell scaffold protein with ankyrin repeats BAnk1, and ion channel TRPV4 [251].
4.12.11 Src Kinase
Src Kinase (or cSrc: cellular Src) is encoded by the SRC proto-oncogene. Mutations of the SRC gene yield cancers. Two splice variants exist. The protein consists of 3 domains: N-terminal SH3 and central SH2 protein-binding sites and kinase domain. The SH2 and SH3 domains cooperate in Src auto-inhibition. Various mechanisms activate Src: (1) C-terminus dephosphorylation by a protein Tyr phosphatase; (2) binding of the SH2 domain by a phosphoprotein such as focal adhesion kinase; and (3) binding of the SH3 domain.
Src corresponds to the prototype of the cytoplasmic, membrane-associated, Tyr kinases that acts as a cotransducer of mitogenic signals emanating from numerous Tyr kinase growth factor receptors, such as those for platelet-derived, epidermal, and fibroblast FGF2 growth factors, and colony-stimulating factor CSF1. Interactions between Src and these receptors are bidirectional, i.e., Src phosphorylates (activates) these receptors and vice versa.
Preferred substrates of Src include almost exclusively molecules that associate with the actin cytoskeleton or focal adhesions, such as cortactin, RhoGAP35, and CAS (or BCAR1) [253]. On the other hand, preferential substrates of growth factor receptors such as EGFR comprise the receptor itself, phospholipase-Cγ, and SHC and DOK1 adaptors. Major mitogenic signaling proceeds directly from the receptor and uses the SHC–GRB2–SOS–Ras–Raf–MAPK–ELK1 axis. Cell proliferation needs actin cytoskeleton, hence Src kinase and its substrates. Kinase Src then serves as transducers of growth signals and/or monitors of anti-apoptotic conditions.
Signaling from PDGF involves 43 potential Src kinase substrates [254]. In particular, PDGF provokes the phosphorylation by Src of calcium-dependent, non-lysosomal cysteine protease calpain-2, epidermal growth factor receptor pathway substrate EPS15, ubiquitin-associated protein UbAP2l, RNA-binding motif protein RBM10, Far upstream element (FUSE)-binding protein FUBP1, TRK-fused gene product TFG, and transcriptional cocontroller Tripartite motif-containing protein TriM28.
Kinase Src contributes to angiotensin-2-induced signal transduction and cardiac hypertrophy [255]. Moreover, Src can be activated during myocardial ischemia. Although, CSK that phosphorylates (inactivates; Tyr527) Src56 is upregulated in failing left ventricles, Src is also markedly upregulated with respect to normal subjects. Increased CSK expression in response to hypertrophic stimuli seems inconsistent with its antihypertrophic effect. However, it can imply that these kinases are required for a fine-tuning of the response.
4.12.12 TEC Kinase
Non-receptor protein Tyr kinase expressed in hepatocellular carcinoma (TEC), or PSCTK4, is encoded by the Tec gene. It is involved in intracellular signaling from cytokine receptors, lymphocyte immunoreceptors, G-protein-coupled receptors, and integrins (Table 4.8). Kinase TEC is indeed able to interact with Gα12 subunit of heterotrimeric G protein, SCFR cytokine receptor, docking protein DOK1, Janus kinase JaK2, suppressor of cytokine signaling SOCS1, and RhoGEF12. Kinase TEC thus contributes to the regulation of the immune response.
Table 4.8
Extracellular stimuli that regulate cell fate exert their action by interacting with plasmalemmal receptors to generate intracellular signaling. Various extracellular signals activate TEC family kinases. There are 3 consecutive activation steps: (1) recruitment to the membrane; (2) phosphorylation by SRC family kinases; and (3) autophosphorylation. Negative feedbacks exist. According to cell and signal types, several pathways can be triggered to regulate cell proliferation, differentiation, apoptosis, migration, and adhesion. One set of receptors, the receptor protein Tyr kinases (RTK), includes receptors for growth factors (e.g., PDGFR, EGFR, and CSF1R). A second set is constituted by cytokine receptors (e.g., IL2R–IL7R, IL9R, IL11R–IL13R, IL15R, IL21R, IL23R, IL27R, CSF2R, CSF3R, EpoR) that correspond to aggregation of receptor subunits to form functional receptors, without enzymatic activity, but by recruiting cytoplasmic Tyr kinases. In both receptor sets, signaling primed by extracellular interaction between ligands and receptors triggers protein phosphorylation.
Receptor type | Mediators |
---|---|
Cytokine receptor | JaK, STAT |
Fc receptor | WASP, SLP76, SYK, PLCγ1/2, PKC, SRC family kinase |
Toll-like receptor | IRAK1, MAL, MYD88, TRIF, TRAM |
Death receptor | FADD |
GPCR | G protein |
RTK | PI3K, SRC family kinase |
Integrin | FAK, cytoskeletal proteins |
NRPTK, CBL, PI3K, PtdIns, PLCγ, | |
DAG, PKC, IP3, Ca + + , PLD, Arf | |
Vav, Ras, ERK1/2, JNK | |
CDC42, Rac, Rho, JNK, cytoskeletal proteins |
Kinase TEC is a potent activator of the transcription of cytokine genes, such as IL2 and IL4. It favors the activity of nuclear factor of activated T cells by, at least, enhancing NFAT nuclear import [256]. It is characterized by a unique subcellular localization in small vesicles at the plasma membrane upon signaling from T-cell receptor (but not other family members) [257]. Kinase TEC colalizes with kinase LCK, PLC1, and early endosomal antigen-1 marker (EEA1).
In addition to TEC kinase (PSCTK4), the TEC family includes protein Tyr kinases BMX (PSCTK2/3), BTK (PSCTK1), ITK (PSCTK2), and TXK (PSCTK5) that are encoded by the Bmx, Btk, Itk, and TXK genes, respectively. Kinases of the TEC family possess several between-protein interaction and localization domains, such as a SH2 domain for interactions with phosphotyrosine moieties, a SH3 domain for linkage with proline-rich sequences, and a PH domain (cysteine-rich string in TXK) for membrane recruitment, as it binds to PI3K products PI(3,4)P2 and PI(3,4,5)P3.
The main goal of TEC family kinases is the activation of phospholipase-Cγ1 to produce inositol trisphosphate and diacylglycerol, hence causing Ca + + influx and activating calcium-dependent enzymes such as PP3, in addition to protein kinase-C and Ras GTPase. Kinases of the TEC family participate in the control of cell survival, proliferation, differentiation, migration, and apoptosis (Tables 4.9, 4.10, and 4.11).
Kinase | Partners |
---|---|
BMX | STAT3 |
BTK | Factin, TNFRSF6a |
PKCβ1/2, Fyn, HCK, Lyn | |
Gαq/12, Gβγ, Vav | |
BAP135, EWSR1, CBL, SH3BP5, KhdRBS1, WASP, SLP65 | |
ITK | CD28, Gαq, Gβγ, PLCγ |
GRB2, hnRNPk | |
TEC | SCFR, CD28, PI3K(p55γ) |
BRDG1/STAP1, SLP76, Vav |
Receptor type | Signaling pathways |
---|---|
GPCR | BTK–JNK–Jun |
TEC–CDC42/Rac/Rho–cytoskeleton | |
RTK | GRB2–SOS–Ras–Raf–ERK–Fos |
PI3K–BTK–CDC42/Rac/Rho–cytoskeleton | |
Cytokine R | BMX |
JaK–STAT | |
BCR | Lyn–BTK |
SYK–BLNK–PLCγ–DAG/IP3–PKC/Ca + + –NFAT | |
SYK–Vav–CDC42/Rac/Rho | |
TCR | ZAP–LAT–SLP76–PLCγ–DAG/IP3–PKC/Ca + + –NFAT |
LCK–ITK–PLCγ–DAG/IP3–PKC/Ca + + –NFAT |
Kinase | Expression | Stimulus |
---|---|---|
BMX | Bone marrow, heart, | IL-6R |
lung, testis, colon | G12/13 | |
macrophage, neutrophil | ||
BTK | Bone marrow, spleen, | BCR, FcεR1, GP130, |
lymph node | IL5R, IL6R, IL10R, | |
B, myeloid, erythroid, | CD19, CD28, CD38, CD40 | |
and mast cells, | ||
megakaryocyte | ||
ITK | Thymus, spleen, | TCR, FcεR1, |
lymph node | CD2, CD28 | |
T, NK, and mast cells | ||
TXK | Thymus, spleen, | TCR |
lymph node, tonsil, | ||
testis | ||
T and myeloid cells | ||
TEC | Bone marrow, spleen, | BCR, TCR, GP130, |
thymus | CD28, CD38, IL3R, IL6R, | |
T, B, and myeloid cells | EpoR, TpoR, SCFR, G-CSFR |
Kinases of the TEC family are regulators of lymphocyte activation and effector function. In T lymphocytes, they comprise TEC, ITK, and TXK, whereas in B lymphocytes the main member is BTK. Unlike in B lymphocytes, in which BTK is indispensable for development and activation, TEC kinases modulate function of activated T lymphocytes. Kinases of the TEC family are indeed less effective than SRC or SYK family kinases. Whereas B lymphocytes are able to synthesize BTK and TEC and T lymphocytes ITK, TXK, and TEC, mastocytes produce BTK, ITK, TEC, and TXK.
4.12.13 TXK Kinase (TEC Family)
Non-receptor protein Tyr kinase mutated in X-linked agammaglobulinemia (TXK)57 is a member of the TEC subset of the SRC family. It is synthesized in T lymphocytes and some types of the myeloid cell lineage. Like other members of the TEC family, TXK is associated with membrane rafts and can be phosphorylated (activated) by kinases of the SRC family.
Naive T lymphocytes differentiate into T helper TH1 or TH2 cells. Both cytokine receptor and T-cell receptor-mediated signaling direct this differentiation. Interleukin-12 and interferon-γ determines TH1 differentiation, whereas IL4 leads to TH2 development. In addition, distinct signaling effectors mediate cytokine expression in TH1 and TH2 cells. Protein TXK importantly contributes to TH1 cytokine production [262].
4.12.14 Yes Kinase (SRCA Subfamily)
Kinase Yes1 (viral yes-1 Yamaguchi sarcoma oncogene homolog) belongs to the SRCA subfamily. It is detected in cerebellar Purkinje cells. It colocalizes with occludin in tight junctions in epithelial and endothelial cells [263].
Kinase Yes1 interacts with many identified partners [251]: (1) plasmalemmal receptors, such as colony-stimulating factor-1 receptor, CD36 of the class-B scavenger receptor family,58 and CD46, a complement regulatory protein; (2) kinases, either plasmalemmal such as EPHb2 or cytoplasmic, such as Fyn, JaK2, and FAK1, as well as P13K; (3) phosphatases such as PP2; (4) adhesion molecules, such as PECAM1, a label to destroy aged neutrophils by macrophages, catenin-δ1 (encoded by the CTNND1 gene) that operates in cell adhesion and signal transduction, cadherin-1, and occludin at tight junctions; (5) partners of small GTPases such as RasGAP RasA1; (6) adaptor, docking, and scaffold proteins such as DOK1; and (7) other proteins that have diverse functions, such ribosomal protein RPL10, a constituent of large ribosomal 60S subunit that binds to Jun transcription factor, ion channel TRPV4, sodium–hydrogen antiporter-3 regulator SLC9a3R1 (or NHERF), and nephrin, a transmembrane protein involved in the renal filtration barrier.
4.13 From Src to SYK
Intracellular signals delivered by kinases of the SRC family, SYK, and ZAP70, are coordinated by adaptors or linkers that mediate protein–protein and, in some cases, protein–lipid interactions. Adaptors allow immunoreceptors and assigned kinases to come into contact with their targets.59
Two families of cytosolic Tyr kinases, the Src and SYK families, are required for T-cell receptor signaling. T-cell receptor engaged with processed antigen fragments presented by antigen-presenting cells primes the sequential activation of Src (LCK and Fyn) and SYK (SYK and ZAP70) kinases. Upon TCR activation, LCK is activated and phosphorylates the intracellular ITAM segment of CD3 of the TCR–CD3 complex. Phosphorylation of ITAM allows binding of ζ-chain-associated protein kinase ZAP70 to CD3. This kinase then phosphorylates adaptor LCP2 (also known as SLP76) that interacts with both adaptor GRB2 and phospholipase-Cγ1.
4.14 Spleen Tyrosine Kinase
The SYK family of non-receptor protein Tyr kinases is composed of 2 members: spleen Tyr kinase (SYK) and ζ-associated protein-70 (ZAP70). Kinase SYK is produced in hematopoietic cells (B lymphocytes, mastocytes, neutrophils, macrophages, platelets, etc.) as well as other cell types, whereas expression of ZAP70 is restricted to T and natural killer cells. Alternatively spliced variant SYKb is less active than SYK protein.
Kinase SYK intervenes in both innate and adaptive immunity. It also participates in diverse processes, such as cell adhesion, osteoclast maturation,60 platelet activation,61 and vascular development. In particular, SYK is required for the separation of newly formed lymphatic vessels from blood vessels [264].
It is activated by immunoreceptors of the adaptive immune response (B- [BCR] and T-cell [TCR] receptors as well as various Ig receptors [FcR]) as well as C-type lectins62 and integrins.63
Enzyme SYK is recruited to aggregated immune recognition receptors with dually phosphorylated immunoreceptor Tyr-based activation motif (ITAM), such as B-cell antigen receptor, IgE receptor (FcεR1), multiple IgG receptors (FcγR1–R3), T-cell antigen receptor, DAP12 coreceptor associated with non-inhibitory Ly49D and H receptors on NK cells, and FcR γ-chain component of platelet collagen receptor [265].64
The resulting conformational change stimulates its autophosphorylation. Activation of SYK is enhanced via phosphorylation by members of the SRC family. Phosphorylation of SYK (Tyr of linker region between SH2 and catalytic domain) allows its interactions with effectors, such as guanine nucleotide-exchange factor Vav, phospholipase-Cγ2, and CBL ubiquitin ligase.
Kinase SYK also associates with IL2 receptor β-chain as well as receptors for G-CSF, GM-CSF, IL3, IL5, IL15, and erythropoietin, in addition to Toll-like receptor TLR4 [265]. Numerous signaling mediators and adaptors also link to SYK [265]. Partners of SYK include [251] (Table 4.12): (1) receptors, such as EpoR, CSF3R, Fc fragment of IgG FCGR2A, Fc fragment of IgE FCER1, GFCRL3, MS4A2, CD3E, CD4, CD19, CD22, CD72, CD79A/B, and CR3; (2) cytoskeleton components and cytoskeleton- and cell adhesion-associated molecules, such as cortactin, paxillin, and tubulin-α1a; (3) kinases, such as BTK, FGR, Fyn, LCK, Lyn, Src, FAK1/2, PKCα, PRKD1 (PKCμ), and ribosomal protein S6 kinases S6K1 and S6K2; (4) phosphatases such as PTP6; (5) phospholipases, such as PLCγ1 and PLCγ2; (6) chaperone α-synuclein; (7) partners of small GTPases such as Vav GEF; and (8) adaptors and scaffolds, such as GRB2, SHC, CRKL, SLAP, TRAF6, BCAP, BAnk, BLnk, SiRPB1, PAG1, SLA, SH3BP2, hematopoietic cell-specific Lyn substrate HCLS1 (or HS1), LCP2 (or SLP76), and SH3P7, as well as adaptor/Ub ligase CBL; (5) transmembrane adaptors in membrane rafts, such as linker for activation of T cells (LAT) in T and NK cells, mastocytes, and platelets), linker for activation of B cells (LAB)65 in B and NK cells, monocytes, and mastocytes, and linker for activation of X cells (LAX) in both B and T cells as well as monocytes.
Table 4.12
Direct and indirect partners of spleen Tyr kinase and effects (Source: [264]; BCR: B-cell receptor; BCL: B-cell lymphoma; BLnk: B-cell linker protein; CARD: caspase-recruitment domain; CBL: ubiquitin ligase Casitas B-lineage lymphoma; DAG: diacylglycerol; ERK: extracellular signal-regulated kinase; FAK: focal adhesion kinase; FcR: (Ig) Fc receptor; JNK: Janus kinase; LAT: linker for activation of T cells; LCP: lymphocyte cytosolic protein; MALT: mucosa-associated lymphoid tissue lymphoma translocation protein; NFAT: nuclear factor of activated T cells; NFκB: nuclear factor-κB; NLRP: NLR family, pyrin domain-containing protein; PI3K: phosphatidylinositol 3-kinase; PKB(C): protein kinase-B(C); PLC: phospholipase-C; PTPn: protein Tyr phosphatase non-receptor; RasGRP: RAS guanyl-releasing protein; ROS, reactive oxygen species; TCR: T-cell receptor; TEC: tyrosine kinase expressed in hepatocellular carcinoma).
Receptors | BCR, TCR, FcR, C-type lectin, integrin |
---|---|
Binding partners | Vav, PLCγ, PI3K, LCP2, BLnk |
Signaling effectors | Ca + + , DAG, LAT, CARD9–BCL10–MALT1, NLRP3, |
Rho, RasGRP, PKB, PKC, FAK2, TEC, ERK, P38MAPK, | |
JNK, NFAT, NFκB | |
Inhibitors | CBL, PTPn6 |
Effects | Cell adhesion, differentiation, proliferation, survival, |
Cytoskeletal reorganization, | |
Production of reactive oxygen species, | |
Immunity, cytokine release, inflammasome activation, | |
Transition from proB cell to preB cell, | |
Early thymocyte development (DN3–DN4 transition), | |
Activity of macrophage, mastocyte, neutrophils, | |
Lymphangiogenesis, platelet activation, | |
Bone resorption |
In phagocytosis of pathogens and apoptotic cells, SYK operates downstream from receptor FcγR and integrin-α M β2 (also called complement receptor CR3) in macrophages,66 NK-cell receptor-like C-type lectin Dectin-1 in dendritic cells, and apoptotic cell-recognizing receptor [266].
Kinase SYK operates in signal transduction primed by activated immunoreceptors, such as B-cell receptor, Fc receptors, and activating receptors of NK cells [266]. Ligation of immunoreceptor actually leads to activation of different members of the SRC family kinase according to the cell type that phosphorylate (activate) the immunoreceptor. The latter then attracts and activates SYK, as SYK undergoes conformational changes and autophosphorylation. Activated SYK phosphorylates various substrates, such as adaptor SH2-domain-containing leukocyte protein SLP76 and the Vav family of guanine nucleotide-exchange factors or B-cell linker protein (BLnk).
Kinase SYK, indeed, acts not only in immunoreceptor signaling, but also cue transduction from integrins in certain hematopoietic cells, such as platelets, neutrophils, and monocytes, in addition to osteoclasts [266].67 Molecules DAP12 and FcγR are able to associate with SYK and mediate β2-integrin signaling in neutrophils and macrophages. In addition, α2Bβ3-integrin that is expressed in cells of the megakaryocyte lineage is involved in platelet aggregation and granule secretion when platelets bind to fibrinogen. Integrin-α2Bβ3–fibrinogen binding leads to activation not only of HCK, FGR, Lyn, and Src kinases, but also of SYK [266]. Neutrophils also need SYK for degranulation upon integrin–ligand binding as well as main SRC family kinases that exist in the myeloid lineage to produce reactive oxygen species.
Kinases Src and SYK cooperatively transmit signals downstream of excited integrins. A kinase of the SRC family that can associate with integrins is activated, adaptors undergo phosphorylation, and then SYK is recruited and stimulated.
References
1.
Ezkurdia I, Del Pozo A, Frankish A, Rodriguez JM, Harrow J, Ashman K, Valencia A, Tress ML (2012) Comparative proteomics reveals a significant bias toward alternative protein isoforms with conserved structure and function. Molecular Biology and Evolution(mbe.oxfordjournals.org/content/early/2012/03/22/molbev.mss100.abstract)
2.
Chavent G (2010) Nonlinear Least Squares for Inverse Problems, Theoretical Foundations and Step-by-Step Guide for Applications. Springer, New York
3.
Bensoussan A (1971) Filtrage optimal des systèmes linéaires [Optimal filtering of linear systems] Dunod, Paris
4.
Bertoglio C, Moireau P, Gerbeau JF (2012) Sequential parameter estimation for fluid-structure problems. Application to hemodynamics. International Journal for Numerical Methods in Biomedical Engineering 28:434–455MathSciNet
5.
Lombardi D, Iollo A, Colin T, Saut O (2009) Inverse problems in tumor growth modelling (communication at CEMRACS summer school)
6.
Lagaert JB (2011) Modélisation de la croissance tumorale [Tumor Growth Modeling], PhD thesis, Bordeaux University
7.
van Meer G, Voelker DR, Feigenson GW (2008) Membrane lipids: where they are and how they behave. Nature Reviews – Molecular Cell Biology 9:112–124
8.
D’Arrigo P, Servi S (2010) Synthesis of lysophospholipids. Molecules 15:1354–1377
9.
Voet D, Voet JG (2011) Signal Transduction (Chap 19). Biochemistry (4th edition), Wiley, Hoboken, New Jersey
10.
Berridge MJ (2009) Module 2: Cell Signalling Pathways. Cell Signalling Biology. Biochemical Journal’s Signal Knowledge Environment Portland Press Ltd., London, UK (www.biochemj.org/csb/002/csb002.pdf)
11.
Delon C, Manifava M, Wood E, Thompson D, Krugmann S, Pyne S, Ktistakis NT (2004) Sphingosine kinase 1 is an intracellular effector of phosphatidic acid. Journal of Biological Chemistry 279:44763–44774
12.
Carricaburu V, Lamia KA, Lo E, Favereaux L, Payrastre B, Cantley LC, Rameh LE (2003) The phosphatidylinositol (PI)-5-phosphate 4-kinase type II enzyme controls insulin signaling by regulating PI-3,4,5-trisphosphate degradation. Proceedings of the National Academy of Sciences of the United States of America 100:9867–9872ADS
13.
Maag D, Maxwell MJ, Hardesty DA, Boucher KL, Choudhari N, Hanno AG, Ma JF, Snowman AS, Pietropaoli JW, Xu R, Storm PB, Saiardi A, Snyder SH, Resnick AC (2011) Inositol polyphosphate multikinase is a physiologic PI3-kinase that activates Akt/PKB. Proceedings of the National Academy of Sciences of the United States of America 108: 1391–1396ADS
14.
Weirich CS, Erzberger JP, Flick JS, Berger JM, Thorner J, Weis K (2006) Activation of the DExD/H-box protein Dbp5 by the nuclear-pore protein Gle1 and its coactivator InsP6 is required for mRNA export. Nature – Cell Biology 8:668–676
15.
Alcázar-Román AR, Tran EJ, Guo S, Wente SR (2006) Inositol hexakisphosphate and Gle1 activate the DEAD-box protein Dbp5 for nuclear mRNA export. Nature – Cell Biology 8: 711–716
16.
Macbeth MR, Schubert HL, VanDemark AP, Lingam AT, Hill CP, Bass BL (2005) Inositol hexakisphosphate is bound in the ADAR2 core and required for RNA editing. Science 309:1534–1539ADS
17.
Huang YH, Grasis JA, Miller AT, Xu R, Soonthornvacharin S, Andreotti AH, Tsoukas CD, Cooke MP, Sauer K (2007) Positive regulation of Itk PH domain function by soluble IP4. Science 316:886–889ADS
18.
Shen X, Xiao H, Ranallo R, Wu WH, Wudagger C (2003) Modulation of ATP-dependent chromatin-remodeling complexes by inositol polyphosphates. Science 299:112–114ADS
19.
Mulugu S, Bai W, Fridy PC, Bastidas RJ, Otto JC, Dollins DE, Haystead TA, Ribeiro AA, York JD (2007) A conserved family of enzymes that phosphorylate inositol hexakisphosphate. Science 316:106–109ADS
20.
Lee YS, Mulugu S, York JD, O’Shea EK (2007) Regulation of a cyclin-CDK-CDK inhibitor complex by inositol pyrophosphates. Science 316:109–112ADS
21.
Saiardi A, Bhandari R, Resnick AC, Snowman AM, Snyder SH (2004) Phosphorylation of proteins by inositol pyrophosphates. Science 306:2101–2105ADS
22.
Chakraborty A, Koldobskiy MA, Sixt KM, Juluri KR, Mustafa AK, Snowman AM, van Rossum DB, Patterson RL, Snyder SH (2008) HSP90 regulates cell survival via inositol hexakisphosphate kinase-2. Proceedings of the National Academy of Sciences of the United States of America 105:1134-1139ADS
23.
Szado T, Vanderheyden V, Parys JB, De Smedt H, Rietdorf K, Kotelevets L, Chastre E, Khan F, Landegren U, Söderberg O, Bootman MD, Roderick HL (2008) Phosphorylation of inositol 1,4,5-trisphosphate receptors by protein kinase B/Akt inhibits Ca2+ release and apoptosis. Proceedings of the National Academy of Sciences of the United States of America 105: 2427–2432ADS
24.
Otto JC, Kelly P, Chiou ST, York JD (2007) Alterations in an inositol phosphate code through synergistic activation of a G protein and inositol phosphate kinases. Proceedings of the National Academy of Sciences of the United States of America 104:15653–15658ADS
25.
Zhang C, Majerus PW, Wilson MP (2012) Regulation of inositol 1,3,4-trisphosphate 5/6-kinase (ITPK1) by reversible lysine acetylation. Proceedings of the National Academy of Sciences of the United States of America 109:2290–2295ADS
26.
Ohnishi T, Ohba H, Seo KC, Im J, Sato Y, Iwayama Y, Furuichi T, Chung SK, Yoshikawa T (2007) Spatial expression patterns and biochemical properties distinguish a second myo-inositol monophosphatase IMPA2 from IMPA1. Journal of Biological Chemistry 282: 637–646
27.
Berggard T, Szczepankiewicz O, Thulin E, Linse S (2002) Myo-inositol monophosphatase is an activated target of calbindin D28k. Journal of Biological Chemistry 277:41954–41959
28.
Irvine RF (2002) Nuclear lipid signaling. Science Signaling 150:re13
29.
Schouten A, Agianian B, Westerman J, Kroon J, Wirtz KWA, Gros P (2002) Structure of apo-phosphatidylinositol transfer protein α provides insight into membrane association. EMBO Journal 21:2117–2121
30.
Woodcock EA, Kistler PM, Ju YK (2009) Phosphoinositide signalling and cardiac arrhythmias. Cardiovascular Research 82:286–295
31.
Jao CY, Roth M, Welti R, Salic A (2009) Metabolic labeling and direct imaging of choline phospholipids in vivo. Proceedings of the National Academy of Sciences of the United States of America 106:15332–15337ADS
32.
Breslow DK, Collins SR, Bodenmiller B, Aebersold R, Simons K, Shevchenko A, Ejsing CS, Weissman JS (2010) Orm family proteins mediate sphingolipid homeostasis. Nature 463:1048–1053ADS
33.
Pavoine C, Pecker F (2009) Sphingomyelinases: their regulation and roles in cardiovascular pathophysiology. Cardiovascular Research 82:175–183
34.
Cogolludo A, Moreno L, Frazziano G, Moral-Sanz J, Menendez C, Castañeda J, González C, Villamor E, Perez-Vizcaino F (2009) Activation of neutral sphingomyelinase is involved in acute hypoxic pulmonary vasoconstriction. Cardiovascular Research 82:296–302
35.
Karliner JS (2009) Sphingosine kinase regulation and cardioprotection. Cardiovascular Research 82:184–192
36.
Means CK, Brown JH (2009) Sphingosine-1-phosphate receptor signalling in the heart. Cardiovascular Research 82:193–200
37.
Gellings Lowe N, Swaney JS, Moreno KM, Sabbadini RA (2009) Sphingosine-1-phosphate and sphingosine kinase are critical for transforming growth factor-beta-stimulated collagen production by cardiac fibroblasts. Cardiovascular Research 82:303–312
38.
Sanchez T, Skoura A, Wu MT, Casserly B, Harrington EO, Hla T (2007) Induction of vascular permeability by the sphingosine 1-phosphate receptor-2 (S1P2R) and its downstream effectors ROCK and PTEN. Arteriosclerosis, Thrombosis, and Vascular Biology 27:1312–1318
39.
Sattler K, Levkau B (2009) Sphingosine-1-phosphate as a mediator of high-density lipoprotein effects in cardiovascular protection. Cardiovascular Research 82:201–211
40.
Igarashi J, Michel T (2009) Sphingosine-1-phosphate and modulation of vascular tone. Cardiovascular Research 82:212-220
41.
Frias MA, James RW, Gerber-Wicht C, Lang U (2009) Native and reconstituted HDL activate Stat3 in ventricular cardiomyocytes via ERK1/2: Role of sphingosine-1-phosphate. Cardiovascular Research 82:313–323
42.
Ke Y, Lei M, Solaro RJ (2008) Regulation of cardiac excitation and contraction by p21 activated kinase-1. Progress in Biophysics and Molecular Biology 98:238–250
43.
Jenkins CM, Cedars A, Gross RW (2009) Eicosanoid signalling pathways in the heart. Cardiovascular Research 82:240–249
44.
Zhao J, O’Donnell VB, Balzar S, St Croix CM, Trudeau JB, Wenzel SE (2011) 15-Lipoxygenase 1 interacts with phosphatidylethanolamine-binding protein to regulate MAPK signaling in human airway epithelial cells. Proceedings of the National Academy of Sciences of the United States of America 108:14246–14251ADS
45.
Jacobs ER, Zeldin DC (2001) The lung HETEs (and EETs) up. American Journal of Physiology – Heart and Circulatory Physiology 280:H1–H10
46.
Watanabe K (2011) Recent reports about enzymes related to the synthesis of prostaglandin (PG) F2 (PGF2α and 9α, 11β-PGF2). Journal of Biochemistry 150:593–596
47.
Yeaman SJ (2004) Hormone-sensitive lipase – new roles for an old enzyme. Biochemical Journal 379:11–22
48.
Okazaki H, Igarashi M, Nishi M, Sekiya M, Tajima M, Takase S, Takanashi M, Ohta K, Tamura Y, Okazaki S, Yahagi N, Ohashi K, Amemiya-Kudo M, Nakagawa Y, Nagai R, Kadowaki T, Osuga J, Ishibashi S (2008) Identification of neutral cholesterol ester hydrolase, a key enzyme removing cholesterol from macrophages. Journal of Biological Chemistry 283:33357–33364
49.
Jaye M, Lynch KJ, Krawiec J, Marchadier D, Maugeais C, Doan K, South V, Amin D, Perrone M, Rader DJ (1999) A novel endothelial-derived lipase that modulates HDL metabolism. Nature – Genetics 21:424–428
50.
Strauss JG, Zimmermann R, Hrzenjak A, Zhou Y, Kratky D, Levak-Frank S, Kostner GM, Zechner R, Frank S (2002) Endothelial cell-derived lipase mediates uptake and binding of high-density lipoprotein (HDL) particles and the selective uptake of HDL-associated cholesterol esters independent of its enzymic activity. Biochemical Journal 368:69–79
51.
Kojima Y, Ishida T, Sun L, Yasuda T, Toh R, Rikitake Y, Fukuda A, Kume N, Koshiyama H, Taniguchi A, Hirata KI (2010) Pitavastatin decreases the expression of endothelial lipase both in vitro and in vivo. Cardiovascular Research 87:385–393
52.
Yano M, Matsumura T, Senokuchi T, Ishii N, Murata Y, Taketa K, Motoshima H, Taguchi T, Sonoda K, Kukidome D, Takuwa Y, Kawada T, Brownlee M, Nishikawa T, Araki E (2007) Statins activate peroxisome proliferator-activated receptor γthrough extracellular signal-regulated kinase 1/2 and p38 mitogen-activated protein kinase-dependent cyclooxygenase-2 expression in macrophages. Circulation Research 100:1442–1451
53.
Favari E, Zanotti I, Zimetti F, Ronda N, Bernini F, Rothblat GH (2004) Probucol inhibits ABCA1-mediated cellular lipid efflux. Arteriosclerosis, Thrombosis, and Vascular Biology 24:2345–2350
54.
Aoki J, Inoue A, Makide K, Saiki N, Arai H (2007) Structure and function of extracellular phospholipase A1 belonging to the pancreatic lipase gene family. Biochimie 89:197–204
55.
Aoki J, Nagai Y, Hosono H, Inoue K, Arai H (2002) Structure and function of phosphatidylserine-specific phospholipase A1. Biochimica et Biophysica Acta 1582:26–32
56.
Wassum KM, Ostlund SB, Maidment NT, Balleine BW (2009) Distinct opioid circuits determine the palatability and the desirability of rewarding events. Proceedings of the National Academy of Sciences of the United States of America 106:12512–12517ADS
57.
D’Agostino D, Lowe ME (2004) Pancreatic lipase-related protein 2 is the major colipase-dependent pancreatic lipase in suckling mice. Journal of Nutrition 134:132–134
58.
Alexander SPH, Mathie A, Peters JA (2009) Guide to Receptors and Channels (GRAC), 4th edn., British Journal of Pharmacology 158:S1–S254 (www3.interscience.wiley.com/ journal/122684220/issue)
59.
Burke JE, Dennis EA (2009) Phospholipase A2 structure/function, mechanism, and signaling. Journal of Lipid Research 50:S237–S242
60.
Schaloske RH, Dennis EA (2006) The phospholipase A2 superfamily and its group numbering system. Biochimica et Biophysica Acta (BBA) – Molecular and Cell Biology of Lipids 1761:1246–1259
61.
Rosa AO, Rapoport SI (2009) Intracellular- and extracellular-derived Ca2 + influence phospholipase A2-mediated fatty acid release from brain phospholipids. Biochimica et Biophysica Acta 1791:697–705
62.
Murakami M, Taketomi Y, Sato H, Yamamoto K (2011) Secreted phospholipase-A2 revisited. Journal of Biochemistry 150:233–255
63.
Lee JC, Simonyi A, Sun AY, Sun GY (2011) Phospholipases A2 and neural membrane dynamics: implications for Alzheimer’s disease. Journal of Neurochemistry 116:813–819
64.
Perrin-Cocon L, Agaugué S, Coutant F, Masurel A, Bezzine S, Lambeau G, André P, Lotteau V (2004) Secretory phospholipase A2 induces dendritic cell maturation. European Journal of Immunology 34:2293–2302
65.
Ancian P, Lambeau G, Mattéi MG, Lazdunski M (1995) The human 180-kDa receptor for secretory phospholipases A2. Molecular cloning, identification of a secreted soluble form, expression, and chromosomal localization. Journal of Biological Chemistry 270:8963–8970
66.
Triggiani M, Granata F, Oriente A, De Marino V, Gentile M, Calabrese C, Palumbo C, Marone G (2000) Secretory phospholipase A2 induce β-glucuronidase release and IL-6 production from human lung macrophages. Journal of Immunology 164:4908–4915
67.
Tada K, Murakami M, Kambe T, Kudo I (1998) Induction of cyclooxygenase-2 by secretory phospholipases A2 in nerve growth factor-stimulated rat serosal mast cells is facilitated by interaction with fibroblasts and mediated by a mechanism independent of their enzymatic functions. Journal of Immunology 161:5008–5015
68.
Ibeas E, Fuentes L, Martin R, Hernandez M, Nieto ML (2009) Secreted phospholipase A2 type IIA as a mediator connecting innate and adaptive immunity: new role in atherosclerosis. Cardiovascular Research 81(1):54-63
69.
Murakami M, Kudo I (2003) New phospholipase A2 isozymes with a potential role in atherosclerosis. Current Opinion in Lipidology 14:431–436
70.
Kuksis A, Pruzanski W (2008) Phase composition of lipoprotein SM/cholesterol/PtdCho affects FA specificity of sPLA2s. Journal of Lipid Research 49:2161–2168
71.
The HUGO Gene Nomenclature Committee (HGNC; www.genenames.org/genefamilies/ PNPLA) Phospholipases
72.
Wilson PA, Gardner SD, Lambie NM, Commans SA, Crowther DJ (2006) Characterization of the human patatin-like phospholipase family. Journal of Lipid Research 47:1940–1949
73.
Kienesberger PC, Oberer M, Lass A, Zechner R (2009) Mammalian patatin domain containing proteins: a family with diverse lipolytic activities involved in multiple biological functions. Journal of Lipid Research 50:S63–S68
74.
Sun GY, Shelat PB, Jensen MB, He Y, Sun AY, Simonyi A (2010) Phospholipases A2 and inflammatory responses in the central nervous system. Neuromolecular Medicine 12:133–148
75.
Ohto T, Uozumi N, Hirabayashi T, Shimizu T (2005) Identification of novel cytosolic phospholipase A2s, murine cPLA2δ, ε, and ζ, which form a gene cluster with cPLA2β. Journal of Biological Chemistry 280:24576–24583
76.
Hoffmann R, Valencia A (2004) A gene network for navigating the literature. Nature – Genetics 36:664 (Information Hyperlinked over Proteins www.ihop-net.org/)
77.
Alberghina M (2010) Phospholipase-A2: new lessons from endothelial cells. Microvascular Research 80:280–285
78.
Suh PG, Park JI, Manzoli L, Cocco L, Peak JC, Katan M, Fukami K, Kataoka T, Yun S, Ryu SH (2008) Multiple roles of phosphoinositide-specific phospholipase C isozymes. BMB Reports 41:415–434
79.
Waldo GL, Ricks TK, Hicks SN, Cheever ML, Kawano T, Tsuboi K, Wang X, Montell C, Kozasa T, Sondek J, Harden TK (2010) Kinetic scaffolding mediated by a phospholipase C-β and Gq signaling complex. Science 330:974–980ADS
80.
Gutman O, Walliser C, Piechulek T, Gierschik P, Henis YI (2010) Differential regulation of phospholipase C-β2 activity and membrane interaction by Gαq, Gβ1γ2, and Rac2. Journal of Biological Chemistry 285:3905–3915
81.
Hunter I, Mascall KS, Ramos JW, Nixon GF (2011) A phospholipase Cγ1-activated pathway regulates transcription in human vascular smooth muscle cells. Cardiovascular Research 90:557–564
82.
von Essen MR, Kongsbak M, Schjerling P, Olgaard K, Odum N, Geisler C (2010) Vitamin D controls T cell antigen receptor signaling and activation of human T cells. Nature – Immunology 11:344–349
83.
Kobayashi M, Lomasney JW (2010) Phospholipase C δ4. UCSD-Nature Molecule Pages, UCSD-Nature Signaling Gateway (www.signaling-gateway.org)
84.
Wing MR, Bourdon DM, Harden TK (2003) PLC-ε: a shared effector protein in Ras-, Rho-, and Gαβγ-mediated signaling. Molecular Interventions 3:273–280
85.
Hu L, Edamatsu H, Takenaka N, Ikuta S, Kataoka T (2010) Crucial role of phospholipase Cepsilon in induction of local skin inflammatory reactions in the elicitation stage of allergic contact hypersensitivity. Journal of Immunology 184:993–1002
86.
Stewart AJ, Morgan K, Farquharson C, Millar RP (2007) Phospholipase C-η enzymes as putative protein kinase C and Ca2 + signalling components in neuronal and neuroendocrine tissues. Neuroendocrinology 86:243–248
87.
Nomikos M, Blayney LM, Larman MG, Campbell K, Rossbach A, Saunders CM, Swann K, Lai FA (2005) Role of phospholipase C-ζdomains in Ca2 + -dependent phosphatidylinositol 4,5-bisphosphate hydrolysis and cytoplasmic Ca2 + oscillations. Journal of Biological Chemistry 280:31011–31018
88.
Martinson EA, Scheible S, Greinacher A, Presek P (1995) Platelet phospholipase D is activated by protein kinase C via an integrin α2b β3-independent mechanism. Biochemical Journal 310:623–628
89.
Gironcel D, Racaud-Sultan C, Payrastre B, Haricot M, Borchert G, Kieffer N, Breton M, Chap H (1996) α2b β3-integrin mediated adhesion of human platelets to a fibrinogen matrix triggers phospholipase C activation and phosphatidylinositol 3’,4’-biphosphate accumulation. FEBS Letters 389:253–256
90.
Elvers M, Stegner D, Hagedorn I, Kleinschnitz C, Braun A, Kuijpers ME, Boesl M, Chen Q, Heemskerk JW, Stoll G, Frohman MA, Nieswandt B (2010) Impaired α2b β3 integrin activation and shear-dependent thrombus formation in mice lacking phospholipase D1. Science Signaling 3:ra1
91.
Mahankali M, Peng HJ, Henkels KM, Dinauer MC, Gomez-Cambronero J (2011) Phospholipase D2 (PLD2) is a guanine nucleotide exchange factor (GEF) for the GTPase Rac2. Proceedings of the National Academy of Sciences of the United States of America 108:19617–19622ADS
92.
Medina-Tato DA, Ward SG, Watson ML (2007) Phosphoinositide 3-kinase signalling in lung disease: leucocytes and beyond. Immunology 121:448–461
93.
Foster FM, Traer CJ, Abraham SM, Fry MJ (2003) The phosphoinositide (PI) 3-kinase family. Journal of Cell Science 116:3037–3040
94.
Vanhaesebroeck B, Guillermet-Guibert J, Graupera M, Bilange B (2010) The emerging mechanisms of isoform-specific PI3K signalling. Nature Reviews – Molecular Cell Biology 11:329–341
95.
Yuan TL, Choi HS, Matsui A, Benes C, Lifshits E, Luo J, Frangioni JV, Cantley LC (2008) Class 1A PI3K regulates vessel integrity during development and tumorigenesis. Proceedings of the National Academy of Sciences of the United States of America 105:9739–9744ADS
96.
Foukas LC, Berenjeno IM, Gray A, Khwaja A, Vanhaesebroeck B (2010) Activity of any class IA PI3K isoform can sustain cell proliferation and survival. Proceedings of the National Academy of Sciences of the United States of America 107:11381–11386ADS
97.
Kurig B, Shymanets A, Bohnacker T, Prajwal, Brock C, Ahmadian MR, Schaefer M, Gohla A, Harteneck C, Wymann MP, Jeanclos E, Nürnberg B (2009) Ras is an indispensable coregulator of the class IB phosphoinositide 3-kinase p87/p110γ. Proceedings of the National Academy of Sciences of the United States of America 106:20312–20317ADS
98.
Shinohara M, Terada Y, Iwamatsu A, Shinohara A, Mochizuki N, Higuchi M, Gotoh Y, Ihara S, Nagata S, Itoh H, Fukui Y, Jessberger R (2002) SWAP-70 is a guanine-nucleotide-exchange factor that mediates signalling of membrane ruffling. Nature 416:759–763ADS
99.
Ihara S, Oka T, Fukui Y (2006) Direct binding of SWAP-70 to non-muscle actin is required for membrane ruffling. Journal of Cell Science 119:500–507
100.
Donald S, Humby T, Fyfe I, Segonds-Pichon A, Walker SA, Andrews SR, Coadwell WJ, Emson P, Wilkinson LS, Welch HC (2008) P-Rex2 regulates Purkinje cell dendrite morphology and motor coordination. Proceedings of the National Academy of Sciences of the United States of America 105:4483–4488ADS
101.
Oudit GY, Penninger JM (2009) Cardiac regulation by phosphoinositide 3-kinases and PTEN. Cardiovascular Research 82:250–260
102.
Foukas LC, Claret M, Pearce W, Okkenhaug K, Meek S, Peskett E, Sancho S, Smith AJ, Withers DJ, Vanhaesebroeck B (2006) Critical role for the p110α phosphoinositide-3-OH kinase in growth and metabolic regulation. Nature 441:366–370ADS
103.
Knight ZA, Gonzalez B, Feldman ME, Zunder ER, Goldenberg DD, Williams O, Loewith R, Stokoe D, Balla A, Toth B, Balla T, Weiss WA, Williams RL, Shokat KM (2006) A pharmacological map of the PI3-K family defines a role for p110α in insulin signaling. Cell 125:647–649
104.
Jia S, Liu Z, Zhang S, Liu P, Zhang L, Lee SH, Zhang J, Signoretti S, Loda M, Roberts TM, Zhao JJ (2008) Essential roles of PI(3)K-p110β in cell growth, metabolism and tumorigenesis. Nature 454:776–779ADS
105.
Kumar A, Fernadez-Capetillo O, Carrera AC (2010) Nuclear phosphoinositide 3-kinase β controls double-strand break DNA repair. Proceedings of the National Academy of Sciences of the United States of America 107:7491–7496ADS
106.
Hawkins PT, Stephens LR (2007) PI3Kγ is a key regulator of inflammatory responses and cardiovascular homeostasis. Science 318:64–66ADS
107.
Park SJ, Lee KS, Kim SR, Min KH, Moon H, Lee MH, Chung CR, Han HJ, Puri KD, Lee YC (2010) Phosphoinositide 3-kinase δ inhibitor suppresses IL-17 expression in a murine asthma model. European Respiratory Journal (doi:10.1183/09031936.00106609)
108.
Marwick JA, Caramori G, Casolari P, Mazzoni F, Kirkham PA, Adcock IM, Chung KF, Papi A (2010) A role for phosphoinositol 3-kinase δ in the impairment of glucocorticoid responsiveness in patients with chronic obstructive pulmonary disease. Journal of Allergy and Clinical Immunology 125:1146–1153
109.
Beltran L, Chaussade C, Vanhaesebroeck B, Cutillas PR (2011) Calpain interacts with class IA phosphoinositide 3-kinases regulating their stability and signaling activity. Proceedings of the National Academy of Sciences of the United States of America 108:16217–16222ADS
110.
Miller S, Tavshanjian B, Oleksy A, Perisic O, Houseman BT, Shokat KM, Williams RL (2010) Shaping development of autophagy inhibitors with the structure of the lipid kinase Vps34. Science 327:1638–1642ADS
111.
Matsunaga K, Saitoh T, Tabata K, Omori H, Satoh T, Kurotori N, Maejima I, Shirahama-Noda K, Ichimura T, Isobe T, Akira S, Noda T, Yoshimori T (2009) Two Beclin 1-binding proteins, Atg14L and Rubicon, reciprocally regulate autophagy at different stages. Nature – Cell Biology 11:385–396
112.
Yan Y, Flinn RJ, Wu H, Schnur RS, Backer JM (2009) hVps15, but not Ca2 + /CaM, is required for the activity and regulation of hVps34 in mammalian cells. Biochemical Journal 417: 747–755
113.
Okada M, Jang SW, Ye K (2008) Akt phosphorylation and nuclear phosphoinositide association mediate mRNA export and cell proliferation activities by ALY. Proceedings of the National Academy of Sciences of the United States of America 105:8649–8654ADS
114.
Platanias LC (2005) Mechanisms of type-I and type-II-interferon-mediated signalling. Nature Reviews – Immunology 5:375–386
115.
Higuchi M, Onishi K, Kikuchi C, Gotoh Y (2008) Scaffolding function of PAK in the PDK1–Akt pathway. Nature – Cell Biology 10:1356–1364
116.
Park SW, Zhou Y, Lee J, Lu A, Sun C, Chung J, Ueki K, Ozcan U (2010) The regulatory subunits of PI3K, p85α and p85β, interact with XBP-1 and increase its nuclear translocation. Nature – Medicine 16:429–437
117.
Morello F, Perino A, Hirsch E (2009) Phosphoinositide 3-kinase signalling in the vascular system. Cardiovascular Research 82:261–271
118.
Blero D, Payrastre B, Schurmans S, Erneux C (2007) Phosphoinositide phosphatases in a network of signalling reactions. Pflugers Archiv – European Journal of Physiology 455: 31–44
119.
Jin N, Chow CY, Liu L, Zolov SN, Bronson R, Davisson M, Petersen JL, Zhang Y, Park S, Duex JE, Goldowitz D, Meisler MH, Weisman LS (2008) VAC14 nucleates a protein complex essential for the acute interconversion of PI3P and PI(3,5)P(2) in yeast and mouse. EMBO Journal 27:3221–3234
120.
Balla A, Balla T (2006) Phosphatidylinositol 4-kinases: old enzymes with emerging functions. Trends in Cell Biology 16:351–361
121.
Hsuan J, Waugh MG, Minogue S (2008) Phosphatidylinositol 4-kinase type 2α. UCSD-Nature Molecule Pages, UCSD-Nature Signaling Gateway (www.signaling-gateway.org)
122.
Berditchevski F, Tolias KF, Wong K, Carpenter CL, Hemler ME (1997) A novel link between integrins, transmembrane-4 superfamily proteins (CD63 and CD81), and phosphatidylinositol 4-kinase. Journal of Biological Chemistry 272:2595–2598
123.
Nishikawa K, Toker A, Wong K, Marignani PA, Johannes FJ, Cantley LC (1998) Association of protein kinase Cμ with type II phosphatidylinositol 4-kinase and type I phosphatidylinositol-4-phosphate 5-kinase. Journal of Biological Chemistry 273: 23126–23133
124.
Kauffmann-Zeh A, Thomas GM, Ball A, Prosser S, Cunningham E, Cockcroft S, Hsuan JJ (1995) Requirement for phosphatidylinositol transfer protein in epidermal growth factor signaling. Science 268:1188–1190ADS
125.
Minogue S, Hsuan J (2008) Phosphatidylinositol 4-kinase type IIβ. UCSD-Nature Molecule Pages, UCSD-Nature Signaling Gateway (www.signaling-gateway.org)
126.
Kakuk A, Friedländer E, Vereb G Jr, Kása A, Balla A, Balla T, Heilmeyer LM Jr, Gergely P, Vereb G (2006) Nucleolar localization of phosphatidylinositol 4-kinase PI4K230 in various mammalian cells. Cytometry A 69:1174–1183
127.
Balla A, Tuymetova G, Tsiomenko A, Várnai P, Balla T (2005) A plasma membrane pool of phosphatidylinositol 4-phosphate is generated by phosphatidylinositol 4-kinase type-IIIα: studies with the PH domains of the oxysterol binding protein and FAPP1. Molecular Biology of the Cell 16:1282–1295
128.
Balla A, Kim YJ, Varnai P, Szentpetery Z, Knight Z, Shokat KM, Balla T (2008) Maintenance of hormone-sensitive phosphoinositide pools in the plasma membrane requires phosphatidylinositol 4-kinase IIIα. Molecular Biology of the Cell 19:711–721
129.
Trotard M, Lepère-Douard C, Régeard M, Piquet-Pellorce C, Lavillette D, Cosset FL, Gripon P, Le Seyec J (2009) Kinases required in hepatitis C virus entry and replication highlighted by small interference RNA screening. FASEB Journal
130.
Guerriero CJ, Weixel KM, Bruns JR, Weisz OA (2006) Phosphatidylinositol 5-kinase stimulates apical biosynthetic delivery via an Arp2/3-dependent mechanism. Journal of Biological Chemistry 281:15376–15384
131.
Clarke JH, Emson PC, Irvine RF (2008) Localization of phosphatidylinositol phosphate kinase IIγin kidney to a membrane trafficking compartment within specialized cells of the nephron. American Journal of Physiology – Renal Physiology 295:F1422–F1430
132.
Loijens JC, Anderson RA (2006) Type I phosphatidylinositol-4-phosphate 5-kinases are distinct members of this novel lipid kinase family. Journal of Biological Chemistry 271: 32937–32943
133.
Chao WT, Daquinag AC, Ashcroft F, Kunz J (2010) Type I PIPK-α regulates directed cell migration by modulating Rac1 plasma membrane targeting and activation. Journal of Cell Biology 190:247–262
134.
Nakano-Kobayashi A, Yamazaki M, Unoki T, Hongu T, Murata C, Taguchi R, Katada T, Frohman MA, Yokozeki T, Kanaho Y (2007) Role of activation of PIP5Kγ661 by AP-2 complex in synaptic vesicle endocytosis. EMBO Journal 26:1105–1116
135.
Rahdar M, Inoue T, Meyer T, Zhang J, Vazquez F, Devreotes PN (2009) A phosphorylation-dependent intramolecular interaction regulates the membrane association and activity of the tumor suppressor PTEN. Proceedings of the National Academy of Sciences of the United States of America 106:480–485ADS
136.
Yu J, Zhang SS, Saito K, Williams S, Arimura Y, Ma Y, Ke Y, Baron V, Mercola D, Feng GS, Adamson E, Mustelin T (2009) PTEN regulation by Akt–EGR1–ARF–PTEN axis. EMBO Journal 28:21–33
137.
Song MS, Salmena L, Carracedo A, Egia A, Lo-Coco F, Teruya-Feldstein J, Pandolfi PP (2008) The deubiquitinylation and localization of PTEN are regulated by a HAUSP–PML network. Nature 455:813–817ADS
138.
Sigal YJ, McDermott MI, Morris AJ (2005) Integral membrane lipid phosphatases/ phosphotransferases: common structure and diverse functions.
139.
Begley MJ, Dixon JE (2005) The structure and regulation of myotubularin phosphatases. Current Opinion in Structural Biology 15:614–620
140.
Sasaki J, Kofuji S, Itoh R, Momiyama T, Takayama K, Murakami H, Chida S, Tsuya Y, Takasuga S, Eguchi S, Asanuma K, Horie Y, Miura K, Davies EM, Mitchell C, Yamazaki M, Hirai H, Takenawa T, Suzuki A, Sasaki T (2010) The PtdIns(3,4)P2 phosphatase INPP4A is a suppressor of excitotoxic neuronal death. Natur 465:497–501ADS
141.
Munday AD, Norris FA, Caldwell KK, Brown S, Majerus PW, Mitchell CA (1999) The inositol polyphosphate 4-phosphatase forms a complex with phosphatidylinositol 3-kinase in human platelet cytosol. Proceedings of the National Academy of Sciences of the United States of America 96:3640–3645ADS
142.
Minagawa T, Ijuin T, Mochizuki Y, Takenawa T (2001) Identification and characterization of a sac domain-containing phosphoinositide 5-phosphatase. Journal of Biological Chemistry 276:22011–22015
143.
Symons MH, Chuang Y (2011) Synaptojanin 2. UCSD-Nature Molecule Pages, UCSD-Nature Signaling Gateway (www.signaling-gateway.org)
144.
Nemoto Y, De Camilli P (1999) Recruitment of an alternatively spliced form of synaptojanin 2 to mitochondria by the interaction with the PDZ domain of a mitochondrial outer membrane protein. EMBO Journal 18:2991–3006
145.
Liu Y, Boukhelifa M, Tribble E, Morin-Kensicki E, Uetrecht A, Bear JE, Bankaitis VA (2008) The Sac1 phosphoinositide phosphatase regulates Golgi membrane morphology and mitotic spindle organization in mammals. Molecular Biology of the Cell 19:3080–3096
146.
Jin N, Chow CY, Liu L, Zolov SN, Bronson R, Davisson M, Petersen JL, Zhang Y, Park S, Duex JE, Goldowitz D, Meisler MH, Weisman LS (2008) VAC14 nucleates a protein complex essential for the acute interconversion of PI3P and PI(3,5)P(2) in yeast and mouse. EMBO Journal 27:3221–3234
147.
Rudge SA, Anderson DM, Emr SD (2004) Vacuole size control: regulation of PtdIns(3,5)P2 levels by the vacuole-associated Vac14-Fig4 complex, a PtdIns(3,5)P2-specific phosphatase. Molecular Biology of the Cell 15:24–36
148.
Harris SJ, Parry RV, Westwick J, Ward SG (2008) Phosphoinositide lipid phosphatases: natural regulators of phosphoinositide 3-kinase signaling in T lymphocytes. Journal of Biological Chemistry 283:2465–2469
149.
Halaszovich CR, Schreiber DN, Oliver D (2009) Ci-VSP is a depolarization-activated phosphatidylinositol 4,5-bisphosphate and phosphatidylinositol 3,4,5-trisphosphate 5’-phosphatase. Journal of Biological Chemistry 284:2106–2113
150.
Brindley DN (2004) Lipid phosphate phosphatases and related proteins: Signaling functions in development, cell division, and cancer. Journal of Cellular Biochemistry 92:900–912
151.
Nakanaga K, Hama K, Aoki J (2010) Autotaxin: an LPA producing enzyme with diverse functions. Journal of Biochemistry 148:13–24
152.
Goding JW, Grobben B, Slegers H (2003) Physiological and pathophysiological functions of the ecto-nucleotide pyrophosphatase/phosphodiesterase family. Biochimica et Biophysica Acta 1638:1–19
153.
Ubersax JA, Ferrell JE (2007) Mechanisms of specificity in protein phosphorylation. Nature Reviews – Molecular Cell Biology 8:530–541
154.
Manning G, Whyte DB, Martinez R, Hunter T, Sudarsanam S (2002) The protein kinase complement of the human genome. Science 298:1912–1934 (www.kinase.com/human/kinome)
155.
Manning BD, Cantley LC (2002) Hitting the target: emerging technologies in the search for kinase substrates. Science STKE 2002:pe49
156.
Phylogenetic tree of the human kinome in Signal Transduction Knowledge Environment (www.stke.org and stke.sciencemag.org)
157.
Katoh Y, Takemori H, Horike N, Doi J, Muraoka M, Min L, Okamoto M (2004) Salt-inducible kinase (SIK) isoforms: their involvement in steroidogenesis and adipogenesis. Molecular and Cellular Endocrinology 217:109–112
158.
LaRonde-LeBlanc N, Wlodawer A (2005) The RIO kinases: An atypical protein kinase family required for ribosome biogenesis and cell cycle progression. Biochimica et Biophysica Acta – Proteins and Proteomics 1754:14–24
159.
Mollet J, Delahodde A, Serre V, Chretien D, Schlemmer D, Lombes A, Boddaert N, Desguerre I, de Lonlay P, de Baulny HO, Munnich A, Rötig A (2008) CABC1 gene mutations cause ubiquinone deficiency with cerebellar ataxia and seizures. American Journal of Human Genetics 82:623–630
160.
Macinga DR, Cook GM, Poole RK, Rather PN (1998) Identification and characterization of aarF, a locus required for production of ubiquinone in Providencia stuartii and Escherichia coli and for expression of 2′-N-acetyltransferase in P. stuartii. Journal of Bacteriology 180: 128–135
161.
Sargent CA, Anderson MJ, Hsieh SL, Kendall E, Gomez-Escobar N, Campbell RD (1994) Characterisation of the novel gene G11 lying adjacent to the complement C4A gene in the human major histocompatibility complex. Human Molecular Genetics 3:481–488
162.
Fraser RA, Heard DJ, Adam S, Lavigne AC, Le Douarin B, Tora L, Losson R, Rochette-Egly C, Chambon P (1998) The putative cofactor TIF1α is a protein kinase that is hyperphosphorylated upon interaction with liganded nuclear receptors. Journal of Biological Chemistry 273:16199–16204
163.
Allton K, Jain AK, Herz HM, Tsai WW, Jung SY, Qin J, Bergmann A, Johnson RL, Barton MC (2009) Trim24 targets endogenous p53 for degradation. Proceedings of the National Academy of Sciences of the United States of America 106:11612–11616ADS
164.
Dupont S, Zacchigna L, Cordenonsi M, Soligo S, Adorno M, Rugge M, Piccolo S (2005) Germ-layer specification and control of cell growth by Ectodermin, a Smad4 ubiquitin ligase. Cell 121:87–99
165.
Dupont S, Mamidi A, Cordenonsi M, Montagner M, Zacchigna L, Adorno M, Martello G, Stinchfield MJ, Soligo S, Morsut L, Inui M, Moro S, Modena N, Argenton F, Newfeld SJ, Piccolo S (2009) FAM/USP9x, a deubiquitinating enzyme essential for TGFβ signaling, controls Smad4 monoubiquitination. Cell 136:123–135
166.
He W, Dorn DC, Erdjument-Bromage H, Tempst P, Moore MA, Massagué J (2006) Hematopoiesis controlled by distinct TIF1γ and Smad4 branches of the TGFβ pathway. Cell 125:929–941
167.
Forrest AR, Taylor DF, Crowe ML, Chalk AM, Waddell NJ, Kolle G, Faulkner GJ, Kodzius R, Katayama S, Wells C, Kai C, Kawai J, Carninci P, Hayashizaki Y, Grimmond SM (2006) Genome-wide review of transcriptional complexity in mouse protein kinases and phosphatases. Genome Biology 7:R5
168.
Boudeau J, Miranda-Saavedra D, Barton GJ, Alessi DR (2006) Emerging roles of pseudokinases. Trends in Cell Biology 16:443–452
169.
Lange A, Wickström SA, Jakobson M, Zent R, Sainio K, Fässler R (2009) Integrin-linked kinase is an adaptor with essential functions during mouse development. Nature 461:1002–1006ADS
170.
Dougherty MK, Ritt DA, Zhou M, Specht SI, Monson DM, Veenstra TD, Morrison DK (2009) KSR2 is a calcineurin substrate that promotes ERK cascade activation in response to calcium signals. Molecular Cell 34:652–662
171.
Rajakulendran T, Sicheri F (2010) Allosteric protein kinase regulation by pseudokinases: insights from STRAD. Science Signaling 3:pe8
172.
Baas AF, Boudeau J, Sapkota GP, Smit L, Medema R, Morrice NA, Alessi DR, Clevers HC (2003) Activation of the tumour suppressor kinase LKB1 by the STE20-like pseudokinase STRAD. EMBO Journal 22:3062–3072
173.
Hawley SA, Boudeau J, Reid JL, Mustard KJ, Udd L, Mäkelä TP, Alessi DR, Hardie DG (2003) Complexes between the LKB1 tumor suppressor, STRAD alpha/beta and MO25 alpha/beta are upstream kinases in the AMP-activated protein kinase cascade. Journal of Biology 2:28
174.
Lizcano JM, Göransson O, Toth R, Deak M, Morrice NA, Boudeau J, Hawley SA, Udd L, Mäkelä TP, Hardie DG, Alessi DR (2004) LKB1 is a master kinase that activates 13 kinases of the AMPK subfamily, including MARK/PAR-1. EMBO Journal 23:833–843
175.
Kiss-Toth E, Bagstaff SM, Sung HY, Jozsa V, Dempsey C, Caunt JC, Oxley KM, Wyllie DH, Polgar T, Harte M, O’Neill LA, Qwarnstrom EE, Dower SK (2004) Human tribbles, a protein family controlling mitogen-activated protein kinase cascades. Journal of Biological Chemistry 279:42703–42708
176.
Kiss-Toth E (2007) Trb1. UCSD-Nature Molecule Pages, UCSD-Nature Signaling Gateway (www.signaling-gateway.org)
177.
Hegedus Z, Czibula A, Kiss-Toth E (2006) Tribbles: novel regulators of cell function; evolutionary aspects. Cellular and Molecular Life Sciences 63:1632–1641
178.
Sung HY, Guan H, Czibula A, King AR, Eder K, Heath E, Suvarna SK, Dower SK, Wilson AG, Francis SE, Crossman DC, Kiss-Toth E (2007) Human tribbles-1 controls proliferation and chemotaxis of smooth muscle cells via MAPK signaling pathways. Journal of Biological Chemistry 282:18379–18387
179.
Kiss-Toth E, Docherty LM (2007) Trb2. UCSD-Nature Molecule Pages, UCSD-Nature Signaling Gateway (www.signaling-gateway.org)
180.
Filippakopoulos P, Kofler M, Hantschel O, Gish GD, Grebien F, Salah E, Neudecker P, Kay LE, Turk BE, Superti-Furga G, Pawson T, Knapp S (2008) Structural coupling of SH2-kinase domains links Fes and Abl substrate recognition and kinase activation. Cell 134:793–803
181.
Pendergast AM, Zipfel P (2006) Abl; Arg. UCSD-Nature Molecule Pages, UCSD-Nature Signaling Gateway (www.signaling-gateway.org)
182.
Eiring AM, Harb JG, Neviani P, Garton C, Oaks JJ, Spizzo R, Liu S, Schwind S, Santhanam R, Hickey CJ, Becker H, Chandler JC, Andino R, Cortes J, Hokland P, Huettner CS, Bhatia R, Roy DC, Liebhaber SA, Caligiuri MA, Marcucci G, Garzon R, Croce CM, Calin GA, Perrotti D (2010) miR-328 functions as an RNA decoy to modulate hnRNP E2 regulation of mRNA translation in leukemic blasts. Cell 140:652–665
183.
Wang B, Golemis EA, Kruh GD (1997) ArgBP2, a multiple Src homology 3 domain-containing, Arg/Abl-interacting protein, is phosphorylated in v-Abl-transformed cells and localized in stress fibers and cardiocyte Z-disks. Journal of Biological Chemistry 272: 17542–17550
184.
Galisteo ML, Yang Y, Ureña J, Schlessinger J (2006) Activation of the nonreceptor protein tyrosine kinase Ack by multiple extracellular stimuli. Proceedings of the National Academy of Sciences of the United States of America 103:9796–9801ADS
185.
Satoh T (2005) Ack1. UCSD-Nature Molecule Pages, UCSD-Nature Signaling Gateway (www.signaling-gateway.org)
186.
Crompton MR (2005) Brk. UCSD-Nature Molecule Pages, UCSD-Nature Signaling Gateway (www.signaling-gateway.org)
187.
Qiu H, Zappacosta F, Su W, Annan RS, Miller WT (2005) Interaction between Brk kinase and insulin receptor substrate-4. Oncogene 24:5656–5664
188.
Vang T, Methi T, Veillette A, Mustelin T, Tasken K (2006) Csk. UCSD-Nature Molecule Pages, UCSD-Nature Signaling Gateway (www.signaling-gateway.org)
189.
Zhao M, Janas JA, Niki M, Pandolfi PP, Van Aelst L (2006) Dok-1 independently attenuates Ras/mitogen-activated protein kinase and Src/c-myc pathways to inhibit platelet-derived growth factor-induced mitogenesis. Molecular and Cellular Biology 26:2479–2489
190.
Lemay S, Davidson D, Latour S, Veillette A (2000) Dok-3, a novel adapter molecule involved in the negative regulation of immunoreceptor signaling. Molecular and Cellular Biology 20:2743–2754
191.
Frame MC, Patel H, Serrels B, Lietha D, Eck MJ (2010) The FERM domain: organizing the structure and function of FAK. Nature Reviews – Molecular Cell Biology 11:802–814
192.
Cooper J, Li W, You L, Schiavon G, Pepe-Caprio A, Zhou L, Ishii R, Giovannini M, Hanemann CO, Long SB, Erdjument-Bromage H, Zhou P, Tempst P, Giancotti FG (2011) Merlin/NF2 functions upstream of the nuclear E3 ubiquitin ligase CRL4DCAF1 to suppress oncogenic gene expression. Science Signaling 4:pt6
193.
Koshman YE, Engman SJ, Kim T, Iyengar R, Henderson KK, Samarel AM (2010) Role of FRNK tyrosine phosphorylation in vascular smooth muscle spreading and migration. Cardiovascular Research 85:571–581
194.
Hauck CR, Hsia DA, Schlaepfer DD (2002) The focal adhesion kinase – a regulator of cell migration and invasion. IUBMB Life 53:115–119
195.
Mitra SK, Hanson DA, Schlaepfer DD (2005) Focal adhesion kinase: in command and control of cell motility. Nature Reviews – Molecular Cell Biology 6:56–68
196.
Dalla Costa AP, Clemente CF, Carvalho HF, Carvalheira JB, Nadruz W Jr, Franchini KG (2010) FAK mediates the activation of cardiac fibroblasts induced by mechanical stress through regulation of the mTOR complex. Cardiovascular Research 86:421–431
197.
Seko Y, Takahashi N, Sabe H, Tobe K, Kadowaki T, Nagai R (1999) Hypoxia induces activation and subcellular translocation of focal adhesion kinase (p125(FAK)) in cultured rat cardiac myocytes. Biochemical and Biophysical Research Communications 262:290–296
198.
Pawson T, Letwin K, Lee T, Hao QL, Heisterkamp N, Groffen J (1989) The FER gene is evolutionarily conserved and encodes a widely expressed member of the FPS/FES protein-tyrosine kinase family. Molecular and Cellular Biology 9:5722–5725
199.
Greer P (2002) Closing in on the biological functions of Fps/Fes and Fer. Nature Reviews – Molecular Cell Biology 3:278–289
200.
Itoh T, Hasegawa J, Tsujita K, Kanaho Y, Takenawa T (2009) The tyrosine kinase Fer is a downstream target of the PLD-PA pathway that regulates cell migration. Science Signaling 2:ra52
201.
Kapus A, Di Ciano C, Sun J, Zhan X, Kim L, Wong TW, Rotstein OD (2000) Cell volume-dependent phosphorylation of proteins of the cortical cytoskeleton and cell-cell contact sites. The role of Fyn and FER kinases. Journal of Biological Chemistry 275:32289–32298
202.
Schwartz Y, Ben-Dor I, Navon A, Motro B, Nir U (1998) Tyrosine phosphorylation of the TATA element modulatory factor by the FER nuclear tyrosine kinases. FEBS Letters 434: 339–345
203.
Craven RJ, Cance WG, Liu ET (1995) The nuclear tyrosine kinase Rak associates with the retinoblastoma protein pRb. Cancer Research 55:3969–3972
204.
Annerén C, Lindholm CK, Kriz V, Welsh M (2003) The FRK/RAK-SHB signaling cascade: a versatile signal-transduction pathway that regulates cell survival, differentiation and proliferation. Current Molecular Medicine 3:313–324
205.
Serfas MS, Tyner AL (2003) Brk, Srm, Frk, and Src42A form a distinct family of intracellular Src-like tyrosine kinases. Oncology Research 13:409–419
206.
Yim EK, Peng G, Dai H, Hu R, Li K, Lu Y, Mills GB, Meric-Bernstam F, Hennessy BT, Craven RJ, Lin SY (2009) Rak functions as a tumor suppressor by regulating PTEN protein stability and function. Cancer Cell 15:304–314
207.
Leonard WJ (2001) Cytokines and immunodeficiency diseases. Nature Reviews – Immunology 1:200–208
208.
Kurdi M, Booz GW (2009) JAK redux: a second look at the regulation and role of JAKs in the heart. American Journal of Physiology – Heart and Circulatory Physiology 297: H1545–H1556
209.
Yang J, Stark GR (2008) Roles of unphosphorylated STATs in signaling. Cell Research 18:443–451
210.
Kile BT, Nicola NA, Alexander WS (2001) Negative regulators of cytokine signaling. International Journal of Hematology 73:292–298
211.
Nicholson SE, Willson TA, Farley A, Starr R, Zhang JG, Baca M, Alexander WS, Metcalf D, Hilton DJ, Nicola NA (1999) Mutational analyses of the SOCS proteins suggest a dual domain requirement but distinct mechanisms for inhibition of LIF and IL-6 signal transduction. EMBO Journal 18:375–385
212.
Kinjyo I, Hanada T, Inagaki-Ohara K, Mori H, Aki D, Ohishi M, Yoshida H, Kubo M, Yoshimura A (2002) SOCS1/JAB is a negative regulator of LPS-induced macrophage activation. Immunity 17:583–591
213.
Gingras S, Parganas E, de Pauw A, Ihle JN, Murray PJ (2004) Re-examination of the role of suppressor of cytokine signaling 1 (SOCS1) in the regulation of toll-like receptor signaling. Journal of Biological Chemistry 79:54702–54707
214.
Cohney SJ, Sanden D, Cacalano NA, Yoshimura A, Mui A, Migone TS, Johnston JA (1999) SOCS-3 is tyrosine phosphorylated in response to interleukin-2 and suppresses STAT5 phosphorylation and lymphocyte proliferation. Molecular and Cellular Biology 19: 4980–4988
215.
Croker BA, Krebs DL, Zhang JG, Wormald S, Willson TA, Stanley EG, Robb L, Greenhalgh CJ, Förster I, Clausen BE, Nicola NA, Metcalf D, Hilton DJ, Roberts AW, Alexander WS (2003) SOCS3 negatively regulates IL-6 signaling in vivo. Nature – Immunology 4:540–545
216.
Ueki K, Kondo T, Kahn CR (2004) Suppressor of cytokine signaling 1 (SOCS-1) and SOCS-3 cause insulin resistance through inhibition of tyrosine phosphorylation of insulin receptor substrate proteins by discrete mechanisms. Molecular and Cellular Biology 24:5434–5446
217.
Mooney RA, Senn J, Cameron S, Inamdar N, Boivin LM, Shang Y, Furlanetto RW (2001) Suppressors of cytokine signaling-1 and -6 associate with and inhibit the insulin receptor. A potential mechanism for cytokine-mediated insulin resistance. Journal of Biological Chemistry 276:25889–25893
218.
Cheng HC, Chong YP, Ia KK, Tan O, Mulhern TD (2006) Csk homologous kinase. UCSD-Nature Molecule Pages, UCSD-Nature Signaling Gateway (www.signaling-gateway.org)
219.
Jhun BH, Rivnay B, Price D, Avraham H (1995) The MATK tyrosine kinase interacts in a specific and SH2-dependent manner with c-Kit. Journal of Biological Chemistry 270: 9661–9666
220.
Yamashita H, Avraham S, Jiang S, Dikic I, Avraham H (1999) The Csk homologous kinase associates with TrkA receptors and is involved in neurite outgrowth of PC12 cells. Journal of Biological Chemistry 274:15059–15065
221.
Kohmura N, Yagi T, Tomooka Y, Oyanagi M, Kominami R, Takeda N, Chiba J, Ikawa Y, Aizawa S (1994) A novel nonreceptor tyrosine kinase, Srm: cloning and targeted disruption. Molecular and Cellular Biology 14:6915–6925
222.
Mano H, Yamashita Y, Miyazato A, Miura Y, Ozawa K (1996) Tec protein-tyrosine kinase is an effector molecule of Lyn protein-tyrosine kinase. FASEB Journal 10:637-642.
223.
Sandilands E, Cans C, Fincham VJ, Brunton VG, Mellor H, Prendergast GC, Norman JC, Superti-Furga G, Frame MC (2004) RhoB and actin polymerization coordinate Src activation with endosome-mediated delivery to the membrane. Developmental Cell 7:855–869
224.
Knock GA, Snetkov VA, Shaifta Y, Drndarski S, Ward JPT, Aaronson PI (2008) Role of src-family kinases in hypoxic vasoconstriction of rat pulmonary artery. Cardiovascular Research 80:453–462
225.
Seko Y, Tobe K, Takahashi N, Kaburagi Y, Kadowaki T, Yazaki Y (1996) Hypoxia and hypoxia/reoxygenation activate Src family tyrosine kinases and p21ras in cultured rat cardiac myocytes. Biochemical and Biophysical Research Communications 226:530–535
226.
Yin H, Chao L, Chao J (2005) Kallikrein/kinin protects against myocardial apoptosis after ischemia/reperfusion via Akt-glycogen synthase kinase-3 and Akt-Bad.14-3-3 signaling pathways. Journal of Biological Chemistry 280:8022–8030
227.
Sato H, Sato M, Kanai H, Uchiyama T, Iso T, Ohyama Y, Sakamoto H, Tamura J, Nagai R, Kurabayashi M (2005) Mitochondrial reactive oxygen species and c-Src play a critical role in hypoxic response in vascular smooth muscle cells. Cardiovascular Research 67:714–722
228.
Pleiman CM, Clark MR, Gauen LK, Winitz S, Coggeshall KM, Johnson GL, Shaw AS, Cambier JC (1993) Mapping of sites on the Src family protein tyrosine kinases p55blk, p59fyn, and p56lyn which interact with the effector molecules phospholipase C-γ2, microtubule-associated protein kinase, GTPase-activating protein, and phosphatidylinositol 3-kinase. Molecular and Cellular Biology 13:5877–5887
229.
Hegde R, Srinivasula SM, Ahmad M, Fernandes-Alnemri T, Alnemri ES (1998) Blk, a BH3-containing mouse protein that interacts with Bcl-2 and Bcl-xL, is a potent death agonist. Journal of Biological Chemistry 273:7783–7786
230.
Dymecki SM, Niederhuber JE, Desiderio SV (1990) Specific expression of a tyrosine kinase gene, blk, in B lymphoid cells. Science 247:332–336ADS
231.
Lin YH, Shin EJ, Campbell MJ, Niederhuber JE (1995) Transcription of the blk gene in human B lymphocytes is controlled by two promoters. Journal of Biological Chemistry 270:25968–25975
232.
Bagheri-Yarmand R, Mandal M, Taludker AH, Wang RA, Vadlamudi RK, Kung HJ, Kumar R (2001) Etk/Bmx tyrosine kinase activates Pak1 and regulates tumorigenicity of breast cancer cells. Journal of Biological Chemistry 276:29403–29409
233.
Mitchell-Jordan SA, Holopainen T, Ren S, Wang S, Warburton S, Zhang MJ, Alitalo K, Wang Y, Vondriska TM (2008) Loss of Bmx nonreceptor tyrosine kinase prevents pressure overload-induced cardiac hypertrophy. Circulation Research 103:1359–1362
234.
Pan S, An P, Zhang R, He X, Yin G, Min W (2002) Etk/Bmx as a tumor necrosis factor receptor type 2-specific kinase: role in endothelial cell migration and angiogenesis. Molecular and Cellular Biology 22:7512–7523
235.
Waldeck-Weiermair M, Zoratti C, Osibow K, Balenga N, Goessnitzer E, Waldhoer M, Malli R, Graier WF (2008) Integrin clustering enables anandamide-induced Ca2 + signaling in endothelial cells via GPR55 by protection against CB1-receptor-triggered repression. Journal of Cell Science 121:1704–1717
236.
Semaan N, Alsaleh G, Gottenberg JE, Wachsmann D, Sibilia J (2008) Etk/BMX, a Btk family tyrosine kinase, and Mal contribute to the cross-talk between MyD88 and FAK pathways. Journal of Immunology 180:3485–3491
237.
Palmer CD, Mutch BE, Workman S, McDaid JP, Horwood NJ, Foxwell BM (2008) Bmx tyrosine kinase regulates TLR4-induced IL-6 production in human macrophages independently of p38 MAPK and NFκB activity. Blood 111:1781–1788
238.
Carpenter CL (2004) Btk-dependent regulation of phosphoinositide synthesis. Biochemical Society Transactions 32:326–329
239.
Uckun FM (2008) Bruton’s tyrosine kinase (BTK) as a dual-function regulator of apoptosis. Biochemical Pharmacology 56:683–691
240.
Lowell CA, Berton G (1999) Integrin signal transduction in myeloid leukocytes. Journal of Leukocyte Biology 65:313–320
241.
Resh M (2006) Fyn. UCSD-Nature Molecule Pages, UCSD-Nature Signaling Gateway (www.signaling-gateway.org)
242.
Banin S, Truong O, Katz DR, Waterfield MD, Brickell PM, Gout I (1996) Wiskott-Aldrich syndrome protein (WASp) is a binding partner for c-Src family protein-tyrosine kinases. Current Biology 6:981–988
243.
Stanglmaier M, Warmuth M, Kleinlein I, Reis S, Hallek M (2003) The interaction of the Bcr-Abl tyrosine kinase with the Src kinase Hck is mediated by multiple binding domains. Leukemia 17:283–289
244.
Scott MP, Zappacosta F, Kim EY, Annan RS, Miller WT (2002) Identification of novel SH3 domain ligands for the Src family kinase Hck. Wiskott-Aldrich syndrome protein (WASP), WASP-interacting protein (WIP), and ELMO1. Journal of Biological Chemistry 277: 28238–28246
245.
Briggs SD, Bryant SS, Jove R, Sanderson SD, Smithgall TE (1995) The Ras GTPase-activating protein (GAP) is an SH3 domain-binding protein and substrate for the Src-related tyrosine kinase, Hck. Journal of Biological Chemistry 270:14718–14724
246.
Shivakrupa R, Radha V, Sudhakar Ch, Swarup G (2003) Physical and functional interaction between Hck tyrosine kinase and guanine nucleotide exchange factor C3G results in apoptosis, which is independent of C3G catalytic domain. Journal of Biological Chemistry 278:52188–52194
247.
Ward AC, Monkhouse JL, Csar XF, Touw IP, Bello PA (1998) The Src-like tyrosine kinase Hck is activated by granulocyte colony-stimulating factor (G-CSF) and docks to the activated G-CSF receptor. Biochemical and Biophysical Research Communications 251:117–123
248.
Poghosyan Z, Robbins SM, Houslay MD, Webster A, Murphy G, Edwards DR (2002) Phosphorylation-dependent interactions between ADAM15 cytoplasmic domain and Src family protein-tyrosine kinases. Journal of Biological Chemistry 277:4999-5007
249.
Hao S, August A (2002) The proline rich region of the Tec homology domain of ITK regulates its activity. FEBS Letters 525:53–58
250.
Bunnell SC, Henry PA, Kolluri R, Kirchhausen T, Rickles RJ, Berg LJ (1996) Identification of Itk/Tsk Src homology 3 domain ligands. Journal of Biological Chemistry 271:25646–25656
251.
BioGRID: General Repository for Interaction Datasets; database of physical and genetic interactions for model organisms (www.thebiogrid.org)
252.
Bléry M, Kubagawa H, Chen CC, Vély F, Cooper MD, Vivier E (1998) The paired Ig-like receptor PIR-B is an inhibitory receptor that recruits the protein-tyrosine phosphatase SHP-1. Proceedings of the National Academy of Sciences of the United States of America 95: 2446–2451ADS
253.
Belsches AP, Haskell MD, Parsons SJ (1997) Role of c-Src tyrosine kinase in EGF-induced mitogenesis. Frontiers in Bioscience 2:d501–d518
254.
Amanchy R, Zhong J, Hong R, Kim JH, Gucek M, Cole RN, Molina H, Pandey A (2009) Identification of c-Src tyrosine kinase substrates in platelet-derived growth factor receptor signaling. Molecular Oncology 3:439–450
255.
Goldman TL, Du Y, Buttrick PM, Walker LA (2009) Src kinase expression, phosphorylation and activation in human and bovine left ventricles. FASEB Journal 23:524.7
256.
Yang WC, Ghiotto M, Castellano R, Collette Y, Auphan N, Nunès JA, Olive D (2000) Role of Tec kinase in nuclear factor of activated T cells signaling. International Immunology 12: 1547–1552
257.
Kane LP, Watkins SC (2005) Dynamic regulation of Tec kinase localization in membrane-proximal vesicles of a T cell clone revealed by total internal reflection fluorescence and confocal microscopy. Journal of Biological Chemistry 280:21949–21954
258.
Felices M, Falk M, Kosaka Y, Berg LJ (2007) Tec kinases in T cell and mast cell signaling. Advances in Immunology 93:145–184
259.
Smith CI, Islam TC, Mattsson PT, Mohamed AJ, Nore BF, Vihinen M (2001) The Tec family of cytoplasmic tyrosine kinases: mammalian Btk, Bmx, Itk, Tec, Txk and homologs in other species. Bioessays 23:436–446
260.
Yang WC, Collette Y, Nunès JA, Olive D (2000) Tec kinases: a family with multiple roles in immunity. Immunity 12:373–382
261.
Rajagopal K, Sommers CL, Decker DC, Mitchell EO, Korthauer U, Sperling AI, Kozak CA, Love PE, Bluestone JA (1999) RIBP, a novel Rlk/Txk- and itk-binding adaptor protein that regulates T cell activation. Journal of Experimental Medicine 190:1657–1668
262.
Maruyama T, Nara K, Yoshikawa H, Suzuki N (2006) Txk, a member of the non-receptor tyrosine kinase of the Tec family, forms a complex with poly(ADP-ribose) polymerase 1 and elongation factor 1α and regulates interferon-γ gene transcription in Th1 cells. Clinical and Experimental Immunology 147:164–175
263.
Chen YH, Lu Q, Goodenough DA, Jeansonne B (2002) Nonreceptor tyrosine kinase c-Yes interacts with occludin during tight junction formation in canine kidney epithelial cells. Molecular Biology of the Cell 13:1227–1237
264.
Mócsai A, Ruland J, Tybulewicz VLJ (2010) The SYK tyrosine kinase: a crucial player in diverse biological functions. Nature Reviews – Immunology 10:387–402
265.
Geahlen RL (2007) Syk. UCSD-Nature Molecule Pages, UCSD-Nature Signaling Gateway (www.signaling-gateway.org)
266.
Tohyama Y, Yamamura H (2009) Protein tyrosine kinase, Syk: a key player in phagocytic cells. Journal of Biochemistry 145:267–273
267.
Kyriakis JM, Avruch J (2001) Mammalian mitogen-activated protein kinase signal transduction pathways activated by stress and inflammation. Physiological Reviews 81:807–869
268.
Chaar Z, O’Reilly P, Gelman I, Sabourin LA (2006) v-Src-dependent down-regulation of the Ste20-like kinase SLK by casein kinase II. Journal of Biological Chemistry 281:28193–28199
269.
Sabourin LA, Tamai K, Seale P, Wagner J, Rudnicki MA (2000) Caspase 3 cleavage of the Ste20-related kinase SLK releases and activates an apoptosis-inducing kinase domain and an actin-disassembling region. Molecular and Cellular Biology 20:684–696
270.
Oehrl W, Kardinal C, Ruf S, Adermann K, Groffen J, Feng GS, Blenis J, Tan TH, Feller SM (1998) The germinal center kinase (GCK)-related protein kinases HPK1 and KHS are candidates for highly selective signal transducers of Crk family adapter proteins. Oncogene 17:1893–1901
271.
Kyriakis JM (1999) Signaling by the germinal center kinase family of protein kinases. Journal of Biological Chemistry 274:5259–5262
272.
Chen YR, Tan TH (1999) Mammalian c-Jun N-terminal kinase pathway and STE20-related kinases. Gene Therapy and Molecular Biology 4:83–98
273.
Kiefer F, Arnold R (2010) Hpk1. UCSD-Nature Molecule Pages, UCSD-Nature Signaling Gateway (www.signaling-gateway.org)
274.
Ensenat D, Yao Z, Wang XS, Kori R, Zhou G, Lee SC, Tan TH (1999) A novel src homology 3 domain-containing adaptor protein, HIP-55, that interacts with hematopoietic progenitor kinase 1. Journal of Biological Chemistry 274:33945–33950
275.
Han J, Kori R, Shui JW, Chen YR, Yao Z, Tan TH (2003) The SH3 domain-containing adaptor HIP-55 mediates c-Jun N-terminal kinase activation in T cell receptor signaling. Journal of Biological Chemistry 278:52195–52202
276.
Liu SK, Fang N, Koretzky GA, McGlade CJ (1999) The hematopoietic-specific adaptor protein gads functions in T-cell signaling via interactions with the SLP-76 and LAT adaptors. Current Biology 9:67–75
277.
Zhou G, Boomer JS, Tan TH (2004) Protein phosphatase 4 is a positive regulator of hematopoietic progenitor kinase 1. Journal of Biological Chemistry 279:49551–49561
278.
Findlay GM, Yan L, Procter J, Mieulet V, Lamb RF (2007) A MAP4 kinase related to Ste20 is a nutrient-sensitive regulator of mTOR signalling. Biochemical Journal 403:13–20
279.
Chernoff J (2008) Mst1; Mst2. UCSD-Nature Molecule Pages, UCSD-Nature Signaling Gateway (www.signaling-gateway.org)
280.
Braun H, Suske G (1998) Combinatorial action of HNF3 and Sp family transcription factors in the activation of the rabbit uteroglobin/CC10 promoter. Journal of Biological Chemistry 273:9821–9828
281.
Glantschnig H, Rodan GA, Reszka AA (2002) Mapping of MST1 kinase sites of phosphorylation. Activation and autophosphorylation. Journal of Biological Chemistry 277:42987–42996
282.
Nakano K, Kanai-Azuma M, Kanai Y, Moriyama K, Yazaki K, Hayashi Y, Kitamura N (2003) Cofilin phosphorylation and actin polymerization by NRK/NESK, a member of the germinal center kinase family. Experimental Cell Research 287:219–227
283.
Fu CA, Shen M, Huang BC, Lasaga J, Payan DG, Luo Y (1999) TNIK, a novel member of the germinal center kinase family that activates the c-Jun N-terminal kinase pathway and regulates the cytoskeleton. Journal of Biological Chemistry 274:30729–30737
284.
Kuramochi S, Moriguchi T, Kuida K, Endo J, Semba K, Nishida E, Karasuyama H (1997) LOK is a novel mouse STE20-like protein kinase that is expressed predominantly in lymphocytes. Journal of Biological Chemistry 272:22679–22684
285.
Walter SA, Cutler RE Jr, Martinez R, Gishizky M, Hill RJ (2003) Stk10, a new member of the polo-like kinase kinase family highly expressed in hematopoietic tissue. Journal of Biological Chemistry 278:18221–18228
286.
Choe KP, Strange K (2010) OXSR1. UCSD-Nature Molecule Pages, UCSD-Nature Signaling Gateway (www.signaling-gateway.org)
287.
Lee SJ, Cobb MH, Goldsmith EJ (2009) Crystal structure of domain-swapped STE20 OSR1 kinase domain. Protein Science 18:304–313
288.
Mudumana SP, Hentschel D, Liu Y, Vasilyev A, Drummond IA (2008) Odd skipped related 1 reveals a novel role for endoderm in regulating kidney versus vascular cell fate. Development 135:3355–3367
289.
Strange K, Denton J, Nehrke K (2006) Ste20-type kinases: evolutionarily conserved regulators of ion transport and cell volume. Physiology 21:61–68
290.
Russell JM (2000) Sodium–potassium–chloride cotransport. Physiological Reviews 80: 211–276
291.
Anselmo AN, Earnest S, Chen W, Juang YC, Kim SC, Zhao Y, Cobb MH (2006) WNK1 and OSR1 regulate the Na + , K + , 2Cl − cotransporter in HeLa cells. Proceedings of the National Academy of Sciences of the United States of America 103:10883–10888ADS
292.
Ko B, Hoover RS (2009) Molecular physiology of the thiazide-sensitive sodium-chloride cotransporter. Current Opinion in Nephrology and Hypertension 18:421–427
293.
Komaba S, Inoue A, Maruta S, Hosoya H, Ikebe M (2003) Determination of human myosin III as a motor protein having a protein kinase activity. Journal of Biological Chemistry 278:21352–21360
294.
Dosé AC, Burnside B (2002) A class III myosin expressed in the retina is a potential candidate for Bardet-Biedl syndrome. Genomics 79:621–624
295.
Hutchison M, Berman KS, Cobb MH (1998) Isolation of TAO1, a protein kinase that activates MEKs in stress-activated protein kinase cascades. Journal of Biological Chemistry 273:28625–28632
296.
Zihni C, Mitsopoulos C, Tavares IA, Ridley AJ, Morris JD (2006) Prostate-derived sterile 20-like kinase 2 (PSK2) regulates apoptotic morphology via C-Jun N-terminal kinase and Rho kinase-1. Journal of Biological Chemistry 281:7317–7323
297.
Chen Z, Cobb MH (2001) Regulation of stress-responsive mitogen-activated protein (MAP) kinase pathways by TAO2. Journal of Biological Chemistry 276:16070–16075
298.
Tassi E, Biesova Z, Di Fiore PP, Gutkind JS, Wong WT (1999) Human JIK, a novel member of the STE20 kinase family that inhibits JNK and is negatively regulated by epidermal growth factor. Journal of Biological Chemistry 274:33287–33295
299.
Hergovich A, Stegert MR, Schmitz D, Hemmings BA (2006) NDR kinases regulate essential cell processes from yeast to humans. Nature Reviews – Molecular Cell Biology 7:253–264
300.
Pearce LR, Komander D, Alessi DR (2010) The nuts and bolts of AGC protein kinases. Nature Reviews – Molecular Cell Biology 11:9–22
301.
Okuzumi T, Fiedler D, Zhang C, Gray DC, Aizenstein B, Hoffman R, Shokat KM (2009) Inhibitor hijacking of Akt activation. Nature – Chemical Biology 5:484–493
302.
Cameron AJM, Escribano C, Saurin AT, Kostelecky B, Parker PJ (2009) PKC maturation is promoted by nucleotide pocket occupation independently of intrinsic kinase activity. Nature – Structural and Molecular Biology 16:624–630
303.
Kohler RS, Schmitz D, Cornils H, Hemmings BA, Hergovich A (2010) Differential NDR/LATS interactions with the human MOB family reveal a negative role for human MOB2 in the regulation of human NDR kinases. Molecular and Cellular Biology 30:4507–4520
304.
Stokoe D (2007) Pdk1. UCSD-Nature Molecule Pages, UCSD-Nature Signaling Gateway (www.signaling-gateway.org)
305.
Masters TA, Calleja V, Armoogum DA, Marsh RJ, Applebee CJ, Laguerre M, Bain AJ, Larijani B (2010) Regulation of 3-phosphoinositide-dependent protein kinase 1 activity by homodimerization in live cells. Science Signaling 3:ra78
306.
Ito K, Akazawa H, Tamagawa M, Furukawa K, Ogawa W, Yasuda N, Kudo Y, Liao CH, Yamamoto R, Sato T, Molkentin JD, Kasuga M, Noda T, Nakaya H, Komuro I (2009) PDK1 coordinates survival pathways and β-adrenergic response in the heart. Proceedings of the National Academy of Sciences of the United States of America 106:8689–8694ADS
307.
Lignitto L, Carlucci A, Sepe M, Stefan E, Cuomo O, Nisticò R, Scorziello A, Savoia C, Garbi C, Annunziato L, Feliciello A (2011) Control of PKA stability and signalling by the RING ligase praja2. Nature – Cell Biology 13:412–422
308.
Masterson LR, Mascioni A, Traaseth NJ, Taylor SS, Veglia G (2008) Allosteric cooperativity in protein kinase A. Proceedings of the National Academy of Sciences of the United States of America 105:506–511ADS
309.
Wu J, Brown SHJ, von Daake S, Taylor SS (2007) PKA type IIα holoenzyme reveals a combinatorial strategy for isoform diversity. Science 318:274–279ADS
310.
Goel M, Zuo CD, Schilling WP (2010) Role of cAMP/PKA signaling cascade in vasopressin-induced trafficking of TRPC3 channels in principal cells of the collecting duct. American Journal of Physiology – Renal Physiology 298:F988–F996
311.
Butterworth MB, Frizzell RA, Johnson JP, Peters KW, Edinger RS (2005) PKA-dependent ENaC trafficking requires the SNARE-binding protein complexin. American Journal of Physiology – Renal Physiology 289:F969–F977
312.
Nejsum LN, Zelenina M, Aperia A, Frøkiaer J, Nielsen S (2005) Bidirectional regulation of AQP2 trafficking and recycling: involvement of AQP2-S256 phosphorylation. American Journal of Physiology – Renal Physiology 288:F930–F938
313.
Faul C, Dhume A, Schecter AD, Mundel P (2007) Protein kinase A, Ca2 + /calmodulin-dependent kinase II, and calcineurin regulate the intracellular trafficking of myopodin between the Z-disc and the nucleus of cardiac myocytes. Molecular and Cellular Biology 27:8215–8227
314.
Hallaq H, Yang Z, Viswanathan PC, Fukuda K, Shen W, Wang DW, Wells KS, Zhou J, Yi J, Murray KT (2006) Quantitation of protein kinase A-mediated trafficking of cardiac sodium channels in living cells. Cardiovascular Research 72:250–261
315.
Lin L, Sun W, Wikenheiser AM, Kung F, Hoffman DA (2010) KChIP4a regulates Kv4.2 channel trafficking through PKA phosphorylation. Molecular and Cellular Neurosciences 43:315–325
316.
Esteban JA , Shi SH, Wilson C, Nuriya M, Huganir RL, Malinow R (2003) PKA phosphorylation of AMPA receptor subunits controls synaptic trafficking underlying plasticity. Nature – Neuroscience 6:136–143
317.
Parvathenani LK, Buescher ES, Chacon-Cruz E, Beebe SJ (1998) Type I cAMP-dependent protein kinase delays apoptosis in human neutrophils at a site upstream of caspase-3. Journal of Biological Chemistry 273:6736–6743
318.
Prinz A, Herberg FW (2009) Prkx. UCSD-Nature Molecule Pages, UCSD-Nature Signaling Gateway (www.signaling-gateway.org)
319.
Semizarov D, Glesne D, Laouar A, Schiebel K, Huberman E (1998) A lineage-specific protein kinase crucial for myeloid maturation. Proceedings of the National Academy of Sciences of the United States of America 95:15412-15417ADS
320.
Lasserre R, Guo XJ, Conchonaud F, Hamon Y, Hawchar O, Bernard AM, Soudja SM, Lenne PF, Rigneault H, Olive D, Bismuth G, Nunès JA, Payrastre B, Marguet D, He HT (2008) Raft nanodomains contribute to Akt/PKB plasma membrane recruitment and activation. Nature – Chemical Biology 4:538–547
321.
Gonzalez E, McGraw TE (2009) Insulin-modulated Akt subcellular localization determines Akt isoform-specific signaling. Proceedings of the National Academy of Sciences of the United States of America 106:7004–7009ADS
322.
Mîinea CP, Sano H, Kane S, Sano E, Fukuda M, Peränen J, Lane WS, Lienhard GE (2005) AS160, the Akt substrate regulating GLUT4 translocation, has a functional Rab GTPase-activating protein domain. Biochemical Journal 391:87–93
323.
Xie X, Zhang D, Zhao B, Lu MK, You M, Condorelli G, Wang CY, Guan KL (2011) IκB kinase ε and TANK-binding kinase 1 activate AKT by direct phosphorylation. Proceedings of the National Academy of Sciences of the United States of America 108:6474–6479ADS
324.
Miyamoto S, Rubio M, Sussman mA (2009) Nuclear and mitochondrial signalling Akts in cardiomyocytes. Cardiovascular Research 82:272–285
325.
Kim HE, Du F, Fang M, Wang X (2005) Formation of apoptosome is initiated by cytochrome c-induced dATP hydrolysis and subsequent nucleotide exchange on Apaf-1. Proceedings of the National Academy of Sciences of the United States of America 102:17545–17550ADS
326.
Yoeli-Lerner M, Yiu GK, Rabinovitz I, Erhardt P, Jauliac S, Toker A (2005) Akt blocks breast cancer cell motility and invasion through the transcription factor NFAT. Molecular Cell 20:539–550
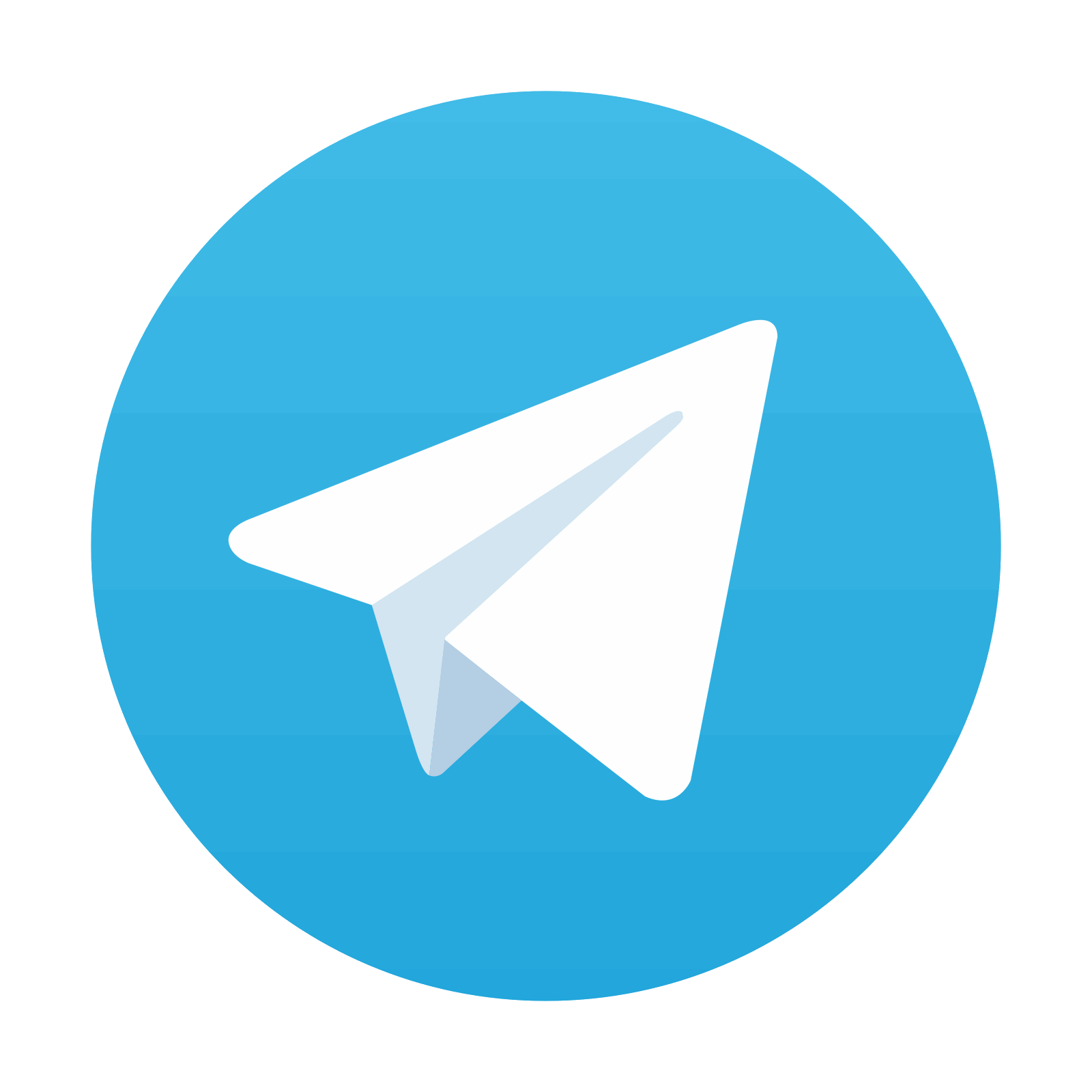
Stay updated, free articles. Join our Telegram channel

Full access? Get Clinical Tree
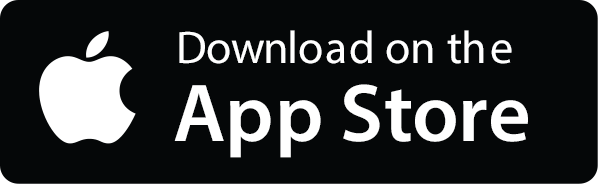
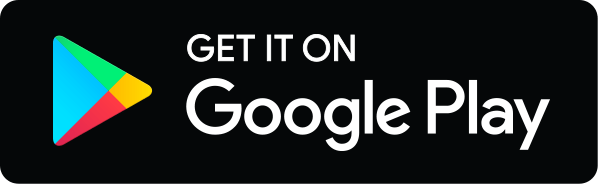
