(1)
Project-team INRIA-UPMC-CNRS REO Laboratoire Jacques-Louis Lions, CNRS UMR 7598, Université Pierre et Marie Curie, Place Jussieu 4, Paris Cedex 05, France
Abstract
Numerous protein serine/threonine kinases operate in signal transduction, either as initiating nodes, such as receptor (RSTK; Vol. 3 – Chap. 8. Receptor Kinases), or as intermediate nodes of signaling cascades, non-receptor (intracellular; NRSTK) protein Ser/Thr kinases. Typically, second messengers activate protein Ser/Thr kinases, whereas extracellular signals stimulate protein Tyr kinases (Chap. 4). For example, IκB kinases (IKK) of IκB inhibitors of nuclear factor-κB are serine kinases (Sect. 10.9.1).
Numerous protein serine/threonine kinases operate in signal transduction, either as initiating nodes, such as receptor (RSTK; Vol. 3 – Chap. 8. Receptor Kinases), or as intermediate nodes of signaling cascades, non-receptor (intracellular; NRSTK) protein Ser/Thr kinases. Typically, second messengers activate protein Ser/Thr kinases, whereas extracellular signals stimulate protein Tyr kinases (Chap. 4). For example, IκB kinases (IKK) of IκB inhibitors of nuclear factor-κB are serine kinases (Sect. 10.9.1).
Non-receptor protein Ser/Thr kinases include numerous kinases, such as cAMP-dependent protein kinase-A (PKA), protein kinase-B (PKB or Akt), protein kinase-C (PKC), cGMP-dependent protein kinase-G (PKG), calmodulin-dependent kinases, protein kinase-D (PKD) RoCK kinases, P90 (RSK) and P70 (S6K) ribosomal S6-kinases, P21-activating kinase, Bcr proteins, etc. Certain enzymes have been considered to be Ser/Thr kinases despite their dual specificity, such as mitogen-activated protein kinases and glycogen synthase kinase-3.1
5.1 Superfamily of Ser/Thr Sterile-20-Related Kinases
The superfamily of Sterile-20-like kinases includes many kinases that are related to Ser/Thr kinase Ste20 in Saccharomyces cerevisiae. Among these kinases, several are mitogen-activated protein kinase kinase kinase kinases (MAP4Ks) and some mitogen-activated protein kinase kinase kinases (MAP3Ks; Chap. 6). Many Ste20-like family kinases actually function as regulators of MAPK cascades. Therefore, Ste20-like kinases regulate multiple cellular processes, such as cell cycle, growth, differentiation, apoptosis, and stress responses. In addition, certain Ste20-like kinases contribute to cellular volume sensing.
The Ste20-like kinase superfamily is subdivided into 2 structurally and functionally distinct families (Table 5.1): (1) family of P21-activated kinases (PAK; Sect. 5.2.13) that are regulated by small GTPases Rac1 and CDC42 (Sect. 9.3) and (2) family of germinal center kinases (GCK).2
Table 5.1
The Ste20-like kinase superfamily and its P21-activated kinase (PAK) and germinal center kinase (CGK) families (Source: [251, 267]; CRK: avian sarcoma virus CT10 oncogene homolog (chicken tumor virus regulator of kinase); CRKL: CRK-like adaptor; GLK: GCK-like kinase; GRB: growth factor receptor-bound protein; GCKR: GCK-related kinase; HGK: HPK1/GCK-related kinase; HPK: hematopoietic progenitor kinase; MinK: Misshapen-like kinase; MAP3K: mitogen-activated protein kinase kinase kinase; NCK: non-catalytic region of tyrosine kinase adaptor; NIK: NCK-interacting kinase; cRaf: v-Raf-1 murine leukemia viral oncogene homolog; TRAF: tumor-necrosis factor-receptor associated factor).
Name | Other | Substrates | Adaptors |
---|---|---|---|
Alias(es) | Effectors | Coactivators | |
Affectors | |||
GCK | MAP4K2 | MAP3K1, MAP2K4 | TRAF2 |
Rab8A | |||
GCKR | MAP4K5, KHS | MAP3K1 | CRK, CRKL, TRAF2 |
GLK | MAP4K3 | ||
HPK1 | MAP4K1 | MAP3K7, MAP3K11 | CRK, CRKL, GRB2, |
EGFR, PP4 | GrAP2, NCK1, Dbnl, | ||
BLnk, LAT, HCls1 | |||
NIK | MAP4K4, HGK | MAP3K1, MAP3K7 | NCK1, GBP3, RasA1, |
Integrin-β1 | BrCa1 | ||
MinK1 | NCK1 | ||
PAK1 | PAKα | cRaf | NCK1/2 |
HER2, CDC2L2, CDK5 | PAK1IP1 | ||
BMX, LIMK1, MLCK | IRSp53 | ||
Abl3, PKB | |||
CDC42, Rac1, RhoJ | RhoGEF2/7 | ||
Dncl1, ArpC1B | |||
PAK2 | PAKγ | Abl1/3, | SH3KBP1, APAP1/2 |
CDC42, Rac1/2, | DOCK2 | ||
MLC2, MHC10 | |||
MCM3 | |||
PAK3 | PAKβ | cRaf | |
Rac1 | RhoGEF7 |
Members of the PAK family contains a C-terminal catalytic domain and an N-terminal binding site CRIB (CDC42–Rac1 interactive binding domain) for small GTPases Rac1 and CDC42. The PAK kinases (Sect. 5.2.13) indeed activate small GTPases Rac and CDC42 to regulate cytoskeletal organization and cell motility. They are also implicated in the activation of the JNK pathways.
On the other hand, GCK family members have an N-terminal kinase domain and a C-terminal regulatory region (without CRIB consensus sequence). Many GCK kinases activate Jun N-terminal kinases or P38MAPKs. Others are activated under some stress conditions or by apoptotic agents.
Certain kinases phosphorylate ion transporters. Furthermore, many of these kinases contribute to numerous pathways excited by the transforming growth factor-β and integrins activated by EPH receptors. They are also involved in lymphocyte aggregation, as they regulate cell clustering via αLβ2-integrin.3 They participate in T-cell receptor signaling.
The set of dual-specificity mitogen-activated protein kinases includes 3 families associated with ERK, JNK, and P38MAPK kinases. Whereas extracellular signal-regulated kinases are activated in response to ligand binding to cognate receptor Tyr kinases or G-protein-coupled receptors via Ras GTPases, JNKs are stimulated in response to cell stresses, such as osmotic and heat shock, ionizing electromagnetic waves, oxidative stress, protein synthesis inhibitors, and pro-inflammatory cytokines. On the other hand, P38MAPKs also respond to pro-inflammatory agents (e.g., interleukin-1, tumor-necrosis factor-α, lipopolysaccharides) and environmental stress (osmotic shock). Both JNKs and P38MAPKs that are targeted by many GCKs are excited by ischemia–reperfusion events and hypertension as well as humoral factors, such as angiotensin-2 and endothelin. Therefore, JNKs and P38MAPKs are poorly activated by mitogens, but potently by environmental stresses. Members of the JNK and P38MAPK subfamilies stimulate several transcription factors, especially Activator protein-1.
Activatory kinases of JNKs are MAP2K4 and MAP2K7, whereas P38MAPK activators are MAP2K3 and MAP2K6. One signaling cascade tier upstream from MAP2Ks, MAP3K1, MAP3K10, and MAP3K11 are specific stimulators of the JNK pathway. In addition, members of the RHO superfamily of the RAS hyperfamily (CDC42 and Rac1) can activate the JNK and P38MAPK cascades, the mediators of which contain a CDC42–Rac-interacting and -binding (CRIB) domain, such as MAP3K1, MAP3K4, MAP3K10, and MAP3K11.
5.1.1 Ste20-like Kinase
Ste20-like kinase (SLK), or STK2, is a microtubule-associated protein that provokes actin stress fiber disassembly. Kinase SLK redistributes with vinculin and colocalizes with CK2 in structures reminiscent of membrane lamellipodia and ruffles during cell spreading. Kinase Src activates casein kinase CK2 that phosphorylates SLK (Ser347 and Ser348) to reduce SLK activity [268].
Kinase SLK mediates apoptosis and actin stress fiber degradation via distinct domains released after caspase-3 cleavage [269]. Activated N-terminal kinase domain promotes apoptosis and cytoskeletal rearrangement, whereas C-terminus disassembles actin stress fibers. Kinase SLK interacts with C-terminal linking and modulating protein (CLAMP) that is also designated as PDZ domain-containing protein PDZK1, or sodium–hydrogen exchanger member-3 regulator NHERF3 [251].
5.1.2 Germinal Center Kinase Family
Many GCK family members, such as MAP4K1 to MAP4K5, activate the JNK pathway. Adaptors are crucial for signal transduction in cells because they mediate rapid, selective formation of multiproteic complexes. Adaptors of the CRK family are composed of Src homology domains SH2 and SH3 to specifically tether phosphorylated tyrosyl- and proline-rich sequence-containing proteins, respectively [270]. Adaptors CRK and CRKL connect guanine nucleotide-exchange factors C3G (Rap1a/b GEF) and dedicator of cytokinesis DOCK1, as well as EGFR pathway substrate Eps15. Adaptors CRK and CRKL also interact with other small adaptors NCK and GRB2, Abl family kinases (Sect. 4.2), SOS1 and SOS2 RasGEFs. Adaptors CRK and CRKL thus intervene in integrin signaling, B-cell receptor activation, and JNK activation.
Among GCK family members, MAP4K1 and MAP4K5 are signaling partners of CRK family adaptors (Table 5.2). Whereas MAP4K5 binds exclusively to CRK family proteins, MAP4K1 associates with both GRB2 and NCK (weakly) [270]. Kinase MAP4K4 interacts with NCK adaptor to link protein Tyr kinase to JNK. Enzymes MAP4K2 and MAP4K5 mediate TNF-induced JNK activation via TRAF2 TNFR-associated factor.
Table 5.2
The CGK kinases and MAPK modules in the JNK pathway (Source: [272]). The MAPK tiered modules encompass MAP4Ks of the group-1 GCKs, MAP3Ks, MAP2Ks, and MAPKs. The MAPK effector set targeted by MAP4Ks are JNK kinases. Group-1 GCKs represented by PAK isoforms are also able to activate JNKs. The activation of the JNK pathway is mediated by adaptors, small GTPases, and TNF receptor-associated factors (TRAF).
Stimuli | Mitogens | Cytokines | Cellular stress |
---|---|---|---|
Adaptors | CRK, CRKL | TRAF | CDC42, Rab8, Rac1, |
GRB2, NCK | Ras, Rho | ||
Tier 1 | MAP4Ks/CGKs and PAKs | ||
Tier 2 | MAP3Ks | ||
Tier 3 | MAP2Ks | ||
Tier 4 | MAPKs – JNKs |
The GCK family can be subdivided into 2 groups based on structural and functional properties [271]. Group-1 GCKs include hematopoietic progenitor kinase HPK1 (or MAP4K1), germinal center kinase (GCK or MAP4K2), GCK-like kinase (GLK or MAP4K3), GCK-related kinase (GCKR or MAP4K5), and NCK-interacting kinase (NIK or MAP4K4)4 Their C-termini possess 2 PEST (proline–glutamic acid–serine–threonine) motifs and at least 2 polyproline consensus binding sites for SH3-containing proteins as well as a hydrophobic, leucine-rich sequence and a C-terminal (CT) region. These enzymes activate selectively SAPKs/JNKs.
Group-2 GCKs comprise Ste20-like oxidant stress-activated kinase SOK1 (or STK25);5 mammalian sterile-20 (twenty)-like kinases MST1 (STK4 or kinase responsive to stress KRS2), MST2 (KRS1 or STK3), and MST3 (STK24); and lymphocyte-oriented kinase (LOK or STK10). These enzymes do not activate any MAPK pathways. Members of the group-2 GCKs — STK3 and STK4 — can be activated by both phosphorylation and dephosphorylation, as they are stimulated by PP2 phosphatase.
The GCK family can be also broken into a higher number of subgroups according to structure and biochemical properties [272]. The HPK1/GCK (MAP4K1–MAP4K2) and NIK (MAP4K4) subgroups are constituted by MAP4K enzymes. The MST/SOK subgroup includes mammalian Ste20-like proteins MST1 to MST3, SOK1, and proline–alanine-rich Ste20-related kinase (PASK). Other branches corresponds to LOK and SLK on the one hand and PSK on the other.
Analyses on human kinome led to the reclassification of the GCK family members into 8 phylogenetically distinct subfamilies. The GCK subfamily 1 comprises MAP4K1 to MAP4K3 and MAP4K5 enzymes. The GCK subfamily 2 encompasses STK3 (KSR1 or MST2) and STK4 (KSR2 or MST1) that are activated during apoptosis. The GCK subfamily 3 consists of 3 mammalian kinases, STK24, STK25 (SOK1 or YSK1), and MST3- and SOK1-related kinase (MASK or MST4). Unlike other GCK family kinases, neither STK24 nor STK25 activate the JNK or P38MAPK pathways. They are stimulated by oxidant stress and hypoxia. However, they do not participate in apoptosis. The GCK subfamily 4 is composed of MAP4K4 (NIK), MAP4K6 (MinK), NRK, and TNIK enzymes. The GCK subfamily 5 is constituted by SLK and STK10 (LOC). The GCK subfamily 6 includes OxSR1 that targets both JNK and P38MAPK and STK39 (PASK, SPAK, or DCHT) that only activates P38MAPK. The GCK subfamily 7 contains unconventional subclass-3 myosins Myo3a and Myo3b that have a kinase domain N-terminal to the conserved N-terminal motor domains. They are expressed in photoreceptors. The GCK subfamily 8 is made up of TAOK1 (PSK2 or KFCb), TAOK2 (PSK1, MAP3K17, or KFCc), and TAOK3 (JIK or KFCa).
Most identified GCK family kinases activate either JNK and/or P38MAPK signaling cascades. The GCK subfamily-3 kinases STK24, STK25, and MASK, GCK subfamily-5 STK10, and GCK subfamily-6 OxSR1 do not stimulate these pathways. Four GCK family kinases, GCK subfamily-1 MAP4K1, GCK subfamily-2 STK3 and STK4, and GCK subfamily-5 SLK directly or indirectly contribute to apoptosis.
5.1.3 MAP4Ks of GCK Subfamilies 1 and 4 of the Ste20-like Kinase Superfamily
The MAP4K1/GCK (HPK1/GCK) subfamily of mammalian Ste20-like kinases, or GCK subfamily 1, includes MAP4K1 to MAP4K3 and MAP4K5, whereas MAP4K4 and MAP4K6 belong to the GCK subfamily 4 (Table 5.3).
Table 5.3
Mitogen-activated protein kinase kinase kinase kinases (MAP4K) of GCK subfamilies 1 and 4 of the Ste20-like kinase superfamily (GCK: germinal center kinase; GCKR: GCK-related kinase; GLK: GCK-like kinase; HGK: HPK1/GCK-related kinase; HPK: hepatocyte progenitor kinase; HPK1: hematopoietic progenitor kinase; KHS: kinase homologous to Ste20; MinK: Misshapen-like kinase; NESK: NIK-like embryo-specific kinase; NIK: non-catalytic region of tyrosine kinase adaptor (NCK)-interacting kinase [not NFκB-inducing kinase, i.e., MAP3K14]; NRK: NIK-related kinase; Rab8IP: Rab8-interacting protein; Rab8IPL: Rab8-interacting protein-like protein; TNIK: TRAF2 and NCK-interacting kinase).
Member | Aliases |
---|---|
GCK subfamily 1 | |
MAP4K1 | HPK1 |
MAP4K2 | GCK, Rab8IP |
MAP4K3 | GLK, Rab8IPL1 |
MAP4K5 | GCKR, KHS1 |
GCK subfamily 4 | |
MAP4K4 | HPK, HGK, NIK |
MAP4K6 | MinK |
NRK | NESK |
TNIK |
5.1.3.1 MAP4K1
Mitogen-activated protein kinase kinase kinase kinase MAP4K1 is also termed hematopoietic progenitor kinase HPK1 as, in mammals, it is a hematopoietic cell-specific Ste20-like protein kinase. It is characterized by an N-terminal kinase domain, an intermediate region, and a C-terminal Citron-homology domain. It is a potent and highly specific activator of Jun N-terminal kinases in various cell types [273].
Enzyme MAP4K1 is involved in various signaling pathways initiated by receptors of epidermal growth factor, transforming growth factor-β, erythropoietin, and prostaglandin-E2, as well as B- (BCR) and T-cell (TCR) receptors. In lymphocytes, MAP4K1 activity rises in response to TCR or BCR crosslinking [273].
Activation of MAP4K1 depends on autophosphorylation (Thr165) and phosphorylation by protein kinase-D (Ser171) [273]. Kinase MAP4K1 may also be activated by Ser/Thr protein phosphatase-4 [277] (Sect. 8.3.4). Furthermore, PP4-induced activation is accompanied by an increase in MAP4K1 expression. Phosphatase PP4 increases the MAP4K1 half-life. Activity of MAP4K1 is also regulated by ubiquitination followed by degradation. Phosphatase PP4 precludes MAP4K1 ubiquitination. Stimulation by TCRs enhances PP4–MAP4K1 interaction.
In T lymphocytes and myeloid cells, MAP4K1 activity is stimulated after exposure to immunosuppressive PGE2 prostaglandin [273]. In T lymphocytes, MAP4K1 impedes PGE2-induced transcription of the FOS gene. Conversely, PGE2 causes HPK1 activation via the cAMP–PKA axis.
Kinase MAP4K1 can stop T-cell activation and provoke T-cell apoptosis [273]. It is also implicated in monocyte differentiation.
Protein MAP4K1 interacts with many proteins: MAP3K7,6 MAP3K11,7 EGFR, HCLS1,8 BLnk,9 CLnk,10 DbnL,11 NCK1, GRB2, CRK, CRKL,12 GRAP2,13 LCP214 LAT15 and DAPP116 (Table 5.1) [251, 273].
Furthermore, MAP4K1 contributes to the activation of NFκB transcription factors. Full length MAP4K1 interacts with IKKβ in T lymphocytes to activate NFκB upon TCR stimulation, using its substrate and adaptor caspase activation and recruitment domain (CARD)-containing protein family CARD11, or CARMA1 [273].
5.1.3.2 MAP4K2
Mitogen-activated protein kinase kinase kinase kinase MAP4K2 is also identified as germinal center kinase GCK as well as BL44, Rab8IP, as it is an interacting protein of small GTPase Rab8. It is detected in many tissues. However, its expression in lymphoid follicles is restricted to the cells of the germinal center, where it can participate in B-lymphocyte differentiation. It can be activated by TNFα. It interacts with TNF receptor-associated factor TRAF2 to activate MAP3K1.
5.1.3.3 MAP4K3
Mitogen-activated protein kinase kinase kinase kinase MAP4K3 is also tagged GLK and Rab8IPL1. Activity of MAP4K3 is regulated by amino acids, but not insulin. It is required to activate P90 ribosomal S6 kinase and for maximal phosphorylation of eukaryotic initiation factor 4E-binding protein 4E-BP1 [278].
5.1.3.4 MAP4K4
Mitogen-activated protein kinase kinase kinase kinase MAP4K417 specifically activate MAPK8 (JNK1) via the MAP3K1–MAP2K4 and MAP3K7–MAP2K7 cascades. It interacts also with GTPase activating protein RasA1 guanylate-binding protein GBP3, adaptor NCKα (or NCK1), β1-integrin, and breast cancer early onset protein BrCa1 that operates in DNA-damage repair, ubiquitination, and transcriptional regulation [251].18
5.1.3.5 MAP4K5
Mitogen-activated protein kinase kinase kinase kinase MAP4K5 is similar to Ste20 kinase, hence its aliases GCKR (GCK-related kinase) and KHS (or KHS1: kinase homologous to Ste20). It activates Jun N-terminal kinases in the framework of stress response. Two alternatively spliced transcript variants encode the same protein. It interacts with CRK, CRKL, and TRAF2 (Table 5.1).
5.1.3.6 MAP4K6 – Misshapen-like kinase
Mitogen-activated protein kinase kinase kinase kinase MAP4K6, or protein Ser/Thr Misshapen-like kinase MinK1,19 activates JNK and P38MAPK kinases. It interacts with the adaptor Non-catalytic region of Tyr kinase NCK1 to transmit signals from receptor Tyr kinases, such as EGFR, PDGFR-B, and EPHb1.
5.1.4 Kinases of GCK Subfamilies 2 and 3 – MST Kinases
Subfamily-2 GCKs are MST1, or STK4, and MST2, or STK3, whereas subfamily-3 GCKs correspond to MST3, or STK24, and MST4 (Table 5.4).
Table 5.4
Members of GCK subfamilies 2, 3, and 5 of the Ste20-like kinase superfamily (KSR: kinase suppressor of Ras; LOK: lymphocyte-oriented kinase; MASK: MST3- and SOK1-related kinase; MST: mammalian Ste20-like protein kinase; SLK: Ste20-like kinase; SOK: Ste20-like oxidant stress-activated kinase; STK: Ser/Thr kinase).
Member | Aliases |
---|---|
GCK subfamily 2 | |
STK3 | KSR1, MST2 |
STK4 | KSR2, MST1 |
GCK subfamily 3 | |
STK24 | MST3 |
STK25 | SOK1 |
MASK | MST4 |
GCK subfamily 5 | |
STK2 | SLK |
STK10 | LOK |
5.1.4.1 STK3 (MST2)
Protein Ser/Thr kinase STK320 possesses a regulatory C-terminus that modulates its kinase activity. It contains at least one caspase-3 cleavage site. In response to apoptotic stimuli, STK3 is indeed cleaved by caspase-3 to release the activated N-terminal kinase domain that is much more active and stimulates P38MAPK and JNK kinases [279]. Moreover, full-length STK3 is highly susceptible to dephosphorylation with respect to caspase-cleaved form. Activity of STK3 is enhanced by autophosphorylation following homodimerization.
Targets of STK3 comprise Large tumor suppressors LaTS1 and LaTS2 kinases ( Hippo pathway; Sect. 10.7), connector enhancer of kinase suppressor of Ras CnKSR1, Ras association (RalGDS/AF6) domain-containing protein family members RASSF1 and RASSF2, Salvador homolog Sav1 (or WW45), thyroid transcription factor TTF1, and forkhead box FoxA (or hepatocyte nuclear factor HNF3) [251, 279]. Transcription factor TTF1 is required for thyroid-specific production of thyroglobulin and thyroperoxidase.
Respiratory epithelium-specific transcription is also mediated by thyroid transcription factor TTF1 and transcription factors of the FoxA subfamily. These transcription factors are needed for lung-specific synthesis of surfactant protein-A, -B, and -C, and small, secreted, dimeric secretoglobin-1A (Scgb1A1).21 The SCGB1A1 promoter is regulated by FoxA1 and FoxA2, but neither FoxJ1 nor TTF1 [280].
Activity of STK3 is regulated by phosphorylation, proteolysis, and other types of between-protein interactions. Kinase STK3 is activated by stressors, such as heat shock, toxin and phosphatase okadaic acid and anti-fungal, anti-hypertensive staurosporine. Kinase cRaf binds to STK3 and prevents dimerization and autophosphorylation.
5.1.4.2 STK4 (MST1)
Protein Ser/Thr kinase STK422 is widely expressed. This member of the Sterile-20 superfamily of cytoskeletal, stress-activated, and apoptotic kinases is stimulated by STK4K kinase that phosphorylates Thr residues (Thr183 and Thr187). Homodimerization enhances its activation via autophosphorylation (Thr177 and Thr387) [281]. Full-length STK4 (STK4FL) shuttles between the nucleus and cytosol.
It is activated by cellular stressors to activate the stress-induced mitogen-activated protein kinase cascade. It provokes chromatin condensation, as it phosphorylates histone-2B (Ser14). It also causes apoptosis, as it is activated upon engagement of the pro-apoptotic receptor TNFRSF6 of the TNFR superfamily. In response to apoptotic stimuli, STK4 is cleaved by caspase-3 (AsP326) to release the N-terminal kinase domain that is much more active. Cleaved STK436 localizes mostly to the nucleus.
Owing to the SARAH domain, it interacts with itself and other proteins, such as Ras association (RalGDS/AF6) domain family members RASSF1A and RASSF5 that associates with DNA-repair protein XPA and activated Ras, Rap1, and Ras-like small GTPases, respectively, and Salvador homolog Sav1 that interacts with 14-3-3-σ (stratifin) [279]. Other STK4 Substrates include Large tumor suppressor LaTS1 kinase that forms a complex with CDK1 in early mitosis, connector enhancer of kinase suppressor of Ras CnKSR1, and protein kinase interferon-inducible double stranded RNA-dependent inhibitor (PRKRIR or death-associated protein DAP4). Moreover, STK4 phosphorylates transcription factor FoxO3 (Ser207) to cause its dissociation from 14-3-3 protein.
5.1.4.3 STK24 (MST3)
Protein Ser/Thr kinase STK2423 belongs to the GCK family (GCK subfamily 3) of Sterile-20-like kinases or SPS1 group. It participates in the response to cellular stress. The proteolytic cleavage of its C-terminus that possesses nuclear localization and export signals provokes nuclear translocation of its kinase domain.
5.1.4.4 MASK (MST4)
Ubiquitous protein Ser/Thr kinase MST424 belongs to the GCK subfamily 3. It contains an N-terminal kinase domain and a C-terminal regulatory domain that mediates homodimerization and prevents its kinase activity. It is located in the Golgi body, where it is specifically activated by binding to the Golgi matrix protein GM130. It is cleaved by caspase-3 to generate a C-terminally truncated form. Several alternatively spliced transcript variants exist. Both full-length MASK and its C-terminally truncated form can induce apoptosis.
5.1.5 STK25 of the GCK Subfamily-3
In addition to STK24 (MST3) and MASK1 (MST4), the GCK subfamily 3 comprises STK25 kinase.25 It interacts with STK24, PP2, apoptosis-related protein-15, or programmed cell death protein PdCD10, striatins Strn, Strn3, and Strn4, suppressor of IKKε, T-complex protein-1, and sarcolemma-associated protein [251].
5.1.6 Other Kinases of the GCK Subfamily 4
The GCK subfamily 4 is composed of MAP4K4 (Sect. 5.1.3.4), or non-catalytic region of Tyr kinase (NCK)-interacting kinase (NIK), and MAP4K6 (Misshapen-like kinase [MinK]; Sect. 5.1.3.6)) as well as NIK-related kinase (NRK) and TRAF2 and NCK-interacting kinase (TNIK).
5.1.6.1 NIK-Related Kinase (NRK)
5.1.6.2 TRAF2 and NCK-Interacting Kinase (TNIK)
TRAF2 (TNF receptor-associated factor-2) and NCK (non-catalytic region of Tyr kinase adaptor)-interacting kinase (TNIK) is characterized by an N-terminal kinase domain and a C-terminal GCK domain that serves as a regulator [283]. In addition to activating the JNK pathway, TNIK causes disruption of Factin structures. Kinase TNIK is able to phosphorylate gelsolin. It also interacts with Na + –H + exchanger SLC9a1 (or NHE1) and TRAF2 [251].
5.1.7 Kinases of the GCK Subfamily 5
The GCK subfamily 5 contains STK10, or lymphocyte-oriented kinase (LOK), and SLK (Sect. 5.1.1). Kinase STK10 is expressed predominantly in lymphoid organs, such as the spleen, thymus, and bone marrow, i.e., lymphocytes. Its non-catalytic domain does not exhibit any similarity to that of other known members of the subfamily. It possesses a proline-rich motif with SH3 domain-binding potential followed by a long coiled-coil structure at the C-terminus [284]. Nevertheless, a close sequence homology exists with SLK of the same subfamily that serves as a Polo-like kinase kinase (PL2K).
Kinase STK10 actually phosphorylates Polo-like kinase PLK1. It then cooperates with SLK to regulate PLK1 activity in cells [285]. Kinase STK10 phosphorylates itself as well as substrates, such as myelin basic protein and histone-2A, but does not target any MAPK (ERK, JNK, or P38MAPK). In T lymphocytes, STK10 prevents interleukin-2 expression via the MAP2K1 pathway.
5.1.8 Kinases of the GCK Subfamily 6
Members of the germinal center kinase subfamily-6 comprise oxidative stress-responsive kinase OxSR1 as well as protein Ser/Thr kinases STK37 and STK39 that are involved in the regulation of ion homeostasis and volume control in mammalian cells as well as extracellular ion and water balance, hence the maintenance of blood pressure (Table 5.5). They indeed activate ubiquitous Na + –K + –2Cl − cotransporter NKCC1 in addition to P38MAPK enzymes.
Table 5.5
Members of subfamilies 6, 7, and 8 of the Ste20-like kinase family (DPK: dendritic cell-derived protein kinase; JIK: Jun N-terminal kinase (JNK)-inhibitory kinase; KFC: kinase from chicken; Myo: myosin; OxSR1, OSR1: oxidative stress-responsive kinase; PASK: PAS domain-containing Ser/Thr kinase; PSK: prostate-derived Sterile-20-like kinase; SPAK: Ste20-related proline/alanine-rich kinase; STK: Ser/Thr kinase; TAOK: thousand and one amino acid protein kinase).
Member | Aliases |
---|---|
GCK subfamily 6 | |
OxSR1 | OSR1 |
STK37 | PASK, paskin |
STK39 | SPAK |
GCK subfamily 7 | |
Myo3a | |
Myo3b | |
GCK subfamily 8 | |
MAP3K16 | TAOK1, KFCb, PSK2, MAR2K |
MAP3K17 | TAOK2, KFCb, PSK1, |
MAP3K18 | TAOK3, KFCa, JIK, DPK |
In fact, OxSR1 and STK39 interact with different members of the SLC12A family: KCC3 (or SLC12a6), NKCC1 (or SLC12a2), NKCC2 (or SLC12a1), and NCC (or SLC12a3) [286].27
5.1.8.1 Regulation of Activity
Both OxSR1 and STK39 are phosphorylated (activated) by with-no-lysine protein Ser/Thr kinase WNK1 and WNK4 [287]. In addition to their similar kinase domains, these kinases share 2 conserved C-terminal PF1 and PF2 domains. The PF1 domain controls the catalytic activity, whereas the PF2 domain is responsible for between-protein interactions.
5.1.8.2 OxSR1
Oxidative stress-responsive kinase OxSR1, or OSR1,28 is widely expressed with high levels in the heart and skeletal muscle. Kinase OSR1 was named for its similarity to Ste20-like oxidant stress response kinase STK25 (or SOK1) of the GCK subfamily 3 (without a functional link to oxidative stress). It is sensitive to osmotic stress.
Oxidative stress-responsive kinase-1 phosphorylates PAK1 and impedes its responsiveness to CDC42. It also phosphorylates TNFRSF19L, a tumor-necrosis factor receptor (TNFR) superfamily member,29 which operates in T-cell proliferation [286].
Both OxSR1 and STK39 phosphorylate (activate) Na + –K + –2Cl − cotransporter-1 (or SLC12a2) and Na + –Cl − cotransporter, or SLC12a3, in response to changes in cell volume or intracellular ion concentrations [286].
5.1.8.3 STK37 (PASK)
5.1.8.4 STK39 (SPAK)
Kinase STK3932 has a tissue distribution that overlaps that of OxSR1. It indeed abounds in neurons and epithelia specialized in transport.
Kinase STK39 interacts with kinases P38MAPKα (MAPK14), STK39, WNK4, and apoptosis-associated Tyr kinase (AATK), as well as gelsolin, myelin basic protein, otoferlin, Na + –K + –2Cl − cotransporter SLC12a2, and heat shock protein HSPH1 (HSP105 or HSP110) [251, 286]. The redundant STK39 and OxSR1 kinases regulates shrinkage-induced activation of ubiquitous Na + –K + –2Cl − cotransporter SLC12a2 [286].
Kinases of the GCK Subfamily 6 and Salt and Water Balance
Ste20-like protein kinases of the GCK subfamily 6, oxidative stress-responsive kinase OxSR1 and STK39 bind to volume-sensitive Na + –K + –2 Cl − cotransporters NKCC1 and NKCC2 of the solute carrier SLC12 subclass (Vol. 3 – Chaps. 2. Membrane Ion Carriers and 4. Membrane Compound Carriers). Kinase STK37 regulates the activity not only of Na + –Ka + –2Cl − , but also K + –Cl − cotransporters (KCC). These 2 types of cotransporters intervene in epithelial transport, intra- and extracellular ion homeostasis in the central nervous system, and systemic salt and water balance, as well as cell-volume regulation [289] (Table 5.6).
Table 5.6
Some properties of cation–Cl − cotransporters: N + –K + –2Cl − (NKCC), K + –Cl − (KCC), and Na + –Cl − (NCC) cotransporters (Source: [290]; DCT: distal convoluted tubule; RBC: red blood cell; TAL: thick ascending limb of Henle’s loop).
Property | NKCC | KCC | NCC |
---|---|---|---|
Cl − flux | Influx | Efflux | Influx |
direction | |||
ATP | Stimulation | Inhibition | |
Tissue | NKCC1: | KCC1: | NCC1: |
distribution | Kidney, heart, | Brain, heart, | Kidney (DCT), |
lung, brain, etc. | kidney, liver, | bladder | |
NKCC2: | lung, etc. | ||
Kidney (TAL) | KCC2: | ||
Brain | |||
Cell | RBC, smooth, | RBC, neuron, | |
distribution | skeletal, cardiac | epithelial cell | Renal cell |
myocytes, | |||
fibroblasts, | |||
epithelial cell | |||
(respiratory) | |||
Intracellular Cl − | Inhibition | Stimulation | |
Activation | WNK1/4 | WNK1/4 | WNK1/4 |
OxSR1, STK37/39 | STK37 | OxSR1, STK39 |
The regulatory volume decrease that results from swelling provokes activation of KCC cotransporters to cause KCl efflux and osmotically associated water motion. Conversely, regulatory volume increase that follows cell shrinkage is mainly elicited by activated NKCC cotransporters that generate uptake of NaCl and KCl. Activation of KCCs and NKCCs is conferred by dephosphorylation and phosphorylation by volume-sensitive kinases and phosphatases, respectively. Phosphorylation of NKCCs and KCCs provokes their activation and inactivation, respectively, whereas dephosphorylation of these cotransporters precludes NKCC and activates KCC.
Kinase STK37 cooperates with OSR1 to control the activity of NKCCs and KCCs. They are themselves regulated by P38MAPK, apoptosis-associated Tyr kinase (AaTyK; Vol. 3 – Chap. 8. Receptor Kinases) and with no lysine (K) family kinase WNK4. The latter works with STK37 to regulate NKCC1 and KCC2 [289]. Isozymes WNK1 and WNK4 actually phosphorylate (activate) STK37 and OSR1 that can then phosphorylate NKCC1.
Kinase WNK1 also regulates the functioning of OSR1, STK39, and NKCC [291]. Enzyme WNK1 phosphorylates (activates) both OSR1 and STK39 kinases. Activated OSR1 and STK39 phosphorylate (activate) NKCC1 and NKCC2 cotransporters.33
Cell-volume sensor modulates concentration of osmolyte glycerol. Glycerol accumulates by increased glycerol synthesis and reduction in glycerol efflux. Glycerol synthesis is partly regulated by Ste20-related kinases such as STK37. Cell shrinkage and swelling induce conformational changes in cytoskeletal and/or proteic sensors that can then activate and inactivate STK37, respectively.
Both OxSR1 and STK39 are activated by with-no-lysine kinases WNK1 and, in turn, phosphorylate (activate) thiazide diuretic-sensitive sodium–chloride cotransporter (NCC) [292]. This sodium-chloride symporter of the SLC12 subclass removes Na + and Cl − from the distal convoluted tubule of the kidney. Mutations of Wnk1 and Wnk4 genes give rise to NCC hyperactivity, increased renal salt reabsorption, and familial hyperkalemic hypertension (pseudohypoaldosteronism type 2). Kinase WNK3 also activates NCC, but antagonizes WNK4 that directly suppresses NCC activity. Calcium- and DAG-regulated Ras guanine nucleotide-releasing protein RasGRP1 primes the MAPK cascade to activate extracellular signal-related kinases ERK1 and ERK2 and regulate NCC carrier. Angiotensin-2 and aldosterone also contribute to the regulation of the WNK4–STK39–NCC pathway.
Epithelial Transport
Certain epithelia, such as the respiratory epithelium and nephron coating that produce periciliary fluid and mucus layers for airway clearance and control blood volume and ion concentrations, respectively, transport fluid and solutes. Epithelial transport requires balance between fluxes across apical and basolateral cell membranes. In respiratory conduits, this material transport is mediated by activated basolateral NKCC1s and apical CFTRs.
Cell Volume and Division Cycle
Cell cycle progression can be linked to changes in cell volume. Therefore, volume-sensitive anion channels and cation–chloride cotransporters can contribute to cell cycle regulation. Inhibition of activity of volume-regulated anion channel (VRAC) causes G0–G1 arrest [289]. Conversely, activated VRAC causes cell shrinkage, an element of cell apoptosis. Ste20-like kinases could operate as a scaffold to recruit signaling mediator and coordinate the activity of volume-sensitive anion channels and cation–Cl − cotransporters with cell cycle events.
5.1.9 Kinases of the GCK Subfamily 7
Kinases of the GCK subfamily-7 include actin-dependent, non-processive nanomotors, the unconventional myosin-3A and -3B. These myosins have a kinase region N-terminal to the conserved N-terminal motor domain. They can undergo autophosphorylation in the C-terminal end of the motor domain that activates the kinase activity, without influencing the ATPase activity [293]. Moreover, human myosin-3A and -3B display an actin-translocating activity.
The MYO3A gene expression is highly restricted, with the strongest level in the retina and cochlea. Myosin-3A interacts with the glutamate receptor ionotropic Nmethyl Daspartate-like protein-1A, or GRINL1A complex gene locus product [251]. Isoform Myo3b is a shorter subclass-3 myosin that is expressed in the kidney, retina, and testis. Myosin-3B has several splice variants containing either one or two calmodulin binding (IQ) motifs in the neck domain and one of 3 predominant tail variations: a short tail ending just past the second IQ motif or 2 alternatively spliced longer tails [294].
5.1.10 Kinases of the GCK Subfamily 8
Members of this kinase set include rat Thousand and one amino acid protein kinase (TAO1) and kinase from chicken (KFCb). In humans, these kinases are preferentially referred to as TAOK1, TAOK2, and TAOK3 [251]. However, the straightforward names are mitogen-activated protein kinase kinase kinases MAP3K16, MAP3K17, and MAP3K18 (Sect. 6.5). Kinases of the GCK subfamily 8 have many similarities, such as a N-terminal kinase domain, a serine-rich region, and a coiled-coil configuration within the C-terminus. All subfamily-8 kinases are able to activate the P38MAPK pathway via the specific activation of MAP2K3, in addition to MAP2K6.
Whereas TAOK3 is ubiquitous with high levels in hematopoietic cells such as peripheral blood leukocytes and related tissues (thymus and spleen) and significantly in some organs (prostate, testes, and pancreas; at moderate to low level elsewhere), concentrations of TAOK1 and TAOK2 are small in peripheral blood leukocytes. Concentration of TAOK1 is high in testes and significant in skeletal muscle and placenta, but undetectable in the liver, kidney, and ovary. Enzyme TAOK2 is ubiquitous with high levels in the brain and testes.
5.1.10.1 Prostate-Derived Sterile-20-like Kinases
Human prostate-derived sterile 20-like kinase PSK1 influences actin cytoskeletal organization and binds to microtubules to regulate their arrangement and stability. On the other hand, PSK2 lacks a microtubule-binding site. It activates Jun N-terminal kinase and induces apoptotic morphological changes (cell contraction, membrane blebbing with caspases, and apoptotic body formation). The PSK isoforms are members of the GCK subfamily-8.34
5.1.10.2 Thousand and One Amino Acid Kinase TAOK1 (MAP3K16)
The Ste20-related, GCK-like, protein Ser/Thr kinase Thousand and one kinase TAOK1 (or MAP3K16)35 binds and regulates microtubules. It is highly expressed in the brain as is the other TAOK family member MAP3K17. It activates MAP2K3, MAP2K4, and MAP2K6 in vitro, but only MAP2K3 in cells [295].
Apoptotic stimuli increase the catalytic activity of MAP3K16 and JNK kinases. The latter is activated by MAP3K16 enzyme. Kinase MAP3K16 regulates apoptotic morphology that involves dynamic reorganization of the actin cytoskeleton via its downstream targets JNK and RoCK1 [296]. Protein MAP3K16 indeed stimulates the cleavage of RoCK1 kinase. Activity of RoCK1 is required for MAP3K16 to cause cell contraction and membrane blebbing. Enzyme MAP3K16 is itself a substrate for caspase-3.
5.1.10.3 Thousand and One Amino Acid Kinase TAOK2 (MAP3K17)
5.1.10.4 Thousand and One Amino Acid Kinase TAOK3 (MAP3K18)
The Ste20-related, GCK-like, protein Ser/Thr kinase Thousand and one kinase TAOK3 (or MAP3K18)37 Instead of stimulating the JNK pathway, MAP3K18 hampers the basal activity of JNK. Its inhibition of JNK signaling appears between EGFR and small GTPases Rac1 and CDC42 [298]. Kinase MAP3K18 thus demonstrates that a Ser/Thr kinase can be inhibited by activated RTKs such as EGFR, as it impedes the JNK pathway.38 Kinase MAP3K18 also interacts with endoplasmic reticulum to nucleus signaling protein ERN1 and TNF receptor-associated factor TRAF2 [251].
5.2 AGC Superfamily
The AGC superfamily of protein Ser/Thr kinases stands for protein kinases-A, -G, and -C. Members of the AGC superfamily display strong homology to PKA, PKC, and PKG kinases. About 70 among the 518 protein kinases encoded by the human genome are members of the AGC superfamily. All share a conserved catalytic kinase domain.
Members of the AGC superfamily are regulated by various mechanisms, but most require phosphorylation and conformational changes to be activated. In addition to the kinase domain, other functional regions allow regulation of the activity and localization of AGC kinases. Activation of many AGC kinases involves phosphorylation of 2 regulatory motifs: the activation segment in the catalytic domain and hydrophobic motif in a non-catalytic region. Several AGC kinases also contain another phosphorylation site that promotes their integrity, the turn motif. Some substrates are phosphorylated by a single AGC kinase, others by multiple AGC kinases.
The superfamily of AGC kinases includes cAMP-dependent protein kinase-A, protein kinases-B, several types of protein kinase-C, cGMP-dependent protein kinase-G, ribosomal S6-kinases S6K (P70RSK) and RSK (P90RSK), mitogen- and stress-activated protein kinases (MSK), serum- and glucocorticoid-induced protein kinase (SGK), 3-phosphoinositide-dependent kinase PDK1, and G-protein-coupled receptor kinases (GRK) [299], as well as nuclear Dbf2-related kinase (NDR or STK38), large tumor suppressors LaTS1 and LaTS2 of the Hippo pathway, members of Rho-activated kinases, such as Rho-associated coiled-coil-containing protein kinase (RoCK), citron Rho-interacting kinase (CRIK), myotonic dystrophy-associated protein kinase (DMPK), and CDC42-binding protein kinase-α(CDC42BPα)39 (Tables 5.7 to 5.9) [300].
Table 5.7
Kinases of the AGC superfamily and selected substrates (Part 1; Source: [300]; ATF: activating transcription factor; CREB: cAMP responsive element-binding protein; DMPK: myotonic dystrophy-associated protein kinase; GRK: G-protein-coupled receptor kinase; LaTS, large tumor suppressor; LIMK: LIM domain kinase; MAST: microtubule-associated Ser/Thr kinase; MRCK: myotonic dystrophy kinase-related CDC42-binding kinase; MSK: mitogen- and stress-activated kinase; PPp1R12a: protein phosphatase-1 regulatory subunit-12A [or MYPT1: myosin phosphatase-1]; NDR: nuclear Dbf2-related kinase [STK38]; NFκB: nuclear factor-κB; NHE3: Na + –H + exchanger; NuR: orphan nuclear receptor; PDGFR, platelet-derived growth factor receptor; PDK1: 3-phosphoinositide-dependent kinase; PP1: phosphatase-1; PTen, phosphatase and tensin homolog deleted on chromosome 10; RSK: P90 ribosomal S6 kinase; S6K: P70 ribosomal S6 kinase; SGK: serum- and glucocorticoid-induced protein kinase; SRF: serum response factor; STK: Ser/Thr protein kinase; YAP: yes-associated protein).
Kinase | Substrates |
---|---|
DMPK | Phospholamban, phospholemman, PPp1R12a, SRF |
GRK | Ezrin, GPCRs, PDGFR, P38MAPKα, synucleins |
LaTS | YAP1 |
MAST | NHE3, PTen |
MRCK | LIMK, PP1δ |
MSK | ATF1, CREB, histone-3, STK11, NFκB, NuR77 |
NDR (STK38) | |
PDK1 | PKB, PKC, PKN1, RSK, S6K, SGK |
Table 5.8
Kinases of the AGC superfamily and selected substrates (Part 2; Source: [300]; AID: activation-induced cytidine deaminase; AS160: Akt [PKB] substrate of 160 kDa; BAD: BCL2 antagonist of cell death; CKI: cyclin-dependent kinase inhibitor; CFTR: cystic fibrosis transmembrane conductance regulator; CREB: cAMP responsive element-binding protein; FHoD: formin homology domain protein; FOxO: forkhead box protein O; GP: glycoprotein; GRK: G-protein-coupled receptor kinase; GSK: glycogen synthase kinase; HDM: human double minute; HSL: hormone-sensitive lipase; IRS: insulin receptor substrate; KCa2.2: K + intermediate–small conductance Ca
-activated channel [SK2]; MARCK: myristoylated Ala-rich C-kinase substrate; NFAT: nuclear factor of activated T cells; PDCD: programmed cell death; PDE: phosphodiesterase; PFK2: 6-phosphofructo-2-kinase; PGC: PPARγ coactivator; PKA: cAMP-dependent protein kinase-A; PKC: protein kinase-C; PKG: cGMP-dependent protein kinase-G; PKN: protein kinase-C-related kinase; PLC: phospholipase; PRAS40: Pro-rich Akt [PKB] substrate of 40 kDa [PKB1S1 or Akt1S1]; PRK: protein kinase; PTPn6: protein Tyr phosphatase non-receptor-6 [a.k.a. SH2 phosphatase SHP1]; SKP: S-phase kinase-associated protein; TRPC: transient receptor potential cation channel; TSC: tuberous sclerosis complex; VASP: vasodilator-stimulated phosphoprotein; WNK: with no K [Lys] kinase).

Kinase | Substrates |
---|---|
PKA | AID, BAD, calpain-2, CFTR, CREB, GRK2, GSK3, |
HSL, KCa2.2, NFAT2, VASP | |
PKB | AS160, BAD, FOxO3, GSK3, HDM2, CKI1b, |
PFK2, PGC1α, PDCD4, PRAS40, bRaf, SKP2, TSC2, WNK1 | |
PKC | Adducin-1, GP130, GRK2, GSK3, IRS1, MARCK, |
PKD, PDE3a, cRaf, PTPn6 | |
PKG | FHoD1, PDE5, PLCβ3, TRPC3, VASP |
PRK | Polycystin-1 |
PKN1 | Histone-3, MARCK, Tau |
Table 5.9
Kinases of the AGC superfamily and selected substrates (Part 3; Source: [300]; ATF: activating transcription factor; BAD: BCL2 antagonist of cell death; CKI: cyclin-dependent kinase inhibitor; CCT: chaperonin-containing T-complex polypeptide; CRMP: collapsin response mediator protein; DAPK: death-associated protein kinase; eEF2K: eukaryotic elongation factor-2 kinase; eIF: eukaryotic initiation factor; ENaC: epithelial Na + channel; FOxO: forkhead box protein O; GSK: glycogen synthase kinase; IRS: insulin receptor substrate; LIMK: LIM domain kinase; MAD: MAX dimerization protein; MARCK: myristoylated Ala-rich C-kinase substrate; MAP3K: mitogen-activated protein kinase kinase kinase; MBS: myosin-binding subunit; MLC: myosin light chain; PPp1R12a: protein phosphatase-1 regulatory subunit-12A [or MyPT1: myosin phosphatase-1]; NDRG: N-Myc downregulated protein; NEDD4L: neural precursor cell expressed, developmentally downregulated 4-like isoform-2; NFAT: nuclear factor of activated T cells; PDCD: programmed cell death; Raptor: regulatory associated protein of TOR; RoCK: Rho-associated coiled-coil-containing protein kinase; RPS6, ribosomal protein-S6; RSK: P90 ribosomal S6 kinase; S6K: P70 ribosomal S6 kinase; SGK: serum- and glucocorticoid-induced protein kinase; SKAR: S6K1 Aly/REF-like target; STK: Ser/Thr protein kinase; TOR: target of rapamycin; TSC: tuberous sclerosis complex).
Kinase | Substrates |
---|---|
RoCK | Adducin-1, CRMP2, ezrin, radixin, moesin, LIMK, |
MARCK, MBS, MLC, PPp1R12a, RhoE, vimentin | |
RSK | ATF4, BAD, CCT, DAPK, eEF2K, eIF4b, GSK3β, |
STK11, NFAT3, MAD1, CKI1b, Raptor, RPS6, TSC2 | |
S6K | CCT, eEF2K, eIF4b, GSK3β, IRS1, |
MAD1, PDCD4, RPS6, SKAR, TOR | |
SGK | ENaC, FOxO3, MAP3K2, NDRG1, NEDD4L |
Members of the AGC superfamily have important functions in normal and pathological cellular processes, as they regulate cell survival, metabolism, motility, growth, division, and differentiation. These kinases indeed form a set of enzymes stimulated by growth factors and insulin. Multiphosphorylation primes kinase activation. However, occupancy of the nucleotide-binding site by phosphodonor ATP or, paradoxically, ATP-competitive inhibitor, induces hyperphosphorylation of PKB and PKC [301, 302]. Nucleotide binding (intrinsic mechanism), rather than ATP-dependent phosphotransfer launched by autophosphorylation (extrinsic mechanism that arises as a functional consequence of intrinsic kinase activity), stimulates enzymatic activity. Once phosphorylated, PKB and S6K intervene in PI3K pathway, PKC in calcium signaling, RSK and MSK in MAPK pathway, and PRK in Rho signaling.
5.2.1 Nuclear DBF2-Related Protein Kinases and Large Tumor Suppressors
Nuclear DBF2-related (NDR) protein kinases and large tumor suppressors (LaTS) constitute a set (LATS and NDR families) of the AGC superfamily of protein kinases. They control morphological changes, mitosis, cytokinesis, and apoptosis. Kinases of the NDR family are regulated by phosphorylation by kinases and dephosphorylation by phosphatase.
There are 4 related kinases: NDR1, NDR2, LaTS1, and LaTS2.40 Kinases NDR and LaTS possess an auto-inhibitory sequence in the kinase domain and an N-terminal regulatory motif. They are activated upon phosphorylation of their activation segment and hydrophobic motif.
Kinases NDR1 and NDR2 contribute to centrosome duplication. Kinases LaTS1 and LaTS2 participate in the Hippo pathway (Sect. 10.7) that controls cell size in organs [300].
Kinases of the NDR and LATS families interact with Mps-one binder (MOB) proteins to promote autophosphorylation of the activation segment and partial activation [300]. Six MOB proteins (MOB1a–MOB1b, MOB2, and MOB3a–MOB3c) are components of signaling pathways that control mitotic exit and centrosome duplication, hence cell proliferation, as well as apoptosis. Subtype MOB1a activates NDR1 and NDR2 kinases as well as LaTS1 and LaTS2 kinases [300]. Protein MOB2 binds to NDR1 and NDR2, but not to LaTS1 enzyme. In fact, 3 among the 6 MOB family members neither bind to nor activate NDR1, NDR2, LaTS1, or LaTS2 kinases [303]. Molecule MOB2 competes with MOB1a and MOB1b for NDR binding. Moreover, MOB2 inhibits NDR1 and NDR2 kinases, unlike MOB1a and MOB1b isoforms.
Kinases NDR1 and NDR2 are also regulated by calcium-binding proteins of the S100 family following Ca
import. Maximal activation occurs by phosphorylation of the hydrophobic motif of NDR and LATS by sterile-20-like kinases. Kinases MST1 (or STK4) and MST2 (or STK3) phosphorylate LaTS1 and LaTS2, whereas MST3 (or STK24) phosphorylates NDR1 and NDR2 [300]. The Ras association domain-containing protein RASSF1 activates MST1 and MST2.

5.2.2 Phosphoinositide-Dependent Kinase-1
Phosphoinositide-dependent Ser/Thr kinase-1 (PDK1) pertains to the AGC superfamily. It has multiple substrates that enable the transduction of extracellular signals. It participates in the regulation of the activity of protein kinases during cell signaling. Homodimerization of PDK1 is spatially and temporally regulated to control its activity. The spatial distribution of PDK1 homodimers differs from that of PDK1–PKB heterodimers.
Constitutively active PDK1 phosphorylates the activation segment of at least 23 AGC kinases: protein kinase-B, ribosomal S6K and RKS kinases, various PKC isoforms, PKC-related protein kinases PKN1 and PKN2, P21-activated kinase, and serum and glucocorticoid-regulated kinase (SGK) [304].
Kinase PDK1 possesses a pleckstrin homology (PH) domain at its C-terminus that binds phospholipids with high affinity. It preferentially binds phosphatidylinositol (3,4,5)-trisphosphate.41 It also has a high affinity for phosphatidylinositol (3,4)-bisphosphate42 and (4,5)-bisphosphate.43 It interacts with I(1,3,4,5)P4 and I(1,3,4,5,6)P5 with submicromolar dissociation constant.
Activity of PDK1 is governed by conformational changes. This activity is focused on protein–PDK1 and lipid–PDK1 interactions that occur with a given dynamics. Post-translational modifications such as phosphorylation of Ser, Thr, and Tyr residues, cause conformational changes, can stabilize the protein, and enable tethering to other proteins or specific domains of the plasma membrane and nucleus.
As PDK1 has a high basal level of activity, it is considered constitutively active, ready to phosphorylate substrates that have been targeted by various extracellular signals, owing to its intrinsic ability to transautophosphorylate (Ser241) its own activation segment.
Phosphorylation causes a mild (2- to 3-fold) increase in activity, but provokes membrane recruitment of PDK1 enzyme. Five phosphorylated Ser residues have been identified in PDK1, with autophosphorylation site (Ser241), as well as Tyr residues (Tyr9, Tyr373, and Tyr376) by ReT receptor Tyr kinase as well as cytosolic Abl, FAK2, and Src kinases in response to insulin, angiotensin-2, and oxidative stress [304]. Kinase PDK1 is sequestered by 14-3-3 proteins. It associates with HSP90 that increases its stability.
Homodimerization influences the behavior of intracellular kinases, as it can provoke the nuclear translocation and modify (elevate or lower according to the enzyme type) the kinase activity. Homodimerization of PDK1 depends on its PH domain [305]. A basal pool of PDK1 homodimers exist in the cytosol and at the plasma membrane. Upon stimulation such as PI3K-based signaling, the plasmalemmal concentration of PDK1 rises. The dimer is not required for autophosphorylation. Kinase PDK1 may exist as a conformational isomer, or conformer, loaded with ATP, that enable the autophosphorylation (Ser241) to destabilize the homodimer and become potentially active upon dissociation of the dimer. Increased amount of active monomer can support an augmented interaction with its PKB substrate. Monomeric PDK1 is the active conformation of the kinase; PDK1 dimer correponds to an autoinhibitory conformation [305]. In stimulated cells, the translocation of PDK1 to the plasma membrane that enables PKB activation is also associated with an elevated population of PDK1 homodimers. The dimerizarion may result from a negative feedback loop.
Conformational change caused by binding to 3-phosphoinositides, especially PI(3,4,5)P3, promotes PKB phosphorylation by PDK1 [300]. Colocalization of PKB and PDK1 is facilitated not only by phosphoinositides, but also scaffold proteins, such as growth factor receptor-bound protein GRB14 and coiled-coil and C2 domain-containing protein CC2D1a,44 and TNF receptor-associated factor TRAF6 Ub ligase. In addition, it binds to A-kinase-anchoring protein for RSK activation [300].
In cardiomyocytes, PDK1 coordinates cell survival and β-adrenergic response via the PI3Kγ–PDK1 axis [306]. Kinase PDK1 prevents cardiomyocyte apoptosis and preserves responsiveness to β-adrenergic stimulation that controls cardiac chronotropy and inotropy, as it avoids β1-adrenoceptor endocytosis primed by β-adrenoceptor kinase-1 (βARK1 or GRK2). It actually accommodates β-adrenergic response to prevent cardiac decompensation. Striated muscle-specific PDK1, especially myocardium-specific PDK1, is also necessary for cardimyocyte growth and its response to hypoxia.
5.2.3 Protein Kinase-A Family
Cyclic adenosine monophosphate (cAMP)-dependent protein kinases, or protein kinases-A, are tetrameric, inactive holoenzyme composed of 2 regulatory (PKAr) and 2 catalytic (PKAc) subunits. Binding of cAMP to PKAr releases PKAc from PKAr. Once the holoenzyme is dissociated and PKAc liberated, PKA is able to phosphorylate its substrates.
Ubiquitin ligases Praja (Pja1–Pja2) are binding partners of PKA enzyme.45 An elevated cAMP concentration promotes ubiquitination of the regulatory subunit by Praja-2 ligase and its subsequent degradation, thereby improving PKA signaling [307].
The catalytic subunit of protein kinase-A has 3 main conformations (apo, intermediate, and closed). The transition from apo (major state in the absence of ligand) to intermediate and from intermediate to closed conformation is triggered by ligand binding [308]. The catalytic subunit of protein kinase-A is inhibited by 2 types of regulatory subunits whether they require adenosine triphosphate or not. Protein kinase-A can thus have isoform-specific activators and antagonists for PKA [309]. Kinase PKA also requires phosphorylation of its activation segment (Thr197) by PDK1 kinase [300].
5.2.3.1 PKA Subtypes
Two major isoforms exist with different regulatory subunits: type-1 and type-2 cAMP-dependent protein kinases.
PKA1
Protein kinase-A1 possesses type-1 regulatory subunits that dimerize. These subunits have a very low affinity for A-kinase-anchoring proteins. It has a higher cAMP-binding affinity, thereby responding to a lower cAMP concentration in the cytoplasm. Protein kinase-A1 is mainly free in the cytoplasm.
PKA2
The regulatory dimer of protein kinase-A2 is made up of type-2 regulatory subunits. These subunits have a much higher affinity for AKAP proteins, thereby localizing to more precise sites. The AKAP scaffolds are involved in the spatial organization of signaling pathways, as they bring PKA into contact with its substrates. Specificity of PKA phosphorylation is, at least partly, achieved by the coupling of PKAr to AKAPs, which tether to different types of substrates.
5.2.3.2 PKA Effects
Activation of Gs-coupled receptors mobilizes localized pulses of cAMP messenger upon stimulation of adenylate cyclases. Protein kinase-A regulate specific and non-specific cellular processes. Specific substrates include AMPA-type glutamate receptor, fructose (2,6)-bisphosphatase, salt-inducible kinase-2 (inhibition), hormone-sensitive lipase, phosphorylase kinase, transcription factor cAMP response element-binding protein (CREB), protein phosphatase PP1 inhibitor I1, cystic fibrosis transmembrane conductance regulator (CFTR), aquaporin-2, and vasodilator-stimulated phosphoprotein (VASP).
PKA and Gene Transcription
Kinase PKA contributes to the regulation of gene transcription primed from G-protein-coupled receptors. It phosphorylates (activates) transcription factors CREB and nuclear factor-κB [300]. Its subcellular localization is directed by AKAP scaffolds that also determine the magnitude and duration of PKA signaling.
PKA and Transfer of Signaling Mediators
The antidiuretic hormone vasopressin stimulates V2 receptors (or AVPR2) in principal cells of collecting ducts of the nephron and causes the translocation of TRPC3 channels to the apical membrane via the activated AC–cAMP–PKA cascade [310].
Acute adaptation of epithelial sodium channel at the apical surface of epithelial cells of the nephron cortical collecting duct results mainly from exocytosis and ENaC insertion into the apical membrane owing to soluble Nethylmaleimide-sensitive factor attachment protein receptors and SNARE-binding protein complexin. A rapid stimulation of sodium transport is elicited by vasopressin that increases the intracellular cAMP concentration to activate protein kinase-A [311].
Phosphorylation by PKA of aquaporin-2 (Ser256) is necessary, but not sufficient, for Aqp2 translocation and insertion into the plasma membrane [312]. On the other hand, prostaglandin-E2 and dopamine induce Aqp2 internalization independently of its dephosphorylation, but upon activation of cAMP production.
In cardiomyocyte, myopodin is an actin-bundling protein that shuttles between the nucleus and Z-disc according to the cell differentiation status and stress context. Myopodin forms a Z-disc signaling complex with α-actinin, PP3, Ca
–calmodulin-dependent kinase CamK2, muscle-specific A-kinase anchoring protein AKAP6, and myomegalin (PDE4dIP) [313]. In myoblasts, phosphorylation of myopodin by protein kinase-A or CamK2 causes binding of myopodin to 14-3-3 protein, importin-α binding, and nuclear import. Conversely, dephosphorylation of myopodin by PP3 abrogates 14-3-3β binding. In adult cardiomyocytes, PKA activation or PP3 inhibition releases myopodin from the Z-disc and induces its nuclear import [313].

Density of cardiac NaV1.5 channel on the sarcolemma of cardiomyocytes rises upon activation of protein kinase-A [314]. In the absence of PKA stimulation, NaV1.5 channels reside in the perinuclear region as well as beneath the cell surface. Activated PKA promotes NaV1.5 transfer from both regions to the plasma membrane.
Voltage-gated KV4.2 channel is the α subunit of the channel that generates the A-type K + current in CA1 pyramidal neurons of the hippocampus to regulate dendritic excitability and adaptivity (i.e., synaptic change in strength — the so-called synaptic plasticity).46 Phosphorylation of KV4.2 by PKA enables KV4.2 transfer via its interaction with K + channel-interacting protein KChIP4a [315].
PKA and Cell Fate
Protein kinase-A influences migration and apoptosis of neutrophils and other cell types. Various inflammatory mediators accelerate or delay neutrophil apoptosis, thereby controlling the neutrophil number in tissues. Activation of type-1 cAMP-dependent protein kinases, but not type-2, reduces caspase-3-mediated apoptosis in neutrophils [317].
5.2.4 Protein Kinase-X1
Protein kinase-X1 (PKX1 or PrKX) is a cAMP-dependent protein kinase of the AGC superfamily of Ser/Thr protein kinases. It is related to PKA kinase. It is encoded by the Pkx1 gene on X chromosome. On chromosome Y, a gene encodes for homologous protein PKY1. Kinase PKX1 is synthesized in most adult and embryonic tissues [318]. However, it is mainly expressed during embryogenesis with high levels in the brain, heart, kidney, and lung. Concentration of PKX1 in adult tissue compared with 20-wk-old human fetal tissue is much lower (brain and kidney) or non-detectable (liver and heart). Kinase PKX1 is also expressed in macrophages and granulocytes and contributes to their maturation.
Its substrates include PKAr2 regulatory subunit, SMAD6, and TRPP1, or G-protein-coupled receptor-like polycystin-1. It is inhibited by the heat stable protein kinase inhibitor (PKI). Under unstressed conditions, it forms a heterotetrameric holoenzyme with PKAr1 regulatory dimer. It can be released from regulatory subunits upon binding of cAMP to these regulatory subunits.
Kinase PKX1 autophosphorylates. Autophosphorylation of PKX1 and phosphorylation is inhibited by PKA regulatory subunit isoforms PKAr1α and PKAr1β. The Prkx gene is expressed during maturation of monocytes, macrophages, and granulocytes under control of PKCβ [319].
5.2.5 Protein Kinase-Y1
P rotein kinase Y-linked (PKY1 or PRKY) is an Y-homolog of protein kinase PRKX. This cAMP-dependent Ser/Thr protein kinase is encoded by a gene that is located on chromosome Y.
5.2.6 Protein Kinase-B Family
Protein kinase-B (or Akt) has 3 isoforms (PKB1–PKB3 or PKBα–PKBγ). It serves as a mediator of growth factor signaling for cell survival and proliferation. Constitutively active PKB prevents apoptosis upon growth factor withdrawal, as it: (1) maintains transporters of and receptors for extracellular substances (glucose, amino acids, low-density lipoprotein, etc.), even in the transient absence of growth factors and (2) phosphorylates (inactivates) several pro-apoptotic mediators. Tumor cells do not initiate apoptosis upon growth factor withdrawal, as they augment PI3K and reduce PTen activity, so that PKB remains hyperactive.
Protein kinase-B phosphorylates numerous regulators that control: (1) glucose transport, glycolysis, glycogen synthesis, and suppression of gluconeogenesis to protein synthesis; (2) cell growth (size enlargement); (3) cell cycle progression; (4) cell survival, repression of apoptosis, and preservation of mitochondrial integrity.
In the absence of growth factors, inactive PKB is located in the cytoplasm. Kinase PKB is activated by liganded growth factor receptors via phosphatidylinositol 3-kinase. Because it phosphorylates phosphatidylinositol (4,5)-bisphosphate, activated PI3K generates a pool of plasmalemmal phosphatidylinositol (3,4,5)-trisphosphate that triggers PKB recruitment from the cytosol to plasma membrane and binds PKB. However, PKB linkage to plasma membrane is necessary but not sufficient to induce its hyperphosphorylation. Recruitment of PKB to the plasma membrane and its activation depend on cholesterol- and sphingolipid-rich PIP3-containing membrane nanodomains (rafts) [320]. Sphingolipid and cholesterol repress lateral diffusion of raft-associated proteins. Upon plasmalemmal contact, PKB is phosphorylated (activated) by phosphoinositide-dependent kinases PDK1 and PDK2 also located in membrane rafts. Kinase PKB is then released from the plasma membrane and phosphorylates both cytosolic and nuclear targets.
Protein kinase-B isoforms have signaling specificity in the regulation of metabolism, cell growth, proliferation, and apoptosis. Insulin activates both PKB1 and PKB2 in adipocytes. At the plasma membrane, PKB2 regulates incorporation of GluT4 glucose transporter, as it phosphorylates 160-kD GTPase-activating protein RabGAP Akt (PKB) substrate AS160 for GluT4 membrane recruitment [321].47
5.2.6.1 PKB Activation
Full PKB activation requires successive events: (1) membrane translocation that depends on phosphatidylinositol (1,4,5)-trisphosphate and (2) phosphorylation by phosphoinositide-dependent kinase-1 (Thr308) and target of rapamycin complex-2 (Ser473). These events can be primed by ATP-competitive PKB inhibitors upon occupancy of the ATP-binding domain that produces a PKB conformational change, thereby promoting membrane association and access of cognate residues (Thr308 and Ser473) for phosphorylation [301].48
Activation of PKB requires its phosphorylation on the activation loop by PDK1 kinase and on the hydrophobic motif by the TORC2 complex. In addition, IKKε and tumor-necrosis factor receptor-associated factor family member (TRAF)-associated NFκB (TANK)-binding kinase TBK1 phosphorylate PKB on both the hydrophobic motif and the activation loop in reponse to PI3K signaling [323].
5.2.6.2 Nuclear PKB
In addition to the plasma membrane and cytosol, the nucleus and mitochondria represent 2 other subcellular target sites for PKB action. Protein kinase-B can be activated at the plasma membrane or in the nucleus, eventually after PI3K and PDK1 translocation. PKB Targets that cause cell proliferation or prevents apoptosis reside within the nucleus (nuclear pool of PI(3,4,5)P3 generated by type-1 PI3K, regulatory GTPase PIKE, PI(3,4,5)P3-specific phosphatases SHIP2 and PTen, nucleophosmin,49 S6K, forkhead transcription factors, adaptor BRCA1, cyclin-dependent kinase inhibitors CKI1a and CKI1b, ubiquitin ligase double minute DM2, kinases PIM1, Wee, and ERKs, and tuberin) [324]. Nuclear PKB accumulation can serve to modulate localization of these effectors. Moreover, PKB regulator can translocate to the nucleus, such as PI3K, PDK1, and PTen. Kinase PI3K resides in the nucleus upon stimulation by insulin and growth factors (IGF1, PDGF, and NGF).
In cardiomyocytes, in response to cardioprotective stimuli such as atrial natriuretic peptide, both PI3K and PDK1 concomitantly accumulate in the nucleus. Cardioprotective and proliferative PKB effects rely on PIM1 kinase50 (Sect. 5.4.8) of the calmodulin-dependent protein Ser/Thr kinase-related (CAMK) superfamily [324]. In general, PIM1 production (upon stimulation by hormones, cytokines, and mitogens) and loss promotes cell survival and death, respectively.
Related isoforms, PIM2 and PIM3, have overlapping as well as distinct functions in cell growth and survival. Furthermore, mitochondrial PIM1 preserves mitochondrial integrity.
5.2.6.3 Mitochondrial PKB
Activated PKB translocates not only to the nucleus, but also to mitochondria. In mitochondria, PKB inhibits opening of the permeability transition pore to maintain mitochondrial integrity [324].51 Protein kinase-B phosphorylates BAD (Ser136; Vol. 2 – Chap. 4. Cell Survival and Death) that then dissociates from BCLxL to inhibit BAX–BAK mediated pore formation. In addition, PKB phosphorylates (inhibits) BAX as well as GSK3β to prevent opening of permeability transition pore. Protein kinase-B also phosphorylates (inhibits) mitochondrial serine peptidase HTRa252 that is released into the cytosol in response to stress, where it binds to and inhibits inhibitor of apoptotic proteins to preclude their caspase inhibition [324] (Table 5.10). Furthermore, PKB phosphorylates (inactivates) procaspase-9.
Table 5.10
Protein kinase-B and mitochondrial integrity (Source: [324]). Protein kinase-B preserves mitochondrial integrity against stress, as it stimulates or inhibits various agents at different subcellular sites (AIF: apoptosis-inducing factor; BAD: BCL2 antagonist of cell death; BAK: BCL2-antagonist/killer-1; BAX: BCL2-associated X protein; GSK: glycogen synthase kinase; HK: hexokinase; IP3R: IP3 receptor; NCX: Na + –Ca
exchanger; NHE: Na + –H + exchanger). Protein kinase-B favors Ca
outflux and hampers Ca
influx, as Ca
overload causes opening of permeability transition pores. Protein kinase-B phosphorylates (inactivates) NHE that extrudes H + and imports Na + . Na + influx precludes Ca
outflux through NCX and can even stimulate Ca
influx (reverse-mode). Protein kinase-B also phosphorylates (inhibits) type-1 IP3R.






Stimulation | Inhibition |
---|---|
Mitochondrion | |
HK | GSK3β |
AIF, BAD, BAX, caspase-9, HTRa | |
Plasma membrane | |
NCX | NHE |
Endoplasmic reticulum | |
IP3R |
Mitochondrial hexokinases — ubiquitous HK1 and insulin-sensitive cell-specific HK2 — preserve mitochondrial integrity and protect against oxidative stress. Moreover, HK2 impedes BAX to bind to mitochondria, thereby releasing cytochrome-C and apoptosis-inducing factor with subsequent activation of caspase-9 and -3 and apoptosis [324].53
In cardiomyocytes, BCL2-related BH3-only protein BNIP3L54 localizes at both the sarcoplasmic reticulum and mitochondria. Pro-apoptotic protein BNIP3L increases Ca
content of the sarcoplasmic reticulum up to Ca
overload. In mitochondria, BNIP3L induces mitochondrial outer membrane permeabilization in coordination with BAK and BAX [324].


5.2.6.4 PKB Effectors
Protein kinase-B mediates survival and proliferation signaling via various effectors. Isozymes PKB1 to PKB3 prime non-redundant signalings. Protein kinase-B1 can block cancer cell migration by inhibition of transcription factor nuclear factor of activated T cells and extracellular signal-regulated kinase [326]. Hyperproliferation and anti-apoptotic activities caused by stimulation of insulin-like growth factor-1 receptor are reversed by PKB2 downregulation [327].
Cell survival mediated by PKB relies on glucose metabolism. This kinase type increases surface concentration of glucose transporters, stimulates the mitochondrial association of hexokinase [328], and phosphorylates phosphofructokinase PFK2 to increase the production of fructose (2,6)-bisphosphate that regulates glycolysis.
Furthermore, PKB promotes the plasmalemmal residence of multiple transporters and receptors relevant to extracellular molecules required for metabolic homeostasis, such as amino acids, cholesterol, and iron, in addition to glucose [329].
5.2.6.5 PKB1 Isoform
Isoform PKB155 is implicated in cell metabolism, survival, and migration. It is activated by platelet-derived growth factor via phosphatidylinositol 3-kinase. In the developing nervous system, PKB1 intervenes in growth factor-induced neuronal survival. In the vascular system, it is the main isoform expressed in endothelial cells, in which it promotes endothelial nitric oxide synthase (NOS3). It contributes to placental angiogenesis.
In acute inflammation, PKB1 regulates leukocyte migration into inflamed tissues, especially neutrophil and monocyte infiltration [330]. It heightens vascular permeability, hence causing edema and leukocyte extravasation, via NOS3 and VE-cadherin. The latter partly dissociates from the cortical cytoskeleton. Endothelial-derived NO mediates microvascular permeability in the early phase. Kinase PKB1 is then required to ensure propermeability effect on postcapillary venules of pro-inflammatory mediators, such as bradykinin, histamine, tachykinins, complement, and nitrogen oxide species.
Activated PKB1 isozyme controls antiviral immunity via interferon-stimulated gene expression, as PKB1 phosphorylates (inactivates; Ser209) EMSY transcriptional repressor [331].56 The latter binds to BrCa2, but not BrCa1, with which it cooperates in transcriptional repression by binding to gene promoters with interferon-stimulated response element (ISRE).
5.2.6.6 PKB2 Isoform
Isoform PKB257 serves in signal transduction from insulin receptor. Abnormal PKB2 functioning causes impaired glucose tolerance and insulin resistance.
5.2.6.7 PKB3 Isoform
Isoform PKB358 is stimulated by platelet-derived growth factor, insulin, and insulin-like growth factor IGF1. It is highly expressed in the brain. Alternatively spliced transcript variants generate distinct subtypes.
5.2.6.8 PKB in Cell Migration
Two PKB isoforms, PKB1 and PKB2, intervene in cell motility with different roles and in a cell type-dependent manner. PDGF-induced cell motility mainly involves PKB1 that forms proteic complex with its upstream kinases PDK1 and PDK2 at the plasma membrane after translocation. The proteic complex formation is favored by group-1 P21-activated kinases PAK1 and PAK2 [115] (Sect. 5.2.13). Recruitment of PKB to the plasma membrane by binding to 3-phosphoinositides followed by PDK-mediated activation is indeed not sufficient for efficient PKB translocation and activation that require the PAK–PDK1–PKB complex. This complex restricts both spatially and temporally growth factor-initiated signaling and can contribute to PKB signaling specificity, as PKB has many substrates. On the other hand, PKB phosphorylates (moderately activates) PAK1 that then dissociates from NCK and focal adhesions. In addition, PDK1 phosphorylates (activates) PAK1 kinase.
5.2.7 Protein Kinase-C Family
The family of typically membrane-localized, lipid-activated protein kinase-C represents about 2% of the human kinome with 12 known genes. Members of the PKC family transduce signals that are transmitted via the hydrolysis of membrane phospholipids.
This family includes multiple enzymes that have specific tissue expression and contribute to the spatial organization of signal propagation owing to their compartmentalized action (localized second messenger production and interaction with membrane-anchored small GTPasess, spatial organizers such as scaffold proteins, and accessory proteins).
These kinases can be divided into 3 main groups: (1) conventional, or classical, PKCs (cPKC) diacylglycerol- and calcium-sensitive isoforms (PKCα, PKCβ1, PKCβ2, and PKCγ); (2) novel (nPKC) calcium-independent isoforms (PKCδ, PKCε, PKCη, and PKCθ) that are activated by phosphatidylserine and diacylglycerol; and (3) atypical (aPKC) PKCs (PKCζ and PKCλ/ι) that are activated neither by calcium nor diacylglycerol, but by phospholipid cofactors as well as stimulus-induced phosphorylation. In addition, atypical PKCs can be allosterically activated by the Par6–CDC42 complex59 that specifies cell polarity [332]. A fourth family encompasses protein kinase-N (PKN1–PKN3). Small GTPases Rho or Rac tether to PKN kinases and disengages their pseudosubstrate to activate the kinase function. The PKC isozymes are expressed in all tissues.60
Conventional PKCs possess 4 common, homologous domains (C1–C4) that are interspaced with isozyme-unique, variable (V) domains. The PKC isozymes have a protein kinase region linked to C1 and C2 domains. The C1 domain binds to diacylglycerol. The C2 domain connects to negatively charged lipids such as phosphatidylinositol (4,5)-bisphosphate and Ca
cations. Domains C1 and C2 are regulatory membrane-targeting motifs. Domains C3 and C4 contain ATP- and substrate-binding sites.61 Novel PKCs have C2 domains that lack calcium-coordinating acidic residue side chains that confer calcium sensitivity, but serve in between-protein interactions. Atypical PKCs lack a calcium-sensitive C2 domain and contain only a single cysteine-rich zinc finger structure that does not bind DAG.

Activity of PKCs requires priming phosphorylations at 3 sites within the kinase domain. Priming phosphorylations depend on the conformation of the nucleotide-binding pocket, rather than autophosphorylation, i.e., intrinsic kinase activity [302]. Removal of ATP from the nucleotide-binding site of PKC induces rapid dephosphorylation of all PKC phosphorylated residues. Occupancy of ATP-binding pocket by PKC inhibitors can also trigger phosphorylation of inactive PKC.
Activation of PKC is primed by phosphorylation that is followed by the recruitment of PKC to the membrane by Ca
as well as both PIP2 and DAG for effective activation.62 The PKC isoforms are activated by at least 2 phosphorylations: one of their activation segment and another of their hydrophobic motif (when present). Phosphoinositide-dependent protein kinase PDK1 phosphorylates the activation segment, whereas target of rapamycin complex-2 phosphorylates the hydrophobic motif of at least some isoforms [300]. However, these modifications alone are not sufficient for activation, as phosphorylated PKCs can be maintained in an inactive state, until the release of the pseudosubstrate.

Lipid mediators are not selective for a given PKC isoform. Products of phosphatidylinositol 3-kinase indeed activate novel (PKCε and PKCη) as well as atypical PKCs (PKCζ and PKCι).
Activated by diacylglycerol and calcium ions, PKCs are involved in signaling initiated by certain hormones, growth factors, and neurotransmitters. Diacylglycerol causes PKC translocation from the cytosol to the plasma membrane, where it binds calcium ions and plasmalemmal phosphatidylserine. These events relieve PKC autoinhibition. Kinase PKC moves to the cytoskeleton, perinuclear sites, and nucleus.
Phosphorylation of various PKC substrates leads to either increased or decreased activity.63
Activity of all of the members of the PKC family is influenced by guanine nucleotide-binding proteins, either indirectly via G-protein-coupled receptors that signal via heterotrimeric G proteins to control allosterically phospholipase-C and subsequent generation of diacylglycerol for PKC activation or directly via small GTPase activators. Atypical PKCs are regulated by small GTPases CDC42 and Rac that act via partitioning defective Par6 [332].
Isoforms PKCβ1 and PKCε control tight junction integrity. Kinase PKCη is expressed predominantly in epithelia, where it regulates tight junctions that constitute a barrier between apical and basolateral membranes [334]. Tight junction is made of at least 3 types of transmembrane proteins, occludin, claudins,64 and junctional adhesion molecules. Isozyme PKCη phosphorylates occludin for tight junction integrity.
5.2.7.1 PKC-Anchoring Proteins
Protein kinase-anchoring and scaffold proteins are essential components of cell signaling. Isozyme specificity results, at least partly, by association with specific anchoring proteins. A heterogeneous set of PKC-binding proteins actually influence PKC subcellular localization, substrate availability, exposure to allosteric activator, and activation-dependent relocation within cells. These proteins include: (1) PKC-binding proteins and PKC substrates that interact with PKC prior to phosphorylation [335]; (2) cytoskeleton–vesicle interacting proteins; (3) A-kinase-anchoring proteins; and (4) receptors for activated C-kinase that are non-substrate proteins that bind to active PKC.
The PKC isoforms not only can be recruited to scaffolds with other transducers, but also can control the behavior of signaling mediator complexes, as they influence their assembly or disassembly and subcellular localization, without incorporating these complexes [332].
Substrates that interact with C-kinase (STICK), such as myristoylated alanine-rich C-kinase substrate (MARCKS) and its related protein MacMARCKS, as well as α- and γ-adducins, among others, are not only PKC-interacting proteins, but also PKC substrates [335]. Binding parters of PKC that are PKC substrates and directly bind phosphatidylserine include talin, vinculin, MARCKS, β-adducin homolog, and AKAP5 and AKAP12 [336]. Sdr-related gene product that binds to C-kinase (SBRC) is a substrate of PKC (PKCα and PKCδbut not to PKCζ) that binds to PKC regulatory domain in a phosphatidylserine-dependent manner and is phosphorylated after PKC activation.
Localization of PKC to different intracellular sites is directed by specific PKC-binding proteins that can discriminate between different PKC isoforms. PDZ (PSD95 [DLg4], Disheveled, and ZO1) domain-containing proteins mediate between-protein interactions during receptor and ion channel clustering and recruit kinases and phosphatases to their membrane-associated substrates. In addition, certain PDZ domains can homo-oligomerize to form complexes at specific subcellular sites.
Protein interacting with C-kinases (PICK), a protein kinase-Cα-binding protein, is a PDZ domain-containing PKC substrate that interacts with a PDZ-binding motif (QSAV) at C-terminus of PKCα to selectively recruit PKCα to suitable subcellular sites [337].
Several different Receptor for inactive C-kinases (RICK) can anchor a given PKC isozyme to different subcellular sites in the inactive state. Bruton Tyr kinase (Sect. 4.12.4) binds to all the inactive PKC isozymes in a lipid-dependent fashion [336]. Kinase BTK thus acts as a RICK.
Receptor for activated C-kinase (RACK) is a membrane-associated anchoring protein responsible for the binding of active forms of the PKC family, hence directing PKC isoform subcellular localization. Different RACKs exist for different isoforms of PKC, thereby preserving the specificity of signaling cascades. Protein RACK1 is not a substrate for PKC, but forms an active PKC–RACK1 complex that raises 7-fold PKC-substrate phosphorylation [336]. Protein RACK1 colocalizes with activated PKCβ2 to the perinucleus in cardiomyocytes.
5.2.7.2 Atypical PKCs as Kinases and Dockers
Atypical PKCs intervene in signaling responses as kinases or binding proteins. The V1 sequence of aPKCs serves as binding site for proteins that regulate apoptosis and cell polarity such as scaffold sequestosome-1; PKC, apoptosis, WT1 regulator (PAWR);65 cell-polarity partitioning-defective protein Par6; and Partitioning defective-3 homolog (Par3).66
Atypical PKCs — PKCζ and PKCι— bind PDK1 (Sect. 5.2.2). They have a single zinc-finger domain in their regulatory region that binds PKC, apoptosis, WT1, regulator (PAWR). The latter selectively inhibits both aPKCs [347]. The PB1 (V1) region interacts with scaffolds sequestosome-1 and Par6 that serve to connect PKCζ to nuclear factor-κB and to control cell polarity, respectively. Protein Par6 forms a complex with active CDC42GTP, Par3, and aPKC to contribute to the formation of tight junctions that establish cell polarity in epithelial cells [338, 339]. Protein Par6 hence serves as an adaptor that links CDC42 and aPKCs to Par3 agent.
Atypical protein kinases PKCζ and PKCι are located in the cytosol, where they can associate with endosomes via sequestosome-1 and tight junctions via Par6 protein. Upon nerve growth factor stimulation, sequestosome-1 facilitates polyubiquitination of TRAF6 and formation of the Sqstm1-TRAF6-IKKβ-PKCι signaling complex that activates the NFκB pathway [340].
5.2.7.3 PKC in Cell Interactions with Environment
The PKC isoforms are involved in homo- and heterotypic intercellular recognition as well as cell–extracellular matrix interactions, particularly during cell migration. Isozyme PKCθ is highly synthesized by T lymphocytes. It regulates recognition by effector T lymphocytes of antigen-presenting cells via T-cell receptor that recognizes a processed antigen bound to a major histocompatibility complex class-1 or -2 molecule on antigen-presenting cells [332]. This recognition triggers assembly of an immunological synapse with plasmalemmal subdomains67 asymmetric T-cell division and differentiation into CD4 + T helper cell (TH1, TH2, and TH17). Subtype PKCθ contributes to activation of transcription factors, such as nuclear factor-κB, nuclear factor of activated T cells, and activator protein AP1, as well as other transcription factors involved in the differentiation of CD4 + helper T cells.
In polarized cells characterized by subapical tight junctions, apically located atypical protein kinase-C isoforms can regulate the formation, composition, and localization of polarity complexes via their scaffold and catalytic activities. In cell apex, aPKCs complex with polarity proteins, the partitioning defective proteins Par3 and Par6 (Par3–Par6–aPKC complex). In addition, the PAR6–aPKC heterodimer is targeted by CDC42 and Rac1 GTPases to relieve PAR6-induced inhibition of aPKCs [332]. Atypical PKCs phosphorylate various proteins, such as protein associated with LIN-7 PALS1, PALS1-associated tight junction protein (PATJ), crumbs protein homolog CRB3, Par1, Par3, and Par6, basolateral domain proteins in epithelial polarized cells, such as mammalian lethal giant larva proteins LGL1 and LGL2, Disc large homolog DLg1 and Scribble homolog; and 14-3-3 scaffolds [332].68
Isoform PKCα promotes transfer of activated hepatocyte growth factor receptor along microtubules from peripheral early endosomes, where HGFR remains active, to perinuclear endocytic recycling compartment, where it can efficiently activate signal transducer and activator of transcription STAT3, overcome the action of phosphoTyr phosphatases more strongly than in early endosomes, and cause nuclear accumulation of phosphorylated STAT3 [332].
The PKC subtypes contribute to cytoskeletal dynamics during cell migration. They interact with numerous plasmalemmal proteins, e.g., PKCα with syndecan-4 and β1-integrins. Atypical PKCs that pertain to activated Par complex or exocyst complex are required for cell migration. In migrating kidney cells, PKCζ and PKCι complex with kidney and brain protein (KIBRA) and the exocyst complex and localize to the leading edge of moving cells, where they phosphorylate (activate) components of the MAPK module, such as Jun N-terminal kinase JNK1 and extracellular signal-regulated kinases ERK1 and ERK2 that all phosphorylate cytoskeletal paxillin [332]. In migrating astrocytes α- and β-integrins activate small GTPase CDC42 at the leading edge that then recruits and activates the Par6–PKC complex. Atypical PKCs then phosphorylate (inactivate) glycogen synthase kinase GSK3β to reorientate the centrosome, i.e., the main microtubule-organizing center, for directed cell migration, as it causes GSK3β substrate adenomatous polyposis coli to connect to microtubule plus-ends at the leading edge [332]. Moreover, atypical PKC acts via the microtubule nanomotor dynein to determine the polarity of the centrosome and Golgi body.
5.2.7.4 Protein Kinase-Cα (Conventional PKC)
Protein kinase-Cα, encoded by the PRKCA gene, is activated in response to hormones, mitogens, and neurotransmitters. In unstimulated cells, PKCα resides primarily in the cytoplasm. Upon stimulation by signals that promote the hydrolysis of membrane phospholipids, PKCα translocates to the plasma membrane as well as the nuclear envelope. This kinase operates in many cellular processes, such as cell cycle checkpoint, cell adhesion and volume control, as well as transformation.
Protein kinase-Cα is located in focal contacts in non-stimulated fibroblasts and, once activated, translocates to the perinuclear region. In the myocardium, PKCα regulates contractility and hypertrophy.
Among classical (PKCα and PKCβ) and novel (PKCδ and PKCθ) isoforms synthesized by platelets, PKCα regulates thrombus formation [341]. Dense-granule genesis and secretion depend on PKCα.
5.2.7.5 Protein Kinase-Cβ (Conventional PKC)
Protein kinase-Cβ, encoded by the PRKCB1 (or PRKCB2) gene, is involved in various cellular functions, such as B-cell activation, apoptosis, endothelial cell proliferation, and intestinal sugar absorption. Both isoforms – PKCβ1 and PKCβ2 – are produced from the same gene. Activated PKCβ triggers expression of early growth response EGR1 and pro-inflammatory and procoagulant molecules that lead to acute vascular stresses.
Protein Kinase-Cβ1
Protein kinase-Cβ1 interacts with many substances, such as kinase receptor-interacting protein kinase RIPK4, β-adrenergic receptor kinase AdRβK1 (or GRK2), PDPK1, CDK1, and BTK, as well as colony-stimulating factor-2 receptor-β, G-protein subunits Gα12 and Gα13, receptor for activated C kinase RACK1,69 regulator of G-protein signaling RGS2, RasGRP3, Rho activator TIAM1, NADPH oxidase organizer-2,70 actin filament-associated protein (AFAP), lamin-B1, neural cell adhesion molecule NCAM1, and 14-3-3γ [251].
Protein Kinase-Cβ2
Protein kinase-Cβ2 associates with fibrillar structures in resting cardiomyocytes and, once activated, translocates to the perinucleus and cell cortex. Filamentous actin binds PKCβ2, but not PKCβ1.
Protein kinase-Cβ2 is a major mediator of TNFα-induced vascular endothelial cell apoptosis. In addition, PKCβ2, as well as PKCε, but neither PKCα nor PKCδ, yields full activation of voltage-gated calcium channelsCaV2.2 via the stimulatory site (Thr422). Isozyme PKCε is better at attenuating channel activity via the inhibitory site (Ser425). In any case, the stimulating effect of PKCβ2 or PKCε is dominant over their inhibitory action [342]. Whereas PKCα intervenes in the potentiation of CaV2.3 flux by muscarinic M1 receptor agonist, PKCβ2 and PKCε contribute to the potentiation of CaV2.3 current by phorbol-12-myristate, 13-acetate [343]. The differential response of CaV fluxes to chemical stimuli is attributed to: (1) type of activated PKC isozyme and (2) PKC-selective phosphorylation sites in drug-binding, channel pore α1 subunit of CaV channels.
5.2.7.6 Protein Kinase-Cγ (Conventional PKC)
Protein kinase-Cγ, encoded by the PRKCG gene, has a localization restricted to neurons, where it operates in long term potentiation (LTP) and depression (LTD).
This kinase interacts with ionotrophic, AMPA-type glutamate receptor GluR4, GABAA receptorα1 and -α4, actin filament-associated protein (AFAP), Rho activator TIAM1, regulator of G-protein signaling RGS2, and 14-3-3γ [251].
5.2.7.7 Protein Kinase-Cδ (Novel PKC)
Protein kinase-Cδ contributes to cell growth inhibition and apoptosis, as well as mitogenesis, differentiation, tumor progression, and tissue remodeling during inflammation [344]. Its lipid cofactors that interact with the C1 domain, promote PKCδ translocation to membrane and induce a conformational change that removes auto-inhibition by expelling the N-terminal auto-inhibitory domain from the substrate-binding pocket. It can also be activated from phosphorylation by SRC family kinases and Abl1 in cells subjected to oxidative stress as well as caspase-mediated proteolysis during apoptosis [344].
Protein kinase-Cδ bears a series of sequential priming phosphorylations (Thr505, Ser643, and Ser662). Phosphorylated PKCδ (at a Tyr residue) does not translocate to membranes. Phosphorylation mediated by Src (Tyr64 and Tyr565) causes inactivation [344]. The single known RACK for PKCδ is the 32-kDa PKC-interacting glycoprotein complement component-1, q subcomponent-binding protein C1qBP71
Protein kinase-Cδ can exert kinase-independent effects, as it can act as a signal-regulated scaffold to translocate signaling mediators that associate with PKCδ, such as SRC family kinases, lipid or protein phosphatases, and adaptor proteins such as Shc [344]. In vascular smooth muscle cells, inactive PKCδ mimicks the effect of wild-type PKCδ and induces apoptosis.
Protein kinase-Cδ interacts with plasmalemmal actin filament crosslinker myristoylated alanine-rich PKC substrate MARCKS, axon constituent growth-associated protein GAP43, kinases, such as SRC family kinases (Src, Fyn, Lyn), Abl1, PI3K, DNAPK, TOR, and receptor-interacting kinase RIPK4,72 insulin receptor and IRS1, protein phosphatases, such as PP2 and PTPn6, adaptor SHC, actin, STAT3, and NADPH oxidase [344].
5.2.7.8 Protein Kinase-Cε (Novel PKC)
In cardiomyocytes, PKCε, encoded by the PRKCE gene, is located in the nucleus and perinuclear region before stimulation to translocate upon activation to sarcomeres and intercellular contacts. Isozyme PKCε, but not other PKC isoforms, binds to filamentous actin and synaptosomes.
This kinase interacts with voltage-dependent anion channel VDAC1, adenine nucleotide translocator SLC25a4, CFTR channel, receptor for activated C kinase RACK1, cRaf kinase, Rho activator TIAM1, RasGRP3, Gα12 and Gα13, glutamate decarboxylase-1 and -2, hexokinase-2, actin-α1, actin filament-associated protein (AFAP), myosin heavy chain-9, keratin-1, -8, and -18, connexin-43, PI3K-binding protein centaurin-α coatomer protein complex subunit-β2, and 14-3-3ζ [251].
5.2.7.9 Protein Kinase-Cη (Novel PKC)
Protein kinase-Cη, encoded by the PRKCH gene, is involved in neuron channel activation, apoptosis, cardioprotection from ischemia, heat shock response, insulin exocytosis, as well as lipopolysaccharide-mediated signaling in activated macrophages. It interacts with cyclin-dependent kinase CDK2.
5.2.7.10 Protein Kinase-Cθ (Novel PKC)
Protein kinase-Cθ, encoded by the PRKCQ gene, is required for the activation of the transcription factors NFκB and AP1. It interacts with kinases PKB, Fyn, LCK, and IKKβ, basic helix–loop–helix transcription factor TAL1, thioredoxin-like-2, guanine nucleotide-exchange factor Vav1, and 14-3-3γ [251].
Protein kinase-Cθ is expressed in T lymphocytes, in which it fosters activation of mature effector T lymphocytes. During T-cell activation, PKCθ is recruited to the interface between the T lymphocyte and activating antigen-presenting cells [346]. However, in regulatory T lymphocytes, PKCθ, an inhibitor, is sequestered from the activating cellular interface.
5.2.7.11 Protein Kinase-Cζ (Atypical PKC)
Atypical protein kinase-Cζ, encoded by the PRKCZ gene, phosphorylates transcription factor RelA NFκB (Ser311), cytoskeletal lethal giant larva homologous protein LGL1 that associates with non-muscle myosin-2 heavy chain, and Crumbs homolog CrB1 expressed specifically in human retina and brain, which organizes an intracellular protein scaffold for the assembly of zonula adherens [347].
Atypical PKCs can be regulated by certain lipid messengers, such as ceramide and phosphatidylinositol (3,4,5)-trisphosphate. Second messengers stimulate PKC by binding to the regulatory domain, hence translocating the enzyme from cytosol to membrane and producing a conformational change that relieves PKC auto-inhibition.
Protein kinase-Cζ is ubiquitous with its highest level in the lung. The brain-specific isoform PKMζ is generated from an alternatively spliced transcript. It lacks the regulatory region of full-length PKCζ, hence being constitutively active. In fact, PKMζ corresponds to the catalytic domain of PKCζ.
GluT4 + vesicle contains VAMP2 that binds to the Stx4–SnAP23 complex formed by syntaxin-4 and synaptosome-associated protein SnAP23 via syntaxin-binding protein StxBP3.73 Protein StxBP3 prevents GluT4 vesicle binding to the plasma membrane in the basal state. Once stimulated by insulin, PKCζ removes the StxBP3 clamp and triggers GluT4 vesicle translocation to and docking in the plasma membrane [348].
PKCζ and Its Splice Variant PKMζ
Learning and memory relies on long-term potentiation mediated by the excitatory neurotransmitter glutamate and maintenance of persistent synaptic connections. Long-term memory and glutamate-induced dendritic protein synthesis relies on full-length protein kinase-Cζ and its N-terminal truncated alternative transcript, brain-specific, constitutively active, protein kinase–Mζ. In dendritic spines and shafts, peptidyl-prolyl isomerase Protein interacting with NIMA1 (PIN1) prevents synthesis of PKMζ as well as dendritic translation [349]. Conversely, PKMζ phosphorylates (Ser16; inhibits) PIN1. Glutamate signals preclude PIN1 activity, thereby enabling PKMζ synthesis. A positive feedback is hence created, as PKMζ impedes PIN1 function, which enables long-term potentiation to perpetuate mental representations of history.
PKCζ-Interacting Proteins
Interactors of PKCζ comprise fasciculation and elongation protein-ζ(FEZ1), or zygin-1, and scaffold sequestosome-1. The former mainly resides in the plasma membrane, where it can associate with PKCζ to be phosphorylated; it is involved in axon guidance. The latter generates complexes between PKCζ and other proteins. Sequestosome-1 is a ubiquitin-binding protein in neuronal and glial cells that regulates NFκB activation in response to nerve growth factor, interleukin-1, and tumor-necrosis factor, i.e., in both immune and nervous systems, via the formation of PKCζ–Sqstm1–TRAF6–zyxin or ajuba complex [350]. Counteracting sequestosome-1 shuttles substrates for proteasomal degradation [351].
5.2.7.12 Protein Kinase-Cι (PKCλ; Atypical PKC)
Protein kinase-Cι is the human homolog of mouse PKCλ. Like PKCζ, PKCι is implicated in cell proliferation and survival via transcription factors AP1 and NFκB, respectively. Isoform PKCι is activated upon PDGFR stimulation by the PI3K pathway. Atypical PKC isotypes contribute to activation of mitogen-activated protein kinase.
PKCι-Interacting Proteins
A selective activator of PKCι — (PKC)λ-interacting protein (LIP) — does not even bind to the highly related PKCζ. On the other hand, both PKCζ and PKCι participate in the regulation of NGF-induced cell differentiation via PKCζ-binding protein Sqstm1 [352]. Shuttle Sqstm1 and PKCι colocalize with Rab7 GTPase in late endosomes regardless of PKCι phosphorylation state.
5.2.8 Protein Kinase-D Family
The family of Ser/Thr protein kinase-D includes PKD1 (or atypical PKCμ), PKD2, and PKD3 (or PKCν). As PKD1 was originally reported to be an atypical isoform of PKC, PKD was incorporated in the PKC family. However, PKD differs from PKC by amino acid composition, domain structure, regulation, and substrate specificity. In particular, the catalytic domain of PKDs has sequence homology with myosin light-chain kinase and calmodulin-dependent kinase. The 3 isoforms of PKD are now classified in a proper family within the CAMK superfamily.
The PKD kinases operate downstream from activated G-protein-coupled receptors and receptor Tyr kinases that transmit signal via phospholipase-C. They regulate cellular processes, such as proliferation, vesicular transport, apoptosis, and stress response, as well as immunity.
5.2.9 Protein Kinase-G Family
Isozymes of protein kinase-G — PKG1 and PKG2 —, or cGMP-dependent protein kinase are encoded by 2 genes. They are activated by cyclic guanosine monophosphate. This second messenger is produced by soluble and transmembrane guanylate cyclases activated by nitric oxide and natriuretic peptides, respectively. Type-1 homodimeric PKG is widespread. On the other hand, type-2 dimeric PKG has a limited tissue distribution.
Two PKG1 isoforms exist: PKG1α and PKG1β. Subtype PKG1α is activated at about 10-fold higher cGMP concentrations than PKG1β isoform. Both PKG1 subtypes are homodimers. Each subunit is composed of 3 functional domains: (1) N-terminal leucine zipper autoinhibitory domain that mediates homodimerization, suppression of kinase activity in the absence of cGMP, and interactions with proteins, as well as autophosphorylation sites that control PKG basal activity; (2) cGMP-binding regulatory motif; and (3) C-terminal kinase sequence.
Enzyme PKG1 is observed at high concentration in all types of smooth muscle cells (both isoforms) and platelets (predominantly PKG1β) and at lower level in vascular endothelial cells and cardiomyocytes.
Enzyme PKG2 resides in renal cells, zona glomerulosa cells of the adrenal cortex, Clara cells in distal airways, among other cell types (but not in cardiac and vascular myocytes).
Kinase PKG1 participates also in vascular tone control, platelet activation, and synaptic remodeling. In vascular smooth muscle cells, PKG1 has an antiproliferative function, in addition to relaxation. Kinase PKG phosphorylates myosin light-chain phosphatase that dephosphorylates myosin light chains to initiate smooth muscle relaxation. Calcium influx, mainly via store-operated Ca
channels in non-excitable cells, controls gene regulation, cell contraction, exocytosis, and apoptosis. Vascular endothelial cells respond to numerous stimuli by secreting vasoactive factors. Most often, the initial response of endothelial cells involves Ca
release from intracellular stores. Store depletion activates Ca
entry. Elevated cGMP level attenuates the store-operated Ca
import via PKG in vascular endothelial cells [353]. Kinase PKG also modulates calcium entry by acting on CaV1 and mechanosensitive Ca
channels, thereby changing the intracellular calcium concentration for the activity of nitric oxide synthases NOS1 and NOS3. In addition, PKG directly stimulates NOS3 and soluble guanylate cyclases, and inhibits phosphodiesterase PDE5 that selectively degrades cGMP.





Kinase PKG1 phosphorylates transcription factors to prime Fos promoter response. In addition, PKG1 colocalize with SMAD1 at the Id1 promoter on BMP2 stimulation [354].
5.2.10 CDC42-Binding Protein Kinases
CDC42-binding protein kinase-α (CDC42BPα)74 possesses a CDC42–Rac-interactive binding (CRIB) domain. It hence can bind CDC42 to promote cytoskeletal reorganization and nuclear displacement to cell rear during cell migration. It phosphorylates myosin light chain to activate actomyosin filament contraction.
5.2.11 G-Protein-Coupled Receptor Kinase Family
G-protein-coupled receptor Ser/Thr kinases (GRK1–GRK7) recognize active GPCRs and phosphorylate these receptors for desensitization. Multiple GRKs (GRK2, GRK3, GRK5, and GRK6) are located in the central nervous system.
G-protein-coupled receptor kinases are classified into 3 categories: (1) rhodopsin kinase (RK or GRK1); (2) β-adrenergic receptor kinases (βARK1–βARK2, i.e., GRK2 and GRK3, respectively); and (3) GRK4 category (GRK4–GRK6). Both category-2 and -3 GRKs bind to PI(4,5)P2 lipid.
Once they are phosphorylated by GRKs, GPCRs bind to arrestins that prevent reassociation of G proteins with their receptors, hence activation of G proteins by GPCRs despite continuous binding of agonist. Phosphorylated Ser and Thr residues in the cytoplasmic loops and tails of activated GPCRs indeed act as binding sites for arrestins. Phosphorylation and desensitization of GPCRs can be followed by endocytosis and resensitization.
Unlike most AGC kinases, GRKs are not activated by phosphorylation of the activation segment or hydrophobic motif. Instead, activation results from a conformational rearrangement triggered by GRK interaction with activated GPCRs at the plasma membrane [300].
Phosphorylation of G-protein-coupled receptors by GRK kinases governs interactions of GPCRs with β-arrestins that control transfer of and signaling from these receptors. β-Arrestins tether to GPCRs and end G-protein activation after phosphorylation of specific Ser residues by GRK kinases as well as redirect the signaling to the MAPK module. β-Arrestin-1 is also recruited to receptor Tyr kinases such as insulin-like growth factor IGF1 receptor, thereby regulating receptor degradation and signaling. β-Arrestin-1 serves as an adaptor to link DM2 ubiquitin-ligase to IGF1R, thereby assisting in IGF1R degradation. On the other hand, IGF1R complexed to β-arrestin-1 activates the MAPK module. Interaction between β-arrestin and IGF1R supported by GRK2 and GRK6 is transient and stable, respectively [355]. Whereas GRK5 and GRK6 phosphorylate Ser1291 of IGF1R and promote IGF1- and β-arrestin-mediated ERK and late-phase PKB activation, GRK2 that targets Ser1248 of IGF1R represses ERK activation, but mildly supports early phase PKB signaling [355]. The stable βArr–IGF1R association enables increased ERK phosphorylation. In addition, GRK2 reduces and GRK6 enhances IGF1R degradation. In summary, distinct GRKs generate different RTK phosphorylation patterns that create different functional outcomes mediated by recruited β-arrestins.
G-protein-coupled receptor kinases interact also with several other proteins, such as calmodulin, caveolin, Raf kinase inhibitor protein, and GPCR kinase interactors APAP1 and APAP2, as well as kinases PI3K, PKB, and MAP2Ks.
5.2.11.1 GRK1
Kinase GRK1 interacts with caveolin-1, lipid-binding synuclein-α, -β, and -γ that are primarily expressed in the nervous system, neuronal calcium-binding protein recoverin that is mainly observed in photoreceptors, and rhodopsin [251]. Membrane localization of GRK1 relies on its farnesylation [300].
5.2.11.2 GRK2
Kinase GRK2, or β-adrenergic receptor kinase-1, interacts not only with β2 adrenoceptors, but also other GPCRs, such as endothelin receptor ETRb (or ET B ), angiotensin receptor-1, chemokine receptor CCR4, and follicle-stimulating hormone receptor. It also connects to Src kinase and protein kinase-Cβ1, Gαq and Gα15 subunits, phosphodiesterase-6G, APAP1 and APAP2, caveolin-1, rhodopsin, phosducin, and phosducin-like protein [251].
Binding of GRK2 to both Gq and Gβγ subunits prevents their interaction with effectors [300]. Kinase PKA phosphorylates (Ser685) GRK2, thereby enhancing its ability to bind to Gβγ. On the other hand, ERK1 phosphorylates GRK2 (Ser670) and impedes GRK2–Gβγ binding.
5.2.11.3 GRK3
Kinase GRK3, or β-adrenergic receptor kinase-2, interacts with GPCRs, such as chemokine receptors CCR4 and CXCR4, as well as APAP1 [251].
5.2.11.4 GRK4
5.2.11.5 GRK5
Kinase GRK5 associates with angiotensin receptor-1, arginine vasopressin receptors AVpR1a (V1A ) and AVpR2 (ADHR), neurokinin-1 receptor (substance-P or tachykinin receptor TacR1), oxytocin receptor (OxtR) on myoepithelial cells of the mammary gland, as well as in uterine myometrium and endometrium at the end of pregnancy [251]. Like GRK1, GRK5 connects to synuclein-α, -β, and -γ, recoverin, caveolin-1, and rhodopsin, in addition to calmodulin-1 and caldendrin (calcium-binding protein-1) [251]. Isoform GRK5 binds PI(4,5)P2 to tether to the plasma membrane [300].
5.2.11.6 GRK6
5.2.11.7 GRK7
Kinase GRK7 phosphorylates light-sensitive, membrane-bound G-protein-coupled receptors cone opsins in photoreceptors of the retina and initiates their deactivation. Membrane localization of GRK7 relies on its farnesylation [300].
5.2.12 Microtubule-Associated Ser/Thr Kinase Family
Microtubule-associated Ser/Thr kinases constitute a family with 5 known members (MAST1–MAST4 and related MASTL). Kinase MAST2 interacts with phosphatase and tensin homolog [300]. Microtubule-associated Ser/Thr kinase-like protein MASTL is the human ortholog of Greatwall kinase.
During the cell division cycle, mitotic regulator MASTL promotes mitotic entry, as it inhibits protein phosphatase-2 that dephosphorylates CcnB–CDK1 complex [356]. Inhibition of PP2 is mandatory for CcnB–CDK1 activation at mitotic entry. The CcnB–CDK1 activity is controlled during G2 phase by inhibitory phosphorylations (Thr14 and Tyr15) by kinases Wee1 and PKMYT1 membrane-associated Tyr–Thr protein kinase. Upon mitotic entry, activatory phosphatase CDC25 relieves this inhibition. Phosphatase PP2 also regulates CcnB–CDK1 activity, as kinases Wee1 and PKMYT1 and CDC25 phosphatase are PP2 substrates. At mitotic exit, the CcnB–CDK1 complex is again inhibited by the ubiquitin-dependent degradation of cyclin-B. This inactivation results from PP2 reactivation. G2 Arrest results from dephosphorylation of the CcnB–CDK1 complex as well as its inhibition by Wee1 and PKMYT1 in the absence of CDC25-induced dephosphorylation. The ability of cells to remain arrested in mitosis by the spindle assembly checkpoint is proportional to the MASTL amount. When MASTL is slightly reduced, cells arrest at prometaphase. More complete depletion in MASTL correlates with premature dephosphorylation of the CcnB–CDK1 complex, inactivation of the spindle assembly checkpoint, and subsequent exit from mitosis with severe cytokinesis defects. The balance between CcnB–CDK1 dimer and PP2 is tightly regulated for correct mitotic entry and exit by MASTL kinase [356].
5.2.13 P21-Activated Kinase Family
The P21 (Ras-like cyclin-dependent kinase inhibitor-1A)-activatedl kinases (PAK) serve as regulators that intervene in cytoskeletal organization as well as cell survival, apoptosis, and angiogenesis. The PAK proteins are effectors that link small GTPases to cytoskeleton reorganization and nuclear signaling.
The PAK family includes 6 known members (PAK1–PAK6). Members of the PAK family have been classified into 2 subfamilies: subfamily 1 (or A; PAK1–PAK3) and 2 (or B); PAK4–PAK6) that do not contain the auto-inhibitory domain of group-1 PAKs. Subfamily-2 PAKs are expressed in the brain, where they participate in locomotion as well as learning and memory [360].
P21-Activated kinases are effectors of the Rac and CDC42 GTPases. In fact, they are CKI1a-, CDC42-, and Rac1-activated kinases. Binding of active, monomeric GTPase RacGTP or CDC42GTP as well as sphingosine-related lipids causes conformational changes. The PAK proteins are then potently activated by autophosphorylation at multiple sites. However, PAK can be phosphoryled by other kinases [357].
On the other hand, Mg
–Mn
-dependent protein phosphatases of the family of protein Ser/Thr phosphatase-2C, the PP2C domain-containing protein phosphatases PPM1e and PPM1f,75 inactivates PAK [358]. Magnesium-dependent protein phosphatase PPM1a also inhibits PAK1 kinase. In addition, PP2 can form a complex with PAK1 and PKCζ to dephosphorylate troponin-I and -T in cardiomyocytes.


5.2.13.1 PAK1
Kinase PAK176 exists as an homodimer under basal conditions. The activity of PAK1 is governed by the phosphorylation–dephosphorylation cycle (Ser144) and binding of certain lipids, such as sphingosine, dihydro- and phytosphingosine, monosialoganglioside (GM3), phosphatidylinositol, lysophosphatidylinositol, dimyristoylphosphatidic acid, and phosphatidic acid [357]. Small GTPases CDC42, Rac1 to Rac3, RhoJ, RhoQ, and RhoU activate PAK1 by disrupting homodimerization and potentiating phosphorylation.
Isoform PAK1 participates in polarized cytoskeletal regulation, cell morphology, motility, survival, apoptosis, and transformation. It is located in regions of cytoskeletal activity (e.g., ruffles, lamellipodia, etc.) and focal adhesions [357]. Various active forms of PAK1 induce cytoskeletal reorganization by kinase- dependent and -independent effects, as they can also act as scaffolds that recruit adaptors NCK and GRB2 as well as guanine nucleotide-exchange factors RhoGEF6 and RhoGEF7. Kinase PAK1 attracts via these Rac–CDC42GEF the G-protein-coupled receptor kinase-interacting protein APAP177 that links PAK1 to focal adhesions via paxillin.
The PAK1 activity can rise upon binding of regulators, such as RhoGEF6 and − 7 and calcium- and integrin-binding protein (CIB) [357]. The PAK1 function is also enhanced by guanylate cyclase78 to promote cell migration and lamellipodium formation, Gβ/γ subunit of G protein for directional sensing and migration, and PI3K. Conversely, cytoskeletal protein Merlin,79 insulin receptor substrate partner nischarin,80 apoptosis mediator CDK11 kinase,81 estrogen-receptor signaling mediator cysteine-rich inhibitor of PAK1 (CRIPAK), and PAK-interacting protein PAK1IP1 (or PIP1) prevent PAK1 activity.
Substrates of PAK1 comprise myosin light-chain kinase (MLCK; inactivation) and LIM domain-containing protein kinase LIMK1 and LIMK2 (activation) [357] (Table 5.1). Phosphorylated MLCK cannot phosphorylate the myosin regulatory light chain, thereby impeding cell contractility. The LIMK kinases regulate cofilin cycling.
Other targets include cytoskeletal proteins, such as myosin-6, myosin heavy chains-1E and -2B, filamin-A, caldesmon, desmin, vimentin, regulators of microtubule dynamics stathmin and tubulin cofactor-B, dynein light chain-1, and linker of synaptic vesicles synapsin-1 [357]. Protein PAK1 operates in GTPase bearing, as it interacts with GTPase regulators and effectors, such as heterotrimeric G-protein subunit Gz, RhoGDIα, and RhoGEF2 and RhoGEF8, in addition to RhoGEF6 and -7, APAPs, and Merlin. Last but not least, PAK1 interacts with components of the MAPK module, i.e., cRaf, MAP3K1, and MAP2K1, as well as Aurora-A kinase and Polo-like kinase-1.
Protein PAK1 is phosphorylated by CDK1 and CDK5 (Thr212; inactivation) to modulate the microtubule dynamics and cell spreading, as well as PDK1 (Thr423; activation), PKG (Ser21) to inhibit NCK binding, PKB1 (Ser21; activation) via scaffold Arg protein Tyr kinase-binding protein ArgBP2, PKB2 (inhibition), BMX, and JAK2 (Tyr153, Tyr200, and Tyr284; activation) kinases [357].
Kinase PAK1 interacts with NCK adaptor to regulate endothelial cell migration, GRB2 to couple PAK1 to EGFR, and inhibitor ABI gene family member ABI3 [357].82
Target transcription factors consist of FoxO1, estrogen receptor-α (or NR3a1), epithelial-specific E74-like factor ELF3 of the ETS transcription factor family, Snai that can contribute to epithelial–mesenchymal transition, STAT5a, histone-3, SHARP that interacts with recombination signal-binding protein for immunoglobulin κJ region (RBPJ) and represses Notch pathway, and transcriptional repressor CtBP1.
In addition to small RhoA GTPase, PAK1 interacts with neurogenic differentiation NeuroD2 factor, A-kinase-anchoring protein AKAP9, phosphoinositide-dependent protein kinase PDK1, phospholipase-D1, coiled-coil domain-containing protein CCDC85b (or δ-interacting protein-A [DIPa]), pyruvate dehydrogenase kinase-1, light and heavy polypeptidic neurofilament (NeFL and NeFH), 62-kDa cerebellar degeneration-related protein CDR2, actinin-α1, and vimentin [251].
5.2.13.2 PAK2
Cardiolipin-activated PAK283 is activated by proteolytic cleavage during caspase-mediated apoptosis. It interacts with CDC42, SH3-domain kinase-binding protein SH3KBP1, and Abl kinase (Table 5.1).
Others partners include cytosolic protein Tyr phosphatase PTPn13, 3-phosphoinositide-dependent protein kinase PDK1, protein kinase-B, mitogen-activated protein kinase kinase kinase MAP3K2, monomeric RhoA GTPase, and NCK1 adaptor [251].
5.2.13.3 PAK3
Two kinases — PAK3 and SGK2 — intervene in viability and proliferation of epithelial cells, in particular, following P53 inactivation. Loss of P53, SGK2, or PAK3 alone has little effect on cell survival, whereas loss of P53 together with that of either SGK2 or PAK3 leads to cell death [362].
5.2.13.4 PAK4
Kinase PAK4 intervenes in filopodium formation. Multiple alternatively spliced transcript variants encode distinct isoforms. Protein PAK4 is an effector of CDC42 in the regulation of cytoskeleton reorganization. It may protect cells from apoptosis, preclude cell adhesion, and promote cell migration.
Protein PAK4 interacts specifically with CDC42GTP. It also targets β5-integrins and LIMK1 kinase. It weakly activates JNK kinases.
5.2.13.5 PAK5 or PAK7
Although PAK5, or PAK7, contains, like subfamily-1 PAKs, an auto-inhibitory domain, it does not possess, unlike subfamily-1 PAKs, a PAK inhibitor domain [359]. Moreover, it is activated by CDC42, but not as strongly as subfamily-1 PAK members. The Pak5 gene possibly belongs to a cluster of genes regulated by miR15a and miR16 agents.
Isoform PAK5 is predominantly expressed in the brain. It interacts with CDC42 to promote neurite outgrowth. It stabilizes the microtubule network. Isoform PAK5 localizes to mitochondria, where it has a strong pro-survival activity [363]. Upon growth factor stimulation or cell cycle progression, PAK5 transits from mitochondria to the nucleus. Its binding to CDC42 and RhoD triggers its relocalization.
Kinase PAK5 can be activated by MAP3K6, an activator of P38MAPK. On the other hand, PAK5 weakly interacts with activated Rac. In addition, PAK5 inhibits microtubule affinity-regulating kinase MARK2. In the mitochondrion, active phosphorylated PAK5 P can phosphorylate BAD, thereby promoting cell survival. PhosphoPAK5 not only phosphorylates BAD (Ser112), but also favors phosphorylation (Ser136) possibly by PKB [363].
5.2.13.6 PAK6
Isoform PAK6 is highly expressed in testis and prostate. It interacts with the androgen receptor (NR3c4), a steroid hormone-dependent transcription factor that operates in male sexual differentiation and development.
5.2.14 RoCK Kinase Family
Rho-associated, coiled-coil-containing protein kinases (RoCK1–RoCK2), also simply called Rho kinases, are effectors of Rho GTPases. The RoCK kinases consist of a N-terminal kinase domain, a coiled-coil region with a Rho-binding sequence (RBD), and pleckstrin homology (PH) motif with an internal cysteine-rich region (CRD) at the C-terminus. The auto-inhibitory region includes RBD and PH domains. Both domains can bind independently to N-terminal kinase region to inhibit the kinase activity [364]. The RoCK enzymes are dimers. Binding of RhoGTP induces a conformational change that relieves RoCK auto-inhibition by the C-terminus.
The RoCK proteins are implicated in cell adhesion, motility, growth, contraction, and cytokinesis. They are activated by autophosphorylation or RhoA (Sect. 9.3). Activation of RoCK, which depends on integrins, induces interaction with Rho and RoCK translocation to the plasma membrane. The RoCK kinases enhance the formation of focal adhesions and assembly of stress fibers. Moreover, RoCKs phosphorylate (inhibit) myosin light-chain phosphatase (MLCP) which dephosphorylates the regulatory light chain of myosin-2 (Fig. 5.1). Hence, RoCKs regulate the strength and the speed of actin–myosin cross-bridging in smooth muscle and non-muscle cells.


Fig. 5.1
RoCK kinase and protein phosphatase regulate the activity of myosin-2, the nanomotor in smooth muscle and non-muscle cells. Myosin-2 is regulated by phosphorylation and dephosphorylation of its myosin regulatory light chain (MRLC) by Ca
–calmodulin-regulated myosin light-chain kinase (MLCK) and myosin light-chain phosphatase (MLCP). Phosphorylated MRLC (pMRLC) activates actin–myosin-2 binding. Myosin light-chain phosphatase dephosphorylates MRLC, thus inducing relaxation. Activated small GTPase RhoA stimulates Rho kinase, which phosphorylates MLCP (pMLCP), thereby inhibiting MLCP activity and increasing MRLC phosphorylation, favoring contraction. The Rho–RoCK pathway contributes to the tonic phase of contraction in smooth muscle cells.

Some lipids, especially arachidonic acid, can activate RoCKs independently of Rho GTPase [364]. Protein oligomerization may also regulate RoCK activity, possibly via N-terminal transphosphorylation.
Kinase RoCK phosphorylates ezrin, radixin, and moesin proteins that are involved in interaction between actin filaments and the plasma membrane. It also phosphorylates LIMK kinases, thereby precluding cofilin-mediated actin filament disassembly.
Activated RoCK kinases by lysophosphatidic acid85 reduce the peak current amplitude ofCaV3.1 and CaV3.3 channels without affecting the voltage dependence of activation and inactivation [365]. They induce depolarizing shifts for activation and inactivation in CaV3.2 channels.
The RhoA–RoCK axis modulates ion channel activity through actin reorganization. It actually hampers the activity of several potassium channels, such asKV1.2 [366], KV11.1 [367], andinward rectifier KIR2.1 [368]. Moreover, RhoA and RoCK impede actin polymerization and vasoconstriction caused by uridine triphosphate. The latter provokes depolarization resulting from KV1.2 channel activity in smooth muscle cells of cerebral arterioles. Activated PKA or PKG reduce inhibition of KV1.2 activity by RhoA and RoCK [369]. In addition, RhoA mediates inhibition of RhoGDIα onCaV1 channels in ventriculomyocytes [370]. On the other hand, RhoA and RoCK increase the activity of epithelial sodium channel, as they activate phosphatidylinositol 4-phosphate 5-kinase and increase channel insertion into the plasma membrane [371]. They also enhance the functioning of volume-regulated anion channels in pulmonary artery endothelial cells [372].
Two isoforms — RoCK186 and RoCK2 —87 are encoded by the Rock1 and Rock2 genes.88 They can be differentially regulated according to circumstances.
Kinase RoCK1 is cleaved by caspase and activated during apoptosis, whereas RoCK2 can be cleaved and activated by granzyme-B89 to cause caspase-independent membrane blebbing.
Both paralogs participate in the regulation of cytokinesis, cell morphology, contraction, motility, and adhesion, as well as endothelial barrier function and phagocytosis, as they favor actin–myosin-mediated contractility. They also indirectly influence gene transcription as well as cell growth, proliferation, and survival. They thus contribute to neuronal morphogenesis, smooth muscle contraction, immune cell chemotaxis, and epithelial sheet movements.
The RoCK kinases phosphorylate various substrates, such as myosin light-chain phosphatase, myosin light chain, ezrin, radixin, moesin, and LIMK kinases to favor the formation of actin stress fibers and focal adhesions.
In smooth muscle cells, they assist agonist-induced muscle contraction by Ca
-sensitization that results from phosphorylation of myosin-binding subunit of myosin light-chain phosphatase.

They participate in cell migration by enhancing cell contractility. They are involved in tail retraction of monocytes. Last, but not least, they contribute to the control of cell size and regulation of distance between the 2 centrioles [364].
In non-myocytes, actomyosin contractility depends on the phosphorylation state of regulatory myosin light chain of nanomotor myosin-2, i.e., the balance between Ca
-dependent myosin light-chain kinase (MLCK) and phosphatase (MLCP). Kinase RoCK phosphorylates myosin light chain (Ser19) like MLCK, even in the absence of Ca
ion. Phosphorylation of myosin light chain leads to its interaction with actin that activates myosin ATPase and causes cell contraction. Phosphorylation of myosin-binding subunit (MBS or myosin phosphatase target subunit MyPT; Ser854, Thr697, and Thr855) of myosin light-chain phosphatase by RoCKs prevents the dephosphorylation of myosin light chain, thereby favoring stress-fiber formation and cell contractility [364].


In endothelial cells, RoCKs disrupt the integrity of both tight and adherens junctions, as they provoke contraction of stress fibers. They thus raise endothelial permeability. However, in epithelial cells, RoCK activation favors tight-junction integrity, hence enhancing barrier function. Nonetheless, activated RoCK may also disturb E-cadherin-mediated adherens junctions by initiating actomyosin bundle contraction.
Small GTPases of the RHO superfamily (CDC42, Rac1, and RhoA to RhoC), under the control of specific guanine nucleotide-exchange factors (RhoGEF) and GTPase-activating proteins (RhoGAP),90 regulate angiogenesis, as they intervene in extracellular matrix remodeling, cell migration, proliferation, and survival, and morphogenesis. Vascular endothelial growth factor activates RhoA GTPase that intervenes in endothelial migration during angiogenesis. In general, morphogenesis of a duct network depends on the frequency and geometry of branchings, hence on the control of endothelial sprouting (i.e., initiation of new branches from existing vessels in response to chemical messengers).91 The RoCK kinases limit endothelial sprouting and heightens the length of vessels formed upon VEGF stimulation [374]. In addition to elevated matrix stiffness, the activity of myosin-2 supported by RoCK enzymes prevents endothelial sprouting, as they lower the activation of VEGFR2 receptor [375].92 Myosin-2 localizes to the cortex of endothelial cells. Local depletion of cortical myosin-2 precedes pseudopodium, hence branch initiation [375]. The RhoA–RoCK axis determines branching sites of tip cells by regulating tension in the cortex of endothelial cells. On the other hand, RhoA at the trailing edge promotes tail retraction to assist migration of stalk cells [373] (Table 5.11). In addition, RoCK kinase prevents activity of endothelial nitric oxide synthase, hence that of NO in the development and maintenance of the microvasculature [373].
RhoA–RoCK | Regulation of tension in the cortex of endothelial cells, |
---|---|
inhibition of tip cell protrusion, | |
hence of vascular sprouting | |
Trailing edge retraction, hence supporting | |
migration of stalk cells | |
VEGFR2–RoCK | Rho-independent activation of RoCK enzymes |
Negative feedback exerted by RoCK1/2 on VEGFR2 |
5.2.14.1 RoCK1 Kinase
Ubiquitous Rho-activated protein kinase RoCK1 has a slightly higher expression in the human brain, spinal cord, retina, and lung [376]. Kinase RoCK1 is located in the cytoplasm, accumulating particularly in the perinuclear region and Golgi body. It colocalizes with actin stress fibers. It associates with centrosomes. It can contribute to centrosome positioning and centrosome-dependent exit from mitosis [376].
The major cellular function of RoCK1 is the regulation of cell morphology by controlling functioning of actin–myosin and intermediate filaments. Isokinase RoCK1, but not RoCK2, is inhibited by RhoE and Gem GTPases [376] (Sects. 9.3.25 and 9.3.26). Isoform RoCK1 could play a more important part in the regulation of the actin–myosin cytoskeleton and phagocytosis, as it differs from RoCK2 in phosphatidylinositol binding and subcellular localization.
The activity of RoCK1 is regulated by binding of RhoAGTP, RhoBGTP, or RhoCGTP via 3 sequences: Rho-binding domain (RBD), Rho-interacting domain (RID), and possibly a leucine zipper homology region HR1 [376]. Activation can also result from caspase-mediated proteolysis that generates an active fragment lacking the C-terminal auto-inhibitory domain. Cyclin-dependent kinase inhibitor CKI1a hinders RoCK1 activity, when CKI1a is located in the cytoplasm.
Substrates of RoCK1 comprise RhoE and PTen phosphatase. Kinase RoCK1 also phosphorylates LIMK1 (Thr508) and LIMK2 (Thr505), thereby enhancing the ability of LIMKs to phosphorylate actin-depolymerizing cofilin (Ser3). Phosphorylation by RoCK of MLCP, MLC, and LIMK heightens actin-filament assembly and actomyosin filament contraction. Kinase RoCK1 also interacts with RhoA and RhoE GTPases, cofilin-1, profilin-2, GRB2-associated-binding protein GAB1, and CDC25a phosphatase [251]. Isoform RoCK1 phosphorylates (activates) Na + –H + exchanger NHE1 (SLC9a1). It participates in RhoA-induced reorganization of actin filaments. It also binds to ezrin, radixin, and moesin to contribute to fibroblast migration [364].
5.2.14.2 RoCK2 Kinase
Ubiquitous RoCK2 is the main type in smooth muscle cells and neurons. It is activated by association with RhoGTP. Kinase RoCK2 stabilizes filamentous actin and enhances myosin ATPase activity. It promotes the formation of contractile actin–myosin bundles (stress fibers) and integrin-containing focal adhesions. It phosphorylates (inhibits) myosin light-chain phosphatase, and thus increases myosin-2 activity even at a constant intracellular calcium level (calcium sensitization ), and causes sustained vasoconstriction and bronchospasm.
Kinase RoCK2 localizes in the cytoplasm, especially close to the plasma membrane and in the perinuclear region. Linkage to RhoGTP primes its translocation to membranes. In some circumstances such as growth factor stimulation, RoCK2 translocates to the nucleus, where it phosphorylates P300 acetyltransferase and CDC25a phosphatase [377]. During late mitosis, it localizes with vimentin (i.e., intermediate filaments) at the cleavage furrow.
Like RoCK1, RoCK2 is regulated by binding of GTP-loaded RhoA, RhoB, or RhoC. Activation also happens by granzyme-B-mediated proteolysis that generates an active fragment lacking the C-terminal auto-inhibitory motif [377] (RoCK2
). Furthermore, binding of PI(3,4,5)P3 or PI(4,5)P2 to the pleckstrin homology domain heightens RoCK2 activity. Cyclin-dependent kinase inhibitor CKI1a, when it resides in the cytoplasm, hinders RoCK activity. Kinase cRaf, particularly after stimulation of the pro-apoptotic TNFRSF6a pathway, inhibits RoCK2 enzyme.

Kinase RoCK2 also associates with activator arachadonic acid and multiple cytoskeletal proteins and their regulators, such as myosin regulatory light chain, myosin-binding subunit of the myosin light-chain phosphatase, smooth muscle-specific PP1r14 inhibitory subunit of myosin phosphatase, calcium-binding myosin-ATPase inhibitor calponin-1, plasma membrane–cytoskeleton connectors ezrin, radixin, and moesin, actin-binding protein profilin-2, α-adducin (Add1), plasmalemmal actin-filament crosslinker myristoylated alanine-rich protein kinase-C substrate (MARCKS), LIMK1 and LIMK2, the sarcomeric and intermediate filament constituents desmin and vimentin, cardiac troponin-I and -T, neurofilament light polypeptide, astrocyte-specific intermediate filament protein glial fibrillary acidic protein (GFAP), dihydropyrimidinase-like protein Dpysl293 that modulates RhoA-induced neuronal morphology [377].94
Adducin is a filamentous actin-binding protein that promotes spectrin–actin assembly beneath the plasma membrane. Phosphorylation of α-adducin by RoCK2 enhances linkage of adducin to actin filaments. α-Adducin phosphorylated by RoCK2 can be dephosphorylated by MLCP. Ezrin–radixin–moesin complexes crosslink actin filaments to plasmalemmal proteins. Phosphorylation of radixin by RoCK2 (Thr564) disrupts the interaction between its N- and C-termini [364]. Like protein kinase-Cθ, RoCK2 can phosphorylate moesin (Thr558), thereby antagonizing MLCP. Kinase RoCK2 phosphorylates several Factin-binding proteins to decrease their actin-binding capacity, such as smooth muscle calponin-1 that hampers actin-activated myosin ATPase activity.
Vimentin, glial fibrillary acidic protein, and neurofilament light protein are intermediate-filament proteins that can be phosphorylated by various kinases. Vimentin phosphorylation induces filament disassembly.
Additional partners include STK1695 and IKKε [251]. Other RoCK2 substrates encompass eukaryotic translation elongation factor eEF1α1 expressed in the brain, lung, liver, kidney, pancreas, and placenta and responsible for the enzymatic delivery of aminoacyl tRNAs to the ribosome, PTen, and Na + –H + exchanger isoform-1 [377].
5.2.15 Other Members of the ROCK Family
In addition to RoCK kinases, members of the ROCK family include citron Rho-interacting kinase (CRIK), myotonin protein kinase (DMPK), and myotonic dystrophy kinase-related CDC42-binding kinase (MRCK).96 They control the cytoskeleton, hence cell shape and motility (Sect. 9.3.12).
Kinase CRIK can bind RhoGTP; DMPK interacts with RacGTP; and MRCK connects to diacylglycerol that provokes a conformational change allowing its binding to CDC42GTP [300]. Kinase RoCK is also activated in apoptotic cells by cleavage of its auto-inhibitory C-terminus by caspase-3. The hydrophobic motif is mainly aimed, at least in RoCK, at promoting dimerization.
5.2.16 P90 Ribosomal S6 Kinase Family
Ribosomal protein S6 kinases constitute a family of enzymes that may be considered as belonging to distinct superfamilies of protein Ser/Thr kinases, the AGC and CAMK superfamilies (Table 5.12) [378]. Kinase isoforms RSKi (i = 1, …, 4) and MSKi (i = 1, …, 2) contain 2 kinase domains, a N-terminal (NTK) and a secondary C-terminal (CTK) kinase motif that pertain to the AGC and CAMK superfamily, respectively [379]. The CTK domain activates the NTK sequence. The latter phosphorylates substrates.
Table 5.12
Ribosomal protein S6 kinases (RPS6K) of the AGC superfamily. Ubiquitous kinase isoforms RSKi (i = 1, …, 4; RSK: 90-kDa ribosomal S6 kinase) and MSKi (i = 1, …, 2; MSK: mitogen- and stress-activated protein kinase) of the AGC superfamily. The RSK proteins, or MAPKAPK1s, and MSK work in signal transduction downstream from MAPK cascades. The former is activated by members of the extracellular signal-regulated kinase (ERK) family in response to growth factors, many polypeptidic hormones, neurotransmitters, chemokines, and other stimuli. The latter is activated by ERKs in response to same stimuli as well as P38MAPKs in response to various cellular stresses and pro-inflammatory cytokines.
Name | Alias | Other alias |
---|---|---|
RPS6K, 52kDa, polypeptide-1 | RPS6Kδ1 (RPS6Kc1) | RSKL1 |
RPS6K-like 1 | RPS6KL1 | RSKL2 |
RPS6K, 70kDa, polypeptide-1 | RPS6Kβ1 | S6K1 |
RPS6K, 70kDa, polypeptide-2 | RPS6Kβ2 | S6K2 |
RPS6K, 70kDa, polypeptide-3 | RPS6Kβ3 | S6K3 |
RPS6K, 90kDa, polypeptide-1 | RPS6Kα1 | RSK1 |
RPS6K, 90kDa, polypeptide-2 | RPS6Kα2 | RSK3 |
RPS6K, 90kDa, polypeptide-3 | RPS6Kα3 | RSK2 |
RPS6K, 90kDa, polypeptide-4 | RPS6Kα4 | RSKb, MSK2 |
RPS6K, 90kDa, polypeptide-5 | RPS6Kα5 | RLPK, MSK1 |
RPS6K, 90kDa, polypeptide-6 | RPS6Kα6 | RSK4 |
The MAPK-activated protein kinases (MAPKAPK) that respond to extracellular stimuli on MAPK-induced phosphorylation (activation) constitute a category with 5 MAPKAPK subfamilies of [380]: (1) P90 ribosomal S6 kinase (RSK1–RSK4); (2) mitogen- and stress-activated kinase (MSK1–MSK2); (3) MAPK-interacting kinases (MNK1–MNK2); (4) MAPK-activated protein kinase-2 and -3 (MK2–MK3);97 and (5) MK5.98 These enzymes are involved in regulation of nucleosome and gene expression, mRNA stability and translation, and cell proliferation and survival (Table 5.13).
Table 5.13
Activation pathways and effects of P90 ribosomal S6 kinases (RSK) and mitogen- and stress-activated kinases (MSK) that constitute 2 MAPK-activated protein kinase (MAPKAPK) sets (Source: [380]).
Stimuli | Pathways | Effects |
---|---|---|
RSK1–RSK4 | ||
Cell stresses | MAP3K2/3/8–MAP2K5–ERK4(5) | Gene transcription, |
Growth factors | MAP3K2/3/8–MAP2K5–ERK4(5) | cell survival, growth, |
a/b/cRaf, Mos–MAP2K1/2–ERK1/2 | and proliferation | |
Mitogens | a/b/cRaf, Mos–MAP2K1/2–ERK1/2 | |
MSK1–MSK2 | ||
Growth factors, | a/b/cRaf, Mos–MAP2K1/2–ERK1/2 | Gene transcription, |
mitogens | nucleosomal response | |
Cytokines, | MAP3K1–5/7/8/10/12/17/18– | |
cell stresses | –MAP2K3/6–P38MAPK | |
MNK1–MNK2 | ||
Growth factors, | a/b/cRaf, Mos–MAP2K1/2–ERK1/2 | mRNA translation |
mitogens | ||
Cytokines, | MAP3K1–5/7/8/10/12/17/18– | |
cell stresses | –MAP2K3/6–P38MAPK | |
MK2–MK3 (MAPKAP2–MAPKAP3) | ||
Cytokines, | MAP3K1–5/7/8/10/12/17/18– | Gene transcription, |
cell stresses | –MAP2K4/7–JNK1–3 | actin remodeling, |
cytokine synthesis, | ||
cell cycle control, | ||
cell migration | ||
MK5 (MAPKAP5) | ||
ER2K3/4–ERK3/4 | Actin remodeling |
Ribosomal protein S6 kinases possess 2 non-identical kinase domains to phosphorylate S6 ribosomal protein, hence to increase protein synthesis and favor cell proliferation. They can phosphorylate various substrates, such as eukaryotic translation initiation factor eIF4b (by RPS6Kβ2 [or S6K1]), members of mitogen-activated protein kinase modules (by members of the RPS6Kα [RSK] set), as well as transcription factors CREB1 and Fos (by RPS6Kα4 [or RSKb]).
Ribosomal protein S6 kinase-δ199 binds to sphingosine kinase-1, phosphatidylinositol 3-phosphate, and anti-oxidant peroxiredoxin Prdx3 (thioredoxin-dependent peroxide reductase) [381, 382]. It contains a phox homology (PX) motif and 2 pseudokinase domains. On early endosomes, RPS6Kδ1 colocalizes with sphingosine kinase-1, which synthesizes lipid messenger sphingosine 1-phosphate. It is implicated in sphingosine 1-phosphate-mediated signaling.
5.2.16.1 P90RSK Family
The family of 90-kDa protein Ser/Thr ribosomal S6-kinases regulates cell growth, survival, proliferation, and motility. The RSK family consists of 4 isoforms (RSK1–RSK4) and 2 structurally related homologs, RSK-like protein kinase or mitogen- and stress-activated kinase-1 (RLPK or MSK1) and -2 (RSKb or MSK2; Table 5.14).100
Table 5.14
Members the RSK family of the AGC superfamily. Kinases MAPKAPK2, -3, and -5 pertain to the CAMK superfamily. Five MAPKAPK subfamilies include the RSK, MSK, MINK, MK2, and MK5 groups (MK [MAPKAPK]: mitogen-activated protein kinase-activated protein kinase; MNK [MINK]: MAPK-interacting protein serine/threonine kinase, or MAPK signal-integrating kinase; MSK: nuclear mitogen-and stress-activated protein kinase; RPS6K [RSK]: ribosomal protein S6 kinase; NA: not applicable).
Gene | Protein | Alias in MAPK module |
---|---|---|
RSK subfamily — RPS6K | ||
RPS6KA1 | RSK1 | MAPKAPK1a, RPS6Kα1 |
RPS6KA2 | RSK3 | MAPKAPK1c, RPS6Kα3 |
RPS6KA3 | RSK2 | MAPKAPK1b, RPS6Kα2 |
RPS6KA6 | RSK4 | NA |
MSK subfamily — RPS6K | ||
RPS6KA4 | RSKb | MSK2, RPS6Kα5 |
RPS6KA5 | RLPK | MSK1, RPS6Kα4 |
Other subfamilies of MAPK-activated protein kinases | ||
(members of the CAMK superfamily) | ||
MINK subfamily | ||
MKNK1 | MNK1 | |
MKNK2 | MNK2 | |
Mapkapk2 | MK2 | MAPKAPK2 |
Mapkapk5 | MK2 | MAPKAPK2 |
The P90 ribosomal S6-kinases act downstream from the Ras–ERK pathway in response to growth factors, peptidic hormones, neurotransmitters, and chemokines. Ligand-bound receptors cause autophosphorylation and create docking sites for adaptors, such as growth factor receptor-bound protein GRB2 that links the receptor to the guanine nucleotide-exchange SOS factor. Son of sevenless stimulates Ras GTPase that recruits Raf kinases to the plasma membrane for activation. Activated Raf phosphorylates both MAP2K1 and MAP2K2 enzymes. Activated MAP2Ks then phosphorylate extracellular signal-regulated kinases ERK1 and ERK2. The P90 ribosomal S6-kinases are targeted not only by ERK1 and -2, but also by 3-phosphoinositide-dependent kinase PDK1.101 In dendritic cells, RSKs can be activated also by P38MAPK. On the other hand, protein phosphatase-2Cδ binds to and dephosphorylates RSKs. Phosphorylation mediated by ERK1 and ERK2 of PPM1d dissociates the RSK–PPM1d complex.
Inactive RSKs are located in the cytoplasm and nucleus, whereas activated RSKs remain linked to the plasma membrane, in the cytosol, or translocate to the nucleus. Phosphorylation of RSK occurs at the plasma membrane and in the cytoplasm and nucleus [383]. In addition, a fraction of activated ERKs and RSKs enters the nucleus to prime immediate-early gene expression. Nevertheless, constitutively active RSK4 is predominantly cytosolic.
RSKs regulate various transcription factors, such as cAMP-responsive element-binding protein, serum response factor, ETS transcription factor ER81, estrogen receptor-α, nuclear factor-κB, nuclear factor of activated T-cell NFAT3, cytoplasmic, calcineurin-dependent NFAT4, and transcription initation factor TIF1A2. RSKs also phosphorylate eukaryotic translation initiation factor-4B that stimulates heterotrimeric eIF4f102 and promotes interaction between eIF4b and eIF3 proteins.
In addition, RSKs favors cell survival by inactivating some pro-apoptotic proteins such as BCL2 antagonist of cell death (BAD), death-associated protein kinase (DAPK), whereas it phosphorylates CCAAT/enhancer binding protein-β that inhibits caspases [383]. Both RSK1 and RSK2 also regulate the cell division cycle, as they phosphorylate cyclin-dependent kinase inhibitor CKI1b for 14-3-3-mediated cytosolic sequestration, thereby promoting G1-phase progression.103 Kinase RSK1 phosphorylates (inactivates) nitric oxide synthase-1. Kinase RSK2 phosphorylates Na + –H + exchanger isoform NHE1, which regulates intracellular pH. Like SGK1, PKB, and AMPK, RSK phosphorylate PKB substrate AS160 that mediates translocation of glucose transporter GluT4 to the plasma membrane.
Inactive RSK1 is able to bind to the PKA regulatory subunit to attenuate the interaction between the regulatory and catalytic subunits. Activated RSK1 associates with PKA catalytic subunit and reduces the ability of cAMP to stimulate PKA [383]. Interaction between RSK1 and PKA allows RSK1 colocalization with A-kinase-anchoring proteins, whereas PKA–AKAP interaction supports nuclear accumulation of active RSK1.
RSK1, -2, and -3 are ubiquitously expressed, but with tissue-specific concentrations [383]. RSK1 is predominantly expressed in kidney, lungs, and pancreas. Both RSK2 and RSK3 are highly produced in skeletal muscle, heart, and pancreas. In the brain, RSK1 is mainly synthesized in the cerebellum; RSK2 in the cerebellum and occipital and frontal lobes; and RSK3 in the medulla. Defective RSK2 gene causes Coffin-Lowry syndrome. Aberrant RSK activation has been detected in some cancer types.
Mitogen- and stress-activated kinases activated by ERK1 and ERK2 as well as P38MAPK (but not PDK1) phosphorylate transcription factors cAMP-responsive element-binding protein and activating transcription factor ATF1, as well as histone-3. Mitogen- and stress-activated kinases participate in full stimulation of several immediate-early genes, such as Fos, JunB, MAPK phosphatase MKP1, and NURR1.
During evolution, a unique feature of kinases of the RSK family arises from fusion of genes for 2 distinct protein kinases generating a kinase with a C-terminal kinase domain (CTKD) that receives an activating signal from ERK and transmits this cue to the N-terminal kinase domain (NTKD).
5.2.16.2 RSK1 Kinase
Kinase RSK1104 is expressed in many tissues, with higher levels in the brain, lung, kidney, liver, pancreas, skeletal muscle, spleen, and thymus. It resides in the cytoplasm. Upon mitogenic stimulation, it moves toward the plasma membrane, where it accumulates transiently, and then translocates into the nucleus, where it phosphorylates several substrates involved in gene transcription [384].
Enzyme RSK1 phosphorylates ribosomal protein-S6 in response to activated Ras–ERK pathway. It regulates several transcription factors, such as SRF, Fos, NFAT4, ETS variant gene product ETV1, and microphthalmia-associated transcription factor (MiTF), as well as testicular receptor TR3 (or nuclear receptor NR4a1 of the NUR family [NuR77]) and estrogen receptor-α (NR3a1) [384]. It also interacts with transcriptional coactivator CREB-binding protein (CBP), cyclin-dependent kinase inhibitor CKI1b, Na + –H + exchanger NHE1, and GSK3 kinase. In response to mitogenic signaling, RSK1 phosphorylates (inhibits) nitric oxide synthase NOS1. Kinase RSK1 also regulates hamartin (tuberous sclerosis-1) TSC1 and tuberin (tuberous sclerosis-2) TSC2, ribosomal protein-S6, calcium–calmodulin-dependent eukaryotic elongation factor-2 kinase eEF2K, STK11 (or LKb1), a kinase of AMPK, and translation initiation factor eIF4b. Therefore, RSK1 participates in cell growth and proliferation. Kinase RSK1 also phosphorylates filamin-A, hence contributing to cytoskeleton reorganization. Other partners include protein kinase PKA, transducer of HER2 TOB1, and calcium-regulated heat stable protein-1 (CaRHSP1) [251].
Protein RSK1 controls cell survival, as it phosphorylates (inactivates) pro-apoptotic protein BAD and death-associated protein kinase (DAPK). It also targets transcription factorsCCAAT/enhancer-binding proteins C/EBPβ and nuclear factor-κB [384].
Kinase RSK1 as well as ERK1 and ERK2 form an inactive complex in quiescent cells. Upon mitogenic stimulation, ERK1 and ERK2 phosphorylate RSK1, that, in turn, autophosphorylates to create a docking site for phosphoinositide-dependent protein kinase PDK1 [384]. On the other hand, RSK1 is sequestered by 14-3-3β protein.
5.2.16.3 RSK2 Kinase
Kinase RSK2105 is implicated in the control of cell growth and differentiation. Like RSK1, RSK2 phosphorylates ribosomal protein-S6 in response to mitogenic stimulation of the Ras–ERK pathway.
Enzyme RSK2 interacts with CREB-binding protein transcriptional coactivator and histone acetyltransferase (CBP), ERK1, ERK2, P38MAPKα, 3-phosphoinositide-dependent protein kinase PDK1, and death effector domain-containing phosphoprotein enriched in astrocytes PEA15 [251].
5.2.16.4 RSK3 Kinase
Kinase RSK3106 is predominantly expressed in the lung, heart, skeletal muscles, and pancreas [385]. Three RSK3 splice variants exist (RSK3a–RSK3c).
Enzyme RSK3 intervenes during cell cycle progression [385]. It translocates to the nucleus during G0–G1 transition. It phosphorylates Fos and histones H1, H2A, H2B, H3, and H4.
Kinase RSK3 contains 6 phosphorylation sites. Maximal activation of growth factor-stimulated RSK3 requires the participation of both ERK1 and ERK2 and PDK1 kinases. Inactive RSK3 forms a complex with ERK1 and ERK2 and remains associated with these active ERKs following mitogen stimulation [385]. It interacts with PPM1d phosphatase. Kinase RSK3 also phosphorylates protein phosphatase PP1 and ribosomal 40S subunit. Lastly, RSK3 interacts with CREB-binding protein [251].
5.2.16.5 RSK4 Kinase
Kinase RSK4107 resides in many tissues, with high levels in fetal tissues as well as adult brain and kidney [384].
Enzyme RSK4 participates in P53-dependent growth arrest. It also promotes protein synthesis. In addition, RSK4 acts as an inhibitor of receptor Tyr kinase signaling during embryogenesis [384].
Kinase RSK4 possesses 6 phosphorylation sites.108 Activity of RSK4 is constitutively high even in the absence of growth factor stimulation [384]. Mitogenic stimulation further heightens RSK4 activity.
Protein RSK4 can associate with its activating extracellular signal-regulated kinases ERK1 and ERK2 via a docking (D) domain near its C-terminus [384]. It interacts with PPM1d phosphatase. In addition, it phosphorylates ribosomal protein S6 peptide and glycogen synthase kinase GSK3β. It also interacts with the nuclear receptor NR4a1 [251].
5.2.16.6 Kinases RSKL1 and RSKL2
Ribosomal S6 kinase-like proteins RSKL1 and RSKL2 are classified into group-E pseudokinases, as they lack the essential catalytic motifs in the kinase domain (Vol. 3 – Chap. 8. Receptor Kinases and Sect. 3.4). Pseudokinase domains of RSKL1 are used to bind sphingosine kinase-1 as well as peroxiredoxin-3 [300]. Isoform RSKL1, but not RSKL2, can interact with PI(3)P, and hence localize in early endosomes. In addition, RSKL1 and RSKL2 can connect to microtubules and intervene in intracellular transfer of cargos.
5.2.17 P70 Ribosomal S6 Kinase Family
The family of 70-kDa Ser/Thr ribosomal S6 kinases of the AGC superfamily includes S6K1 and S6K2 that are encoded by the RPS6KB1 and RPS6KB2 genes, respectively.
Both paralogs are ubiquitous. They participate in the regulation of cell metabolism, survival, and growth. These monomeric kinases are activated in cardiac, skeletal, and smooth muscle in response to a hypertrophic stimulus. Both paralogs have a catalytic activity that is controlled via multisite phosphorylation.
Activity of S6Ks is controlled by a set of phosphorylations of their activation segment and hydrophobic motif in response to insulin and growth factors. They are activated by target of rapamycin, a signaling node for many pathways that mainly rely on PI3K, PTen, and PKB enzymes. The S6K isoforms are phosphorylated (Thr389) by TORC1 complex using proline-rich Akt (PKB) substrate of 40 kDa (PRAS40), a TORC1 inhibitor, and tuberous sclerosis complex TSC2 and afterward by PDK1. As their name suggests, they phosphorylate 40S ribosomal protein subunit-S6 (RPS6). Ribosomal S6 kinases S6K1 and S6K2 augment protein synthesis at ribosomes and favor cell proliferation. Both isoforms have also been shown to phosphorylate eukaryotic translation initiation factor eIF4b [386].
5.2.17.1 S6K1
Paralog S6K1109 possesses 2 isoforms encoded by alternatively spliced transcript variants, the short (S6K1 S or P70S6K1) and long (S6K1 L or P85S6K1) splice variants [386].
Kinase S6K1 is activated by various growth factor pathways. In addition, it is sensitive to intracellular nutrient conditions. A sudden increase in cellular amino acid level primes S6K1 activation, whereas a lowered level precludes S6K1 activity. Similarly, when ATP levels drop, S6K1 is inhibited [386].
Glycogen synthase kinase-3 and target of rapamycin cooperate to control the activity of S6K1 [387].
Paralog S6K1 phosphorylates programmed cell death protein PDCD4, an inhibitor of eIF4a and eIF4g interaction. Moreover, it phosphorylates TOR (retrograde signaling). Kinase S6K1 can directly influence cell growth by acting on its specific substrates, such as S6K1-specific Aly/REF-like target (SKAR) that is also known as polymerase DNA-directed, δ-interacting protein PolDIP3 or PDIP46, a nuclear protein that intervenes at mRNA processing stage [386]. Under certain circumstances, S6K1 can phosphorylate glycogen synthase kinase GSK3 and BCL-2 associated death promoter (BAD; inhibition). Insulin-stimulated S6K1 phosphorylates (inactivates) insulin receptor substrate IRS1 (negative feedback loop). Additional S6K1 interactors include PDK1, small GTPases CDC42 and Rac1, the eIF3 complex, and isoforms PKCζ and PKCι.
5.2.17.2 S6K2
Messenger RNA of S6K2 generates 2 isoforms: S6K2S and S6K2L. Unlike S6K1, S6K2 contains an additional C-terminal nuclear localization signal [386]. Paralog S6K2 is regulated by many S6K1 interactors (PI3K, PKB, PKCζ, Rac1, and CDC42). However, S6K2 has unique functions that can complement those of S6K1 kinase. It forms a complex with bRaf and PKCε upon signaling launched by fibroblast growth factor FGF2. The extracellular-signal-regulated kinase pathway can activate S6K2.
5.2.18 Serum- and Glucocorticoid-Regulated Kinase Family
Serum- and glucocorticoid-regulated kinases constitute a family of 3 members: SGK1 (or SGK), SGK2 (or hSGK2); and SGK3 (a.k.a. CISK and SGKL). They can activate certain potassium, sodium, and chloride channels. Kinases SGK1 and SGK2 have a single identifiable functional domain, its catalytic domain, but SGK3 possesses a phosphoinositide-binding Phox homology (PX) domain at its N-terminus that interacts with phosphatidylinositol 3-phosphate to mediate the endosomal association of SGK3, which is essential for its phosphorylation and activation by PDK1 kinase.
Kinase SGK1 can stimulate sodium transport into epithelial cells, as it enhances the density and stability of epithelial sodium channels. It may also have overlapping functions with PKB isoforms. Isoform SGK1 is phosphorylated (Ser422) by TORC2 complex, then by PDK1 kinase [300].
5.3 Diacylglycerol Receptors and Kinases
There are many diacylglycerol receptors (DAGR): protein kinase-C (PKC) and -D (PKD),110 chimaerins,111 and others.
Receptors for activated C-kinase (RACK) bind to and position newly activated PKCs to discrete cellular locations. Peptides associated with RACK–PKC interaction act as translocation activators or inhibitors. Both PKCδ and PKCε are implicated in the evolution of the cardiac function after myocardial infarction [390]. They are also implicated in vasculogenesis. Enzymes PKCα and PKCε control integrin signaling to extracellular signal-regulated protein kinase [391].
The family of protein Ser/Thr kinase-D includes PKD1 (or PKCμ), PKD2, and PKD3 (or PKCν). They are located in the cytosol, nucleus, Golgi body, and plasma membrane.
Diacylglycerol kinases112 phosphorylate diacylglycerol to stop its signaling and produce phosphatidic acid. They then modulate PKC signaling because protein kinase-C is activated by diacylglycerol, and Ras signaling, as well as protein recruitment to membrane domains.
5.4 CAMK Superfamily
5.4.1 Calmodulin-Dependent Protein Kinase (CamK) Family
Calmodulin-dependent Ser/Thr protein kinases (CamK) require activated calmodulin for their activity. Like protein kinases PKA and PKC, CamK phosphorylates nitric oxide synthase (Sect. 10.3.1) [392].
Two categories of CamKs include: (1) specialized CamKs such as myosin light-chain kinase that phosphorylates myosin for muscle contraction and (2) multifunctional CamKs such as type-1 and -4 calmodulin-dependent protein kinases. Members of the CamK family include CamK1, CamK2, and CamK4 that are encoded by the genes of the CAMK1 (CAMK1, CAMK1D, and CAMK1G), CAMK2 (CAMK2A, CAMK2B, CAMK2D, and CAMK2G), and CAMK4 subfamily, respectively (Table 5.15).
Table 5.15
Calmodulin-dependent protein kinases. Alternatively spliced transcript variants encode distinct isoforms. Disc large homolog DLg1 is a member of the membrane-associated guanylate kinase family of scaffolds that control clustering of postsynaptic ion channels in neurons and in adherens junction formation in epithelial cells. Regulatory subunit-1 (P35) of cyclin-dependent kinase-5 (CDK5r1) is a neuron-specific CDK5 activator. Synapsins regulate neurotransmitter release at synapses. Small GTPase Ras-associated with diabetes (Rad) inhibits CamK2 in cardiomyocytes (CMC). Phospholamban inhibits sarcoplasmic reticulum Ca
ATPase SERCA. In cardiomyocytes, CamK2 has both positive inotropic and lusitropic effects.

Gene | Isoforms | Distribution | Interaction |
---|---|---|---|
CAMK1 | Many types | Cam2K; synapsins; CFTR; CREB, ATF1 | |
CAMK1D | |||
CAMK1G | |||
CAMK2A | 2 | Neuron | Actinin-α4, DLg1, CDK5r1 |
CAMK2B | 8 | Neuron | Actinin-α4 |
CAMK2D | 3 | Many types | Phospholamban, RyR, CaV1 |
CMC | HDAC4 | ||
CAMK2G | 6 | Many types | Rad, phospholamban |
CMC | RyR and CaV1 | ||
CAMK4 | T lymphocyte | Cam2K, PP2 | |
Neuron | |||
Male germ cell |
Calmodulin-dependent protein kinases contain catalytic, regulatory, and association domains. These enzymes assemble into a dodecameric holoenzyme. Several CamKs can aggregate into a homo- or hetero-oligomer. In the absence of Ca
–calmodulin, the catalytic domain is auto-inhibited by the regulatory domain. Upon activation by Ca
–calmodulin, activated CamK autophosphorylates. To gain maximal activity, CamK1 and -4 can be further phosphorylated by calmodulin-dependent protein kinase kinase (Cam2K).113 Two distinct Cam2Ks exist: Cam2Kα and Cam2Kβ.114


5.4.1.1 Calcium–Calmodulin-Dependent Protein Kinase-1
Calcium–calmodulin-dependent protein kinase-1 is expressed by many cell types. Kinase CamK1 phosphorylates many substrates, such as synapsin-1 and -2, cystic fibrosis transmembrane conductance regulator, and transcription factors CREB and ATF1. Isozyme CamK1 is involved in angiotensin-2 and K + stimulation of aldosterone synthase gene CYP11B2 transcription in the adrenal glomerulosa to produce aldosterone [394]. Subtype CamK1 activation cascade involves calcium–calmodulin and Cam2K. Protein kinase-A phosphorylates (inactivates) Cam2K [395]. Kinase Cam2K is also phosphorylated by CamK1 (negative feedback). Regulation by PKA of the Cam2K–CamK1 cascade can modulate the balance between cAMP and Ca
signals.

5.4.1.2 Calcium–Calmodulin-Dependent Protein Kinase-2
Calcium–calmodulin-dependent protein kinase-2 phosphorylates a large number of substrates, such as ion channels and intracellular mediators of signal transduction. For example, it increases PLA2 activity. The canonical activation of CamK2 requires Ca
influx and calmodulin binding. However, CamK2 can be activated by oxidation.

Kinase CamK2 is made of catalytic, regulatory, and binding domains to form oligomers and act on its targets. Four isoforms of CamK2 (CamK2α–CamK2δ) are encoded by different genes. Isoforms CamK2α and CamK2β are almost exclusively produced in the central nervous system, where they intervene in synaptic evolvability, whereas CamK2γ and CamK2δ are more ubiquitous. Numerous splice variants have been recognized: 13 for CamK2γ and 15 for CamK2δ.
CamK2 Kinase in Smooth Muscle Cell
Vascular smooth muscle cells synthesize CamK2γ and CamK2δ isoforms and their variants. Isoform CamK2δ is coupled to ERK1 and ERK2 signaling. Isoform CamK2δ2 specifically regulates smooth muscle cell migration.
CamK2 Kinase in Cardiomyocyte
In the heart, CamK2β is expressed at a low level. Cardiac CamK2γ and CamK2δ are implicated in calcium homoeostasis, cell cycle progression, protein secretion, cytoskeletal organization, cell apoptosis, gene expression, and excitation–contraction coupling, as they phosphorylate phospholamban, ryanodine receptor, and CaV1 channel (Vols. 3 – Chap. 3. Main Classes of Ion Channels and Pumps and 5 – Chap. 7. Heart Wall).
Calcium–calmodulin-dependent protein kinase-2δ is the major isoform synthesized in the heart that is implicated in transduction of calcium signals. Isoform CamK2δ participates in maladaptive cardiac remodeling (heart hypertrophy) under pressure overload [396]. Enzyme CamK2δ targets transcriptional repressor histone deacetylase HDAC4 that is a substrate of stress-responsive protein kinases and suppressor of stress-dependent cardiac remodeling. Phosphorylated HDAC4 dissociates from transcription factor MEF2 and translocates to the cytoplasm, where it associates with 14-3-3 protein (Fig. 5.2).


Fig. 5.2
Calcium–calmodulin-dependent protein kinase-2δ regulates gene transcription in cardiomyocyte (Source: [396]). Isoform CamK2δ phosphorylates histone deacetylase HDAC4 for nuclear export to relieve its repression on gene transcription. Nuclear HDAC4 can form dimer with HDAC5. Both HDAC4 and HDAC5 impedes activity of transcription factor myocyte enhancer factor MEF2. Phosphoinositide-dependent kinase PDK1 is another kinase that phosphorylates class-2a HDACs, i.e., both HDAC4 and HDAC5.
In rat ventriculomyocytes, endothelin-1 has a positive inotropic effect via ET A receptor,115CaV1 channel, and PKC-CamK2 pathway [397]. Endothelin-1 causes phosphoinositide hydrolysis and activation of protein kinase-C. Consequently, ET1 also increases intracellular pH by PKC-induced activation of the Na + –H + exchanger.
5.4.1.3 Calcium–Calmodulin-Dependent Protein Kinase-4
Calcium–calmodulin-dependent protein kinase-4 is a potent stimulator of Ca
-dependent gene expression. Its activation requires both Ca
–calmodulin binding and phosphorylation by Cam2K. This kinase can associate with serine/threonine protein phosphatase-2. Binding of Ca
–calmodulin and PP2 is mutually exclusive [398]. Phosphatase PP2 impedes CamK4-mediated gene transcription. Kinase CamK4 is a potent activator of Ca
-dependent transcription mediated by cAMP-responsive element-binding protein, CREB-related factor ATF1, MADS-box family members serum response factor and myocyte enhancer factor MEF2d, and nuclear receptors RORα, RORγ, and NR2f1. Whereas CamK1 and some CamK2 are ubiquitously expressed, CamK4 is detected in a limited number of cell types, such as thymocytes (CD4 + T lymphocytes), testis cells, and some cells of the central nervous system.




5.4.2 Myosin Light-Chain Kinase Family
Myosin light-chain kinases (MLCK) constitute a family of soluble protein kinases with 4 MLCK isoforms. In addition to MLCK4 (a.k.a. caMLCK-like and Sugen kinase SgK085) encoded by the MYLK4 gene, caMLCK (MLCK3), skMLCK (MLCK2), and smMLCK (MLCK1) subtypes reside in cardiomyocytes, skeletal myocytes, and smooth muscle cells, respectively; they are encoded by the MYLK3, MYLK2, and MYLK1 genes, respectively
Myosin light-chain kinases are protein Ser/Thr kinases that primarily phosphorylate 20-kDa regulatory myosin light chain (MLC2 or MyRL2), or myosin, light chain-9, regulatory (MyL9). In most cell types, MLCKs intervenes in response to Ca
influx and binding to calmodulin. Calcium–calmodulin then associates with MLCK enzyme.

The MLCK enzymes thus contribute to muscle contraction, migration, and endo- and exocytosis. In endo- and epithelia, MLCKs control barrier function. Stress fiber and focal adhesion formation rely on myosin-2 activation. Actomyosin contractility is mainly regulated by myosin light-chain kinase (MLCK) and Rho kinase (RoCK). Phosphorylation of the 20-kDa myosin light chain is counteracted by myosin light-chain phosphatase (MLCP). Enzyme MLCK directly activates myosin-2, whereas small GTPase RhoA stimulates its effector RoCK that, in turn, phosphorylates (inactivates) MLCP, hence promoting myosin light-chain phosphorylation (Fig. 5.3). Kinases MLCK and RoCK have complementary roles.


Fig. 5.3
Myosin light-chain kinase and its regulators.
5.4.2.1 MLCK Structure
MLCK contains a C2 immunoglobin domain that binds to unphosphorylated MLC, a catalytic site, and a calmodulin-binding motif. The latter operates as an auto-inhibitory domain that blocks any MLCK constitutive activity in the absence of calmodulin. This repression is relieved upon Ca
–calmodulin binding.

The MLCK structure required for its binding to MLC and activity are conserved between cell type-specific isoforms. Nonetheless, these isoforms experience different types of regulation. In smooth muscle cells, Ca
binding to calmodulin suffices to activate MLCK (necessary and sufficient condition). In other cell types, Ca
binding to calmodulin is necessary for MLCK activity, but not sufficient.


5.4.2.2 Muscular MLCK Isoforms
Muscular MLCK isoforms encompass smooth (smcMLCK or MyLK1), skeletal (skMLCK or MyLK2), and cardiac (caMLCK or MyLK3) products. In striated myocytes, Ca
-binding troponin triggers actomyosin contraction. Activation of MLCK serves to heighten the contractile strength. On the other hand, in smooth muscle cells, MLCK primes the contraction. Isotype smcMLCK has a greater structural similarity with non-muscle MLCK isoforms.

Unlike skMLCK and caMLCK, smcMLCK possesses an additional C-terminal insert and a long N-terminus with a fibronectin domain, 2 immunoglobin domains, and numerous potential phosphorylation sites [399].
5.4.2.3 Non-Muscle MLCK Isoforms
In non-muscle cells, a single gene – the MYLK1 gene – of the human genome encodes 4 high-molecular-weight MLCK isoforms (MLCK1–MLCK4). Non-muscle MLCK isoforms differ from smcMLCK mainly by their additional element of the N-terminus that results from alternative splicing. This region contains multiple sites for between-protein interactions [399].
Isoform MLCK1 was originally called endothelial cell MLCK (ecMLCK), as it was discovered in human endothelial cells. However, it is also expressed in other cell types, such as intestinal epithelial cells and neutrophils [399]. Nevertheless, MLCK1 as well as MLCK2 reach their highest levels in endothelium.
Isoform MLCK2 lacks a sequence necessary for phosphorylation and activation by Src kinase [399]. Subtypes MLCK3a and MLCK3b result from an additional distal deletion w.r.t. MLCK1 and MLCK2, respectively [399]. Isoform MLCK4 has a tissue distribution similar to other non-muscle cell MLCKs: brain, lung, kidney, liver, in addition to endothelia.
In non-muscle cells, phosphorylation of myosin-2 (Ser19) elicits stress-fiber formation. Phosphorylation of MLC enables myosin cross-bridging with actin filaments. Ca
–Calmodulin-dependent MLCK catalyzes the transfer of phosphate from ATPMg to serine residue of regulatory myosin light chain to trigger actomyosin contraction. This specialized kinase of the Ca
–calmodulin-regulated kinase superfamily exclusively phosphorylates regulatory light chain of myosin-2.


5.4.2.4 MLCK and the Endothelial Barrier
The microvascular endothelium consists of a monolayer of closely apposed endothelial cells (Vol. 5 – Chap. 9. Endothelium). It forms a semipermeable barrier between blood and tissue that controls exchange of water, electrolytes, and proteins. Transcellular transport of molecules offers a first route for the transfer of materials. The paracellular route is limited by intercellular junctions, especially tight and adherens junctions (Vol. 1 – Chap. 7. Plasma Membrane).
Tight junctions are connected to the actin cytoskeleton by zona occludens proteins ZO1 and ZO2. Adherens junctions are linked to the actin cytoskeleton via various catenin types (α, β, γ, and δ). Therefore, the tone of stress fibers influences the paracellular permeability.
5.4.2.5 MLCK Regulation
In vertebrates, the smooth and non-muscle MLCK gene locus produces 2 MLCK isoforms: MLCKL (long, high molecular mass) and MLCKS (short). The long MLCK variant is the predominant isoform in cultured cells. The short MLCK is a substrate for several kinases, such as kinases PKA, PKC, PKG, CamK2, PAK, CK2, ERK1, and ERK2 [400]. Enzymes ERK1 and ERK2 can phosphorylate (activate) MLCK and (inactivate) MLCP phosphatase.
In endothelial cells, stress fiber contraction influence vascular barrier regulation and leukocyte diapedesis. Two MLCK splice variants – ecMLCK1 and ecMLCK2 – exist. Isoform ecMLCK1 can be phosphorylated by Src kinase [401].
Myosin light-chain kinase acts as a contractile signal for tonic airway smooth muscle cells [402]. Agent MLCK is needed in phasic smooth muscle contraction. Enzyme MLCK is required for the initial contraction, whereas the sustained contraction may rely on Ca
sensitization and Ca
-independent kinases with myosin light-chain phosphatase inhibition. Nonetheless, MLCK is strongly involved in bronchoconstriction [402].


Vasodilation results from relaxation of vascular smooth muscle cells. Nitric oxide activates soluble guanylate cyclase that synthesizes cGMP messenger. The latter activates cGMP-dependent protein kinase-G that phosphorylates MLCK, but also cAMP-dependent protein kinase-A, thereby reducing the Ca
-sensitivity of myosin [403]. Protein kinase-G phosphorylates (inhibits) RhoA [404]. Upon phospholipase-C stimulation, nitric oxide synthase produces NO that targets guanylate cyclase to synthesize cGMP messenger. The latter activates PKG that can work via the cRaf–MAP2K1–ERK1/2 pathway [399]. In addition, PLC elicits Ca
ingress and PKC activation.


Intracellular Ca
concentration that depends on in- and outflux through calcium channels, exchangers, and pumps, such as voltage-gated Ca
channels, transient receptor potential Ca
channels, Na + –Ca
exchanger, and Ca
ATPase, is required for smooth muscle contraction.





5.4.3 AMP-Activated Protein Kinases
Adenosine monophosphate (AMP)-activated protein kinase (AMPK) is a heterotrimer with a catalytic α and 2 regulatory β and γ subunits (Table 5.16).
Table 5.16
Structure of AMPK. The heterotrimeric protein AMPK is consituted by a catalytic αand 2 regulatory β and γ subunits. The most common subunit isoforms synthesized in most cells are α1, β1, and γ1 chains; nevertheless, the remaining isoforms are also expressed in cardiac and skeletal muscle cells.
Subunit type | Subtypes |
---|---|
Catalytic subunit | |
α | AMPKcα1–AMPKcα2 |
(encoded by the PRKAA1 to PRKAA2 genes) | |
Regulatory subunits | |
β | AMPKrβ1–AMPKrβ2 |
(encoded by the PRKAB1 to PRKAB2 genes) | |
γ | AMPKrγ1–AMPKrγ3 |
(encoded by the PRKAG1 to PRKAG3 genes) |
This sensor of the energy state of the cell is activated by a decreasing ATP concentration and an increasing AMP concentration.116 Once activated, AMPK phosphorylates enzymes in all types of metabolism as well as transcription factors to regulate gene expression and redirect cellular metabolism from ATP-consuming anabolism to energy-generating catabolism.
Under normal conditions, the intracellular concentration of adenosine triphosphate is on the order of 1 mmol. Enzyme AMPK belongs to the main enzyme set that regulates ATP levels, discriminating between the mono- and triphosphate compounds. Nucleotides ATP and AMP competitively bind to the same AMPK site. Kinase AMPK then senses the relative ratio of ATP to AMP and controls the cell metabolism according to energy availability.
The γ subunit contains 3 binding domains for regulatory nucleotides: AMP and ATP bind to 2 sites in a mutually exclusive manner, whereas another site tethers AMP in a non-exchangeable manner [405]. Adenosine monophosphate binds to AMPK not only to stimulate it via allosteric regulation, but also to modify AMPK configuration, thereby enhancing its affinity for its kinase (AMPKK or AMP2K).
The AMPK activity is actually modulated not only by AMP-to-ATP ratio, but also by various types of AMP2K enzymes. Activation of AMPK depends on phosphorylation of the catalytic α subunit (Thr172) by STK11 (or LKb1), Ca
–calmodulin-dependent protein kinase kinase (CamKK or Cam2K), and MAP3K7 enzyme.117

Enzyme Cam2K phosphorylates AMPK in response to elevated intracellular protein Ser/Thr kinase STK11 and AMPK acts as a cellular energy sensor that regulates cell metabolism, and hence, cell fate. The STK11–AMPK pathway particularly phosphorylates acetyl coenzyme-A carboxylase (ACC), which catalyzes the rate-limiting step in lipogenesis, and tumor suppressors.
Enzyme Cam2K phosphorylates AMPK in response to elevated calcium concentration, in the absence of change in cellular adenine nucleotide level, especially Cam2Kβ that activates AMPK much more rapidly than Cam2Kα [406].
Phosphorylation (activation) of AMPK is promoted by AMP binding to the γ subunit. This signal sustains AMPK activity, as it inhibits dephosphorylation of α subunit, whereas ATP fosters dephosphorylation.118 Inactivation of AMPK occurs in response to a rising concentration of ATP.119 Phosphorylation stimulates AMPK activity several hundred-fold, whereas AMP excites AMPK severalfold, the degree of activation depending on the nature of AMPK isoforms. Like AMP, ADP binds to γ subunit and supports α-subunit phosphorylation. Unlike AMP, ADP does not directly prime AMPK activation [405].120 Kinase AMPK is also activated by leptin and adiponectin.
Activated AMPK promotes ATP-producing catabolism (fatty acid oxidation, glucose uptake, and glycolysis; Vol. 1 – Chap. 4. Cell Structure and Function) and impedes ATP-consuming metabolism (synthesis of fatty acid, cholesterol, glycogen, and proteins).
Ubiquitous AMPK, a sensor of cellular energy, increases sensitivity of cells to insulin [407]. In the liver, AMPK phosphorylates (inhibits): (1) acetylCoA carboxylase, which converts acetylCoA to malonylCoA for fatty acid synthesis in the liver; (2) 3-hydroxy 3-methylglutarylCoA reductase, thus decreasing cholesterol synthesis in the liver; and (3) CREB-regulated transcription coactivator-2 (TORC2), which activates gluconeogenesis and upregulates expression of insulin receptor substrate-2, a mediator of insulin signaling. In contrast, in muscles, activated AMPK promotes fatty acid oxidation and activates PGC1α promoter, thus increasing metabolism.
Enzyme AMPK regulates tight junction assembly and disassembly [408]. It is phosphorylated by STK11 that controls cell polarity. Kinase STK11, indeed, activates Par1 implicated in cell polarity and microtubule affinity-regulating kinase (MARK). It phosphorylates AMPK during tight junction assembly stimulated by calcium. Moreover, AMPK enhances the stability of tight junction from disassembly induced by calcium depletion. Enzyme AMPK also phosphorylates the regulatory site of non-muscle myosin regulatory light chain involved in mitosis and cell polarity [409]. Regulation by STK11 of epithelial cell polarity and mitosis is due to MRLC phosphorylation by AMPK.
In central neurons,KV2.1 channel regulates action potential frequency. Firing of action potentials in excitable cells accelerates ATP turnover. Enzyme AMPK activated by ATP depletion phosphorylates KV2.1 channel, thereby causing hyperpolarizing shifts in the current–voltage relationship and, then, modifying channel activation and inactivation [410]. As AMPK facilitates activation of KV2.1 channel, it reduces neuronal excitability and conserves energy. On the other hand, AMPK phosphorylates (inactivates) KCa1.1 and KCa3.1 as well as KIR6.2 channels.
5.4.4 AMPK-Related Kinases
Twelve AMPK-related kinases — brain-specific kinases (BrSK1–BrSK2), microtubule affinity-regulating kinases (MARK1–MARK4), maternal embryonic leucine-zipper kinase (MELK), nuclear AMPK-related kinases (NuAK1–NuAK2), and members of the salt-induced kinase family (SIK1–SIK3; Sect. 3.1.3) constitute with AMP-activated protein kinase the AMPK family. These 12 enzymes are closely related to the AMPKcα1 and AMPKcα2 catalytic subunits.
Some members are involved in the regulation of cell polarity; others control cell differentiation. Brain-specific kinases are neuron-polarity regulators. Microtubule affinity-regulating kinases regulate microtubule dynamics, as they phosphorylate microtubule-associated proteins; they are also involved in the establishment of cell polarity. Maternal embryonic Leu-zipper kinase contributes to stem cell renewal, cell cycle progression, and pre-mRNA splicing. Enzyme NuAK1 impedes apoptosis and controls cellular senescence. Kinase NuAK2 is activated in response to glucose deprivation, ATP depletion, and hyperosmotic stress. Salt-induced kinases participate in steroido- and adipogenesis, particularly SIK2121 that intervenes during early stage of adipogenesis.
Kinase STK11 (LKb1) phosphorylates (activates) AMPK family members [411]. In addition, STK11 phosphorylates a single member of the family of SNRK kinases (SNF1 [plant AMPK]-related protein Ser/Thr kinase) that are distant relatives of AMPK. Kinase STK11 forms a complex with regulator pseudokinase StRAd and Mo25 to phosphorylate AMPK (Sect. 3.4.6).
Except MELK kinase, all AMPKRKs are phosphorylated by STK11, but not other AMP2Ks, at a site equivalent to Thr172 in AMPK.122 Kinase Cam2Kα phosphorylates BrSK1 kinase (Thr189). Other AMPKR2Ks (or AMPKRKKs) exist such as MAP3K16, a MAR2K for microtubule-associated protein (MARK) [411]. Glycogen synthase kinase-3β phosphorylates (inactivates) MARK2. Protein kinase-A targets salt-inducible kinase SIK1 (Ser577) to cause translocation from the nucleus, relieve cAMP-response element-binding protein (CREB) inhibition, and lower phosphorylation of CREB-regulated transcription coactivator (CRTC or transducer of regulated CREB). Kinase SIK1 can also be phosphorylated (activated) by Ca
–calmodulin-dependent kinase (Thr322) in response to an increase in intracellular sodium concentration. Protein kinase-B2 phosphorylates SIK2 (Ser358) to disrupt and degrade target of rapamycin TORC2 complex.

The AMPK-related kinases undergo ubiquitination.123 Their ubiquitin-associated domain interact swith the catalytic sequence to render kinase conformation suitable for STK11-induced phosphorylation [411]. On the other hand, ubiquitin-associated domains of MARK1 and MARK2 bind to and inhibit the catalytic motif. Conversely, MARK4 and NuAK2 interact with the deubiquitinase X-linked ubiquitin-specific USP9X peptidase.
Phosphorylated AMPK-related kinases can be sequestered in the cytosol by ubiquitous 14-3-3 proteins. For example, MARK phosphorylation by atypical PKC favors 14-3-3 binding and causes relocalization from the plasma membrane to the cytoplasm [411]. Once phosphorylated by STK11, SIK1 and SIK3 interact with 14-3-3 proteins.
5.4.4.1 Brain-Specific Kinases
Two brain-specific kinase isoforms exist: BrSK1124 and BrSK2.125 They are involved in neuronal polarity and synapse development. Isoform BrSK1 localizes to and associates with synaptic vesicles [411]. In addition, BrSKs participate in neuron polarity to produce axons and dendrites. Both BrSK1 and BrSK2 phosphorylate microtubule-associated protein Tau that regulates microtubule stability. Furthermore, BrSK1 acts as a checkpoint kinase, as it phosphorylates Wee1 (Ser642) and CDC25c (Ser216). It can be phosphorylated by ATMK and ATRK that are implicated in the DNA-damage response.
5.4.4.2 Maternal Embryonic Leucine Zipper Kinase
Maternal embryonic leucine zipper kinase (MELK) is maximally active during mitosis. It is counteracted by CDC25b phosphatase. It interacts with the splicing factor nuclear inhibitor of PP1 (NIPP1) to block pre-mRNA splicing during mitosis [412]. It is strongly expressed during early embryogenesis. It contributes to the self-renewal of multipotent neural progenitors. Two splice variants (MELK1 and MELK2) have been identified.
Kinase MELK possesses many autophosphorylation sites, but its activity is not affected by phosphorylation by the STK11–STRAD–CaB39 complex that is required to activate all other AMPK-related protein kinases. It interacts with ZPR9 transcription factor [412].
5.4.4.3 Microtubule Affinity-Regulating Kinase Subfamily
Four microtubule affinity-regulating kinases constitute the MARK subfamily of the family of AMPK-related protein kinases: MARK1, MARK2 (or EMK), MARK3 (or cTAK), and MARK4 (or MARKL1). These 4 members are encoded by 4 Mark genes.
The Mark paralogs differ in tissue distribution, subcellular location, and expression regulation [413]. Expression of MARK1 is high in the brain, heart, kidney, skeletal muscle, spleen, and pancreas, and low in the lung and liver [413].
Two isoforms of MARK4 exist: MARK4S and MARK4L that are produced in neural progenitor cells and neurons, respectively [411].
Microtubule affinity-regulating kinases contribute to the regulation of cell polarity and microtubule stability as well as possibly cell cycle control and Wnt signaling. They can dissociate microtubule-associated proteins from microtubules, hence destabilizing the microtubule network, and affecting intracellular transport [411]. Overexpression of MARK kinase in cultured cells actually causes removal of Tau and related microtubule-associated proteins from the microtubule surface, hence transforming microtubules into bundles and reducing the microtubule network.
Other MARK activities can be specific to MARK isoforms. Isoform MARK1 can be involved in the regulation of synaptic remodeling, MARK2 in the regulation of metabolism, and MARK3 in cell cycle control.
Enzyme MARK phosphorylates doublecortin, thereby regulating doublecortin binding to microtubule. On the other hand, MARK2 that impedes axon formation and favors dendrite development is inhibited by P21-activated kinase PAK5. All human MARKs are phosphorylated (activated) by Ser/Thr kinase STK11 (or LKb1) [413].
MARK1
MARK2
In addition to STK11, MARK2 kinase can also be activated by MAP3K16 and GSK3β kinases. Conversely, MARK2 is inhibited by its association with PAK5 kinase (without phosphorylation). Kinase MARK2 interacts with PKB, MAP3K3, and 14-3-3γ, 14-3-3σ, and 14-3-3θ sequestrators [251]. Kinases PKCλ and PKCζ can phosphorylate (inactivate) MARK2 and MARK3, as they provoke binding to 14-3-3 proteins.
MARK3
Kinase MARK3 phosphorylates CDC25 phosphatase, hence preventing CDC25 activation of the CcnB–CDK1 complex. It also phosphorylates (inactivates) PIM1 kinase to promote cell cycle progression at the G2–M transition [411].
Kinase MARK3 interacts with Ras guanine nucleotide-releasing factor RasGRF1 vacuolar protein-sorting protein VSP11, mitochondrial processing peptidase β subunit, neuropeptide secretoneurin (or chromogranin-B), lysosomal H + ATPase (ATP6V0C), serum amyloid-A-like-1, patatin-like phospholipase domain-containing protein PnPLa2, transcription elongation factor TcEA2, carrier of thyroxine (T4) and retinol transthyretin, ubiquitin-C, and 14-3-3β, 14-3-3γ, 14-3-3σ, and 14-3-3θ [251].
MARK4
Kinase MARK4 interacts with microtubule-associated protein-2 and -4, and MAPT (Tau), tubulin-α1a, -β, and -γ1, myosin heavy chains-9 and -10, actin-α1, actin-dependent regulator of chromatin SMARCA4, RhoGEF2, CDC42, PP2 regulatory PP2r1α and catalytic PP2cβ subunits, hepatocyte growth factor-regulated tyrosine kinase substrate, heat shock 70-kDa protein-4, ubiquitin-C, ubiquitin ligase RNF41, ubiquitin-specific peptidase-7 and -9, and 14-3-3η [251, 413]. Two MARK4 splice isoforms exist: long (MARK4 L ) and short (MARK4 S ) form that has a truncated C-terminus. In the brain, MARK4 S is predominant.
5.4.4.4 NuAK Kinases
Two isoforms of NuAK kinases exist: NuAK1127 and NuAK2.128 Both NuAK1 and NuAK2 are activated by STK11 (LKb1) kinase.
NuAK1
Kinase NuAK1 interacts with several myosin phosphatases, such as the dimer made of protein phosphatase-1 regulatory subunit-12A (PP1r12a), or myosin phosphatase target subunit-1 (MyPT1), and catalytic PP1cβ subunit. Phosphorylation of PP1r12a (Ser445, Ser472, and Ser910) by NuAK1 promotes the interaction of PP1r12a with 14-3-3 adaptors, thereby suppressing phosphatase activity [414]. Cell detachment is characterized by phosphorylation of PP1r12a by NuAK1 to enhance phosphorylation of myosin light chain-2.
NuAK2
Anti-apoptotic NuAK2 is regulated by glucose or glutamine deprivation, elevated intracellular AMP concentration, as well as endoplasmic reticulum, hyperosmotic, salt, and oxidative stress [411]. Isoform NuAK2 phosphorylates regulatory PP1r12a subunit.
5.4.4.5 Salt-Inducible Kinases
Salt-inducible kinase SIK1 contributes to the regulation of steroidogeneis following ACTH stimulation. STK11-activated SIK1 phosphorylates transducer of regulated CREB, thereby causing its nuclear export and inhibiting CREB activity [411]. Kinase SIK1 is also activated by elevated intracellular sodium concentration and stimulates Na + –K + ATPase to maintain cell volume and composition [411].
Salt-inducible kinase SIK2 (a.k.a. Qin-induced kinase [QIK]) is ubiquitous with its highest levels in adipose tissue. Kinase SIK2 phosphorylates insulin receptor substrate-1 [411]. Kinase SIK2 also inhibits CREB-mediated gene expression, as it phosphorylates target of rapamycin complex TORC2, a coactivator of CREB, that then connects to 14-3-3 for cytoplasmic sequestration.
Salt-inducible kinase SIK3 (a.k.a. QSK) can intervene in cell proliferation, as its suppression causes spindle and chromosome abnormalities [411].
5.4.4.6 Other Types of AMPK-Related Kinases
Eight other kinases — hormonally upregulated Neu-associated kinase (HUNK), non-inducible immunity gene product NIM1, sucrose non-fermenting protein (SNF1 [plant AMPK])-related kinase (SNRK), testis-specific Ser/Thr kinases (TSSK1–TSSK4 and TSSK6) — belong to the AMPK branch of the human kinome tree. Kinase SNRK is highly expressed in the testis. Kinase STK11 preferentially phosphorylates Thr residues with a Leu residue at the − 2 position, hence not kinases of the HUNK and TSSK subfamilies that do not possess this Leu residue.
5.4.5 Checkpoint Kinase Family
Protein Ser/Thr kinases checkpoint kinases ChK1 and ChK2 are encoded by the CHEK1 and CHEK2 genes, respectively. These protein kinases are activated in response to DNA damage and provoke cell cycle arrest.
5.4.5.1 ChK1 Kinase
Kinase ChK1 phosphorylates phosphatases CDC25 that operate during the cell cycle, particularly for entry into mitosis. Phosphatase CDC25 (Sect. 8.3.15), when it is phosphorylated (Ser216) by ChK1 binds to an adaptor in the cytoplasm for sequestration. Hence, it cannot relieve the inhibition of the mitotic CcnB–CDK1 complex caused by Wee1 kinase. Consequently, cell is prevented from entering into mitosis.
5.4.5.2 ChK2 Kinase
Kinase ChK2 operates in DNA-damage response and mitotic progression, during which it can play a role distinct from that in DNA damage, independently of P53 factor. Kinase ChK2 is rapidly phosphorylated by ATMK in response to DNA damage. Once activated, ChK2 inhibits CDC25c phosphatase, thereby preventing entry into mitosis. It also stabilizes P53, leading to cell cycle arrest in G1 phase. In addition, ChK2 interacts with and phosphorylates another tumor suppressor with ubiquitin ligase activity, the breast cancer-related protein BrCa1 for accurate spindle formation and stable attachment of microtubules to kinetochores that aims at maintaining genome integrity [415]. It eventually allows BrCa1 to restore cell survival after DNA damage.
5.4.6 Death-Associated Protein Kinase Family
Death-associated protein kinase DAPK1 is a Ca
–calmodulin-regulated Ser/Thr protein kinase that participates in apoptosis launched by factors, such as interferon-γ, tumor-necrosis factor-α, transforming growth factor-β, TNFSF6, and lipid ceramide [416]. It suppresses integrin signaling, particularly during ischemic brain injury. Autophosphorylation (Ser308) inhibits Ca
–calmodulin interaction, thus attenuating its basal activity. Activity of DAPK1 rises upon phosphorylation by extracellular signal-related kinase (Ser735).


Kinase DAPK1 is expressed in the nervous system, particularly in hippocampus. It has been detected in mouse lung, bladder, liver, kidney, heart, and uterus [416]. In the mouse, DAPK has 2 α and β isoforms. Isoform DAPKβ has an 11-amino acid extension at its C terminus. The latter has a slight pro-apoptotic activity.
Substrates of DAPK1 include myosin light chain, DAPK3 kinase that regulates stress fiber–focal adhesion assembly, syntaxin-1A, and calmodulin-dependent kinase kinase (Cam2K) [416]. Kinase DAPK1 interacts with ubiquitin ligase DAPK-interacting protein DIP1,129 Fas (TNFRSF6a)-associating death domain-containing protein FADD, ankyrin repeat-containing protein Krit1, TNFR-binding protein TNFSF1a, and Tau protein [251].
Members of the CAMK superfamily tether to calmodulin. Ability of DAPK to bind calmodulin correlates with its catalytic activity [417].
5.4.7 Death Receptor-Associated Protein-Related Apoptotic Kinase Family
Two protein Ser/Thr kinases, death receptor-associated protein-related apoptotic kinases DRAK1 and DRAK2, have a catalytic domain related to that of death-associated protein (DAP) kinase (DAPK) involved in apoptosis initiated by interferon-γ. Like death-associated protein kinase DAPK3,130 the kinase domain of both DRAK subtypes are homologous to that of DAPK enzyme [418].131 Both DRAK isoforms triggers cell apoptosis. However, the sensitivity to DRAK activity may depend on the cell type. Both DRAK subtypes localize to the nucleus and cytoplasm, unlike DAPK, which is tightly associated with the cytoskeleton, and DAPK3, which resides in the nucleus [418].
5.4.7.1 DRAK1
DAPK-related apoptosis-inducing protein kinase DRAK1 is also called protein Ser/Thr kinase STK17a. This calmodulin-dependent kinase interacts with calcineurin homologous protein (CHP),132 an inhibitor of DRAK2 and PP3. It is highly expressed in the lung, pancreas, and placenta, as well as, at lower levels, in the brain, heart, liver, kidney, and skeletal muscle.
5.4.7.2 DRAK2
Enzyme DRAK2, or STK17b, resides in the cytoplasm and nucleus, particularly in lymphocytes. It is expressed in many cells, with its highest expression in lymphoid organs. It is synthesized at high levels in adipose tissue and low levels in lungs and small intestine [419].
Kinase DRAK2 undergoes autophosphorylation (Ser10 and Ser12) upon antigen receptor ligation in B lymphocytes and thymocytes. It phosphorylates myosin light chain in vitro. It inhibits calcineurin homologous protein-B (CHP). In particular, DRAK2 is also able to cause apoptosis of cardiomyocytes [419].
5.4.8 PIM Kinase Family
Proto-oncogene PIM1 has been identified as an activated gene in T-cell lymphoma induced by provirus insertion of Moloney murine leukemia virus. Its product is a protein Ser/Thr kinase that is highly expressed in hematopoietic cells as well as testis. The PIM family contains 3 known members (PIM1–PIM3). Kinase PIM1 and other family members are also produced in cells other than blood cells in a cell cycle-dependent manner. Related isoforms, PIM2 and PIM3, have overlapping as well as distinct functions in cell growth and survival.
5.4.8.1 PIM1
Many PIM1 target molecules regulate cell cycle progression and apoptosis. Furthermore, in heart, PIM1 has positive inotropic effects and impedes maladaptive hypertrophy. Moreover, mitochondrial PIM1 preserves mitochondrial integrity. Kinase PIM1 enhances mitochondrial resistance to Ca
-induced opening of permeability transition pores and inhibits cytochrome-C release primed by pro-apoptotic set of the BCL2 family (BAX and BAK) that elicits mitochondrial outer membrane permeabilization in response to apoptotic stimuli.

Kinase PIM1 interacts with chromobox homologs CBx1 and CBx3 (a.k.a. heterochromatin proteins HP1β and HP1γ), cyclin-dependent kinase inhibitor CKI1a, nuclear mitotic apparatus protein NuMA1, nuclear factor of activated T cells NFAT1, CDC25a phosphatase, sorting nexin SNx6, and heat shock protein HSP90a1 [251].
5.4.8.2 PIM2
Kinase PIM2 interacts with BCL2-antagonist of cell death (BAD), copper transporter CuTc, heterogeneous nuclear ribonucleoprotein HNRPh3, and NADH dehydrogenase (ubiquinone)-1β subcomplex-8 (NDUFb8) [251].
5.4.9 Protein kinase-D Family
Protein kinases of the PKD family (PKD1–PKD3) belongs, in fact, to the CAMK superfamily. Its members are activated by diacylglycerol. Protein kinases-D are activated via a PKC-dependent pathway by: (1) various regulator petides (bombesin, bradykinin, endothelin, and vasopressin), lysophosphatidic acid, and thrombin that act via Gq, G12, Gi (Vol. 3 – Chap. 7. G-Protein-Coupled Receptors) and Rho GTPases (Sect. 9.3.12); (2) growth factors (e.g., platelet-derived- and insulin-like growth factor); (3) crosslinking of B- and T-cell receptors on B and T lymphocytes, respectively, and (4) oxidative stress [420]. PKC-dependent PKD activation has been detected in many normal cell types, including fibroblasts, smooth muscle cells, cardiomyocytes, neurons, lymphocytes, mastocytes, and platelets, among others. Kinase PKCε particularly activates PKD.
5.4.9.1 Protein kinase-D1
Protein kinase-D1 (or protein kinase-Cμ) participates in the regulation of many processes, such as signal transduction, membrane trafficking, and cell survival, differentiation, proliferation, and migration. They targets JNK and ERK kinases. Both PKD1 and PKD2 undergo a reversible translocation from the cytosol to the plasma membrane in response to GPCR stimulation (Gq and G12/13 pathways), which requires PKC activity.
Upon activation of small RhoA GTPase, protein kinase-D1 regulates cofilin-mediated Factin reorganization and cell motility via slingshot phosphatase [422]. Actin remodeling at the leading edge of migrating cells that generates cell protrusions, defines migration direction, and initiates lamellipodium growth, is indeed controlled by a temporospatial coordination of Rho GTPases, kinases, and phosphatases.
Protein kinase-D1 transduces stress stimuli involved in maladaptive cardiac remodeling generated by pressure overload, angiotensin-2, and adrenergic signaling [421]. Protein kinase-D is a stress-responsive kinase that phosphorylates class-2 histone deacetylases, thereby dissociating their association with transcription factor myocyte enhancer factor MEF2, which, upon its liberation, stimulates prohypertrophic transcription. Class-2 histone deacetylases that silence MEF2 target genes are also targeted by Ca
–calmodulin-dependent kinases to relieve MEF2 repression. Isoforms PKD2 and PKD3 cannot fully compensate for PKD1 loss. PKD1 and PKA phosphorylate cardiac troponin-I on the same sites and hence reduce myofilament Ca
sensitivity.


5.4.9.2 Protein Kinase-D2
Protein kinase-D2 functions in diacylglycerol-mediated signaling cascades initiated by the activated G-protein-coupled receptors. It targets phosphatidylinositol-4 kinase-3 and regulates nuclear factor-κB. It also controls the formation of exocytic carriers from the trans-Golgi network and vesicular transport by regulation of PI(4)K3β. Activity of PKD2 is regulated by PLCγ and novel PKC isoforms (PKCδ, PKCε, PKCη, and PKCθ).
5.4.9.3 Protein Kinase-D3
Protein kinase-D3, or PKCν, intervenes in diacylglycerol-mediated signaling cascades initiated by activated B-cell receptors and G-protein-coupled receptors. Isozyme PKD3 resides in the nucleus and cytoplasm of unstimulated cells. PKD3 Activity is regulated by PLCγ and novel PKC isoforms (PKCδ, PKCε, PKCη, and PKCθ), as well as Rac GTPase and G12 and G13 subunits of G proteins [424]. Ubiquitous PKD3 operates in the formation of exocytic carriers from the trans-Golgi network and glucose transport.
5.5 PIKK Superfamily
Members of the phosphatidylinositol 3-kinase-related kinase (PIKK) superfamily include ataxia telangiectasia mutated (ATMK), ATM and Rad3-related (ATRK), DNA-dependent protein kinase (DNAPK), suppressor with morphological effect on genitalia SMG1,133 and target of rapamycin, as well as transformation/transcription domain-associated protein (TRRAP).134
5.5.1 Ataxia Telangiectasia Mutated and ATM and Rad3-Related Kinases
Ataxia telangiectasia mutated (ATMK) and ataxia telangiectasia and Rad3-related (ATRK) kinases are recruited and activated by DNA damage.135 These kinases serves as a cellular damage sensor that coordinates the cell cycle with damage-response checkpoints and DNA repair to preserve genomic integrity. These cell cycle checkpoint kinase indeed phosphorylate several proteins that activate of the DNA-damage checkpoint to elicit cell cycle arrest and DNA repair or apoptosis. Genotoxic stresses repress the target of rapamycin complex TORC1 upon P53 activation and via P53-regulated sestrin-1 and -2.136 Sestrin-1 and -2 activate AMPK and repress TORC1 signaling (negative feedback loop) [425]. Sestrin concentration rises when reactive oxygen species accumulate and cause activation of Jun N-terminal kinase and transcription factor Forkhead box O. Sestrins prevent excessive TOR activation.
Ataxia-telangiectasia mutated protein kinase is a component of DNA-damage processing (Vol. 1 – Chaps. 4. Cell Structure and Function and 5. Protein Synthesis). Enzyme ATMK becomes activated within a complex made of meiotic recombination-11 homolog (MRE11), radiation sensitivity DNA-repair Rad50 homolog (Rad50), and Nijmegen breakage syndrome-1 homolog or nibrin (NBS1), i.e., the MRN (MRE11–Rad50–NBS1) DNA-repair complex at sites of double-stranded DNA breaks to coordinate the diverse components of the DNA-damage response.
Kinase ATMK phosphorylates transcriptional regulators P53, BrCa1, and BrCa2, DNA repair protein RAD51, checkpoint kinases ChK1 and ChK2, checkpoint proteins RAD9 and RAD17, DNA-repair nibrin (Nbs1), double-strand break repair protein MRE11a, telomeric repeat-binding factor TeRF1, segregation of mitotic chromosome-1-like protein SMC1l1, minichromosome maintenance complex component MCM2, DNA-mismatch repair protein Mlh1, mutS homologs MSH2 and MSH6 (or G/T mismatch-binding protein GTBP), replication factor-C (RFC1), DNA polymerase-θ, ligase-4, telomerase RNA component, retinoblastoma-binding protein RbBP8, histone H2A, adaptors AP1β1, AP2α2, and AP3β2, Abl1 kinase, small RHEB GTPase, eukaryotic translation initiation factor 4E-binding protein eIF4eBP1, and cAMP responsive element binding protein CREB1 [251].
Like ATMK, ATRK targets transcriptional regulators P53, DNA-damage repair proteins BrCa1 and BrCa2, double-strand break repair protein MRE11a, mutS (mismatch repair protein) homologs MSH2, checkpoint protein RAD17, checkpoint kinase ChK1, adaptors AP1β1 and AP2α2, Abl1 kinase, and small GTPase RHEB [251]. Kinase ATRK also interacts with ATR-interacting protein (ATRIP) to recognize single-stranded DNA coated with replication protein RPA, replication protein RPA1, histone deacetylases HDac1 and HDac2, chromodomain helicase DNA-binding protein CHD4, metastasis-associated proteins Mta1 and Mta2, PTP synthase, E4F transcription factor E4F1, claspin, DNA-directed polymeraseδ1, HER3-binding protein EBP1 or proliferation-associated protein PA2G4, PI3K, protein phosphatase PP2, and RhoGEF1 [251].
Ataxia telangiectasia mutated kinase also participates in the cellular damage response to reactive oxygen species owing to a P53-independent pathway [426].137 Kinase ATMK activates the tumor suppressor tuberous sclerosis protein TSC2 via the STK11 (LKb1)–AMPK metabolic pathway in the cytoplasm to repress target of rapamycin complex TORC1 and induce autophagy.138 Moreover, ATMK is also directly activated (oxidized and dimerized) by reactive oxygen species [427]. It thus serves as a sensor of an increased production of reactive oxygen species in cells.
5.5.2 DNA-Dependent Protein Kinase
Nuclear DNA-dependent Ser/Thr protein kinase (DNAPK) is a proteic complex. The catalytic subunit of DNA-dependent protein kinase (DNAPKc)139 is inactive, but is able to undergo autophosphorylation. This subunit forms DNAPK with Ku70–Ku80 heterodimer140 to trigger its kinase activity. Enzyme DNAPK intervenes positively or negatively in the regulation of DNA end access to repair via the homologous recombination pathway as well as in non-homologous end joining pathway of DNA repair to rejoin double-strand breaks [428]. It is also required for V(D)J recombination that uses the same NHEJ pathway to promote immune system diversity. Enzyme DNAPK also cooperates with ATRK and ATMK to phosphorylate proteins involved in the DNA-damage checkpoint. Checkpoint kinase ChK1 that regulates mitotic progression in response to DNA damage by blocking the activation of CcnB–CDK1 stimulates the kinase activity of DNAPK to phosphorylate P53 (Ser15 and Ser37) [429]
5.5.3 Target of Rapamycin
Protein Ser/Thr kinase target of rapamycin (TOR)141 is a controller of cell growth, proliferation, survival, and metabolism (Vol. 2 – Chap. 2. Cell Growth and Proliferation). It reacts to 4 major inputs: (1) nutrients such as amino acids; (2) hormones and growth factors such as insulin; (3) cellular energy level, i.e., AMP/ATP ratio; and (4) stress such as hypoxia. It controls several anabolic and catabolic processes, as it regulates protein translation, ribosome genesis, nutrient transport, autophagy, and activation of members of the AGC superfamily,142 such as S6K, PKB, PKCα, and SGK kinases.143
The primary cilium contributes to the regulation of cell size. Cilia that act as signaling platforms control TOR signaling. In ciliated cells, bending by flow of the primary cilium144 downregulates TOR enzyme ( mechanotransduction) [432]. Cilia are actually mechanosensors that can cause calcium fluxes. The basal body of cilia serves as a restricted subcellular compartment for activation of LKb1 kinase (located at the axoneme and basal body) upon primary cilium bending that phosphorylates at the basal body the energy sensor and kinase AMPK, thereby inhibiting the TORC1 complex.145
5.5.3.1 TOR Complexes
Target of rapamycin kinase acts in protein complexes in a nutrient-sensing system. It forms 2 functional TOR complexes (Table 5.17): (1) mitogen-, nutrient-, and rapamycin-sensitive complex TORC1 and (2) mitogen-sensitive and nutrient- and rapamycin-insensitive complex TORC2. Protein Ser/Thr kinase target of rapamycin thus serves as the catalytic subunit of TORC1 and TORC2 complexes.
Table 5.17
Activity of target of rapamycin complexes (mitogen- and nutrient-sensitive complex TORC1 and mitogen-sensitive and nutrient-insensitive complex TORC2).
Complex | Effects |
---|---|
TORC1 | DNA replication, RNA processing, protein synthesis |
cell growth (S6K1–S6K2 substrates), | |
cell division (4eBP1–4eBP3 substrates), | |
cell autophagy, | |
intracellular transport, | |
cell metabolism, lipogenesis, | |
inhibitory feedback of growth factor signaling | |
TORC2 | Cell survival, |
actin polymerization, chemotaxis | |
(PKC–AC9–cAMP–RhoA–RoCK–MLC axis) |
Kinase TOR also complexes with BCLxL protein of the mitochondrial outer membrane, a regulator of apoptosis and mitochondrial permeability, and voltage-dependent anion-selective channel VDAC1 [433]. In fact, it phosphorylates BCLxL agent. The mitochondrial TOR complex may regulates the balance between glycolytic and respiratory metabolism. Kinase TOR reduces aerobic glycolysis.
The TORC1 and TORC2 complexes possess numerous substrates. Among the latter, adaptor growth factor receptor-bound protein GRB10 operates in the formation of signaling complexes linked to growth factor receptors. In particular, the cytoplasmic protein GRB10146 suppresses signaling by insulin and insulin-like growth factors, thereby adding a complementary negative feedback loop of the PI3K and ERK pathways, in addition to the S6K1–IRS control [430, 434] (Table 5.18).
Table 5.18
Negative feedback loops triggered by the insulin and insulin-like growth factor pathways.
IR/IGFR–IRS–TORC1–GRB10 | Inhibition of RTKs (Tier 0) |
---|---|
IR/IGFR–IRS–TORC1–S6K1 | Inhibition of IRS (Tier 1) |
Small guanosine triphosphatase Rac1 (much more than Rac2) connects to TOR as well as with Raptor and Rictor, independently of its activity status (i.e., Rac1GTP or Rac1GDP) [435]. Agent Rac1 regulates both TORC1 and TORC2 in response to growth factor stimulation. Once bound to TOR, Rac1 recruits TORC1 and TORC2 at specific membrane sites.
TORC1 Complex
The TORC1 complex is composed of TOR, regulatory associated protein of TOR (Raptor), and Lethal with Sec13 (thirteen) LST8147 In addition, TORC1 associates with 40-kDa, 14-3-3-binding, proline-rich Akt (PKB) substrate PRAS40,148 apoptosis inhibitor FK506-binding protein FKBP38, and Rag GTPases [436]. Furthermore, TORC1 promotes the synthesis of ribosomes and transfer RNAs.
TORC1 Substrates in Cell Growth and Proliferation
Among many targets, TORC1 phosphorylates eukaryotic translation initiation factor eIF4e-binding proteins (4eBP1–4eBP3), thereby controlling protein synthesis. The 4eBP proteins are necessary for TORC1 activation of cell proliferation, but are dispensable for TORC1 effect on cell growth [437]. The TORC1 complex promotes cap-dependent translation initiation through phosphorylation and inhibition of 4eBP proteins.149
On the other hand, cell growth requires another TORC1 substrate, the ribosomal S6K1 and S6K2 kinases. Whereas TORC2 phosphorylates protein kinase-B to promote cell proliferation and survival, TORC1 targets S6K and 4eBP1 proteins to activate translation initiation and elongation. The TORC1 complex indeed phosphorylates S6K kinases.150
TORC1 in Cell Metabolism
The liver is an important site for glucose and lipid metabolism. In hepatocytes, insulin activates insulin receptor that recruits insulin receptor substrate and activates phosphoinositide 3-kinase and protein kinase-B, hence lipo- and gluconeogenesis [438].
The PI3K–PKB axis promotes glucose uptake via the translocation of glucose transporter GluT4 to the plasma membrane as well as Forkhead box FoxO1 phosphorylation (inactivation), thereby impeding gluconeogenesis.
Insulin stimulates lipogenesis via the transcription factor sterol and regulatory element-binding protein SREBP1c via the TORC1 complex. Factor SREBP1c controls the transcription of genes for cholesterol, fatty acid, triglyceride, and phospholipid synthesis. Insulin promotes SREBP1c activity, as it: (1) increases the transcription of the Srebp1c gene and (2) favors SREBP1c cleavage from its membrane-bound precursor.
TORC2 Complex
The TORC2 complex consists of TOR, rapamycin-insensitive companion of TOR (Rictor), stress-activated protein kinase (SAPK)-interacting protein MAPKAP1 (or SIN1),152 LST8, and the proteins observed with Rictor Protor-1 and -2.
TORC2 in Chemotaxis
Adenylate cyclase AC9 impedes the activity of RhoA and, hence, RoCK that phosphorylates myosin light chain and increases the activity of myosin-2, thereby reducing the persistence of the uropod.153 Both Rictor and PKC (but not PKB) are required for cAMP generation at the rear of the cell and uropod retraction. Myosin-2 is regulated by TORC2 via the PKC–AC9–cAMP–RhoA–RoCK axis, independently of actin reorganization during neutrophil chemotaxis [440]. Subunit Gi controls cell polarity via TORC2, as it regulates actin polymerization at the front and impedes myosin-2 assembly at the rear.
5.5.3.2 Other Effects of TOR Kinase
5.5.3.3 Molecular Tranfer
Kinase TOR precludes turnover of amino acid, glucose, iron, and lipoprotein transporters and promotes exocytosis of transporters to the plasma membrane. The TORC1 complex is activated by amino acids via RHEB and Rag GTPases, the latter ditermining the localization of TORC1 to RHEB-containing compartments.
Cholesterol is a constituent of the plasma membrane that confers impermeability to cellular membranes and can participate in signal transduction via membrane rafts. Two sources of cholesterol exist: intra- and extracellular. Whatever its origin, cholesterol needs proper membrane insertion. Intracellular transfer of cholesterol, particularly cholesterol egress from endosomes to the plasma membrane, requires TOR in endothelial cells [441].
Cell Autophagy
Kinase TOR inhibits autophagy. It senses cellular energy level via the STK11–AMPK–TSC1/2 pathway. It is inhibited by cellular stresses such as hypoxia via HIF1 that stimulates Regulated in development and DNA-damage response gene products REDD1 and REDD2 that activate TSC1 and TSC2 proteins.
5.6 CK1 Superfamily
Casein kinase activity can be detected in most cell types and is associated with multiple enzymes. Two families of casein kinases exist: family-1 and -2 casein kinases. Casein kinases of the CK1 and CK2 families contribute to signaling specificity in the β-catenin-independent Wnt axis (Vol. 3 – Chap. 10. Morphogen Receptors).
5.6.1 Casein Kinase-1 Family
Casein kinases of the CK1 family regulate signal transduction in most cell types, in association with multiple enzymes. The CK1 family includes at least 7 isoforms (CK1α–CK1ε) and their splice variants. The CK1γ subfamily comprises 7 isozymes (CK1α1–CK1α2, CK1γ1–CK1γ3, CK1δ, and CK1ε) that are encoded by 7 genes (CSNK1A1, CSNK1A1L, CSNK1G1–CSNK1G3, CSNK1D, and CSNK1E).
Distinct CK1 family members have various roles. Mammalian CK1 isoforms are involved in membrane trafficking, circadian rhythm, cell cycle progression, chromosome segregation, cell apoptosis, and cellular differentiation. Mutations and deregulation of CK1 expression and activity lead to multiple diseases (neurodegenerative disorders such as Alzheimer’s and Parkinson’s disease, sleeping disorders, and proliferative diseases such as cancer).
Substrates of CK1 include cytosolic proteins (glycogen synthase, acetylCoA carboxylase, and inhibitor-2 protein of protein phosphatase-1), cytoskeletal proteins (myosin, troponin, and ankyrin), membrane-associated proteins (spectrin, neural cell adhesion molecule, and insulin receptor), nuclear proteins (P53, cAMP response element modulator, and RNA polymerase-1 and -2), and proteins involved in protein synthesis (initiation factor-3, -4B, -4E, and-5, aminoacyl-tRNA synthase, and ribosomal protein-S6) [442].
Casein kinase-1α phosphorylates Disheveled of the Wnt pathway. It also controls the basal activity of Hh signaling. Casein kinase-1γ associated with the cell membrane binds to and phosphorylates low-density lipoprotein receptor-related protein LRP6 to promote binding of Axin to LRPs and prime Wnt signaling. Casein kinase-1δ is required for Four and a half LIM protein-mediated TGF-β-like responses. Protein kinase-A, -B and Cα and CDC-like kinase CLK2 phosphorylate CK1δ to modulate CK1δ-dependent processes. Casein kinase-1ε also intervenes in the Wnt and Hedgehog pathways and regulation of the circadian rhythm. Isoform CK1ε phosphorylates Disheveled and controls RhoA, Rac, and Rap1 GTPases.
5.6.2 Vaccinia-Related Kinase Family
The mammalian family of vaccinia-related kinases comprises 3 members (VRK1–VRK3). Whereas VRK1 and VRK2 can strongly and modestly autophosphorylate, respectively, as well as phosphorylate casein, VRK3 has key amino acid substitutions in its catalytic activity, so that it is enzymatically inert [443]. Enzymes VRK1 and VRK2 contain C-terminal extracatalytic sequences that mediate intracellular localization. Kinase VRK1 possesses a basic nuclear localization signal and resides in the nucleus. Protein VRK2 is highly hydrophobic and is located in membranes of the endoplasmic reticulum. N-terminal region of VRK3 contains a bipartite nuclear localization signal that determines its nuclear location.
5.6.2.1 Vaccinia-Related Kinase-1
Vaccinia-related kinase-1 (VRK1) belongs to a family of 3 members that has diverged from the casein kinase-1 branch. It can reside in the nucleus. Human VRK1 is expressed mainly in cell lines with high proliferation rates. VRK1 undergoes autophosphorylation. VRK1 is upregulated by E2F and downregulated by retinoblastoma protein and p16 [444].
Kinase VRK1 phosphorylates N-terminus of barrier to autointegration factor (BAF) that is required for assembly of the nuclear envelope, as well as ATF2 transcription factor (Ser62 and Thr73). Because JNK targets Thr69 and Thr71, JNK and VRK1 can have an additive effect on ATF2 phosphorylation and transcriptional activation [444]. Kinase VRK1 binds to the nuclear non-phosphorylated fraction of Jun and phosphorylates Jun (Ser62 and Ser73; Ser73 is targeted by JNK). Together, JNK and VRK1 have an additive effect on Jun dimerization and transcriptional activation. Moreover, VRK1 can form a stable complex with Jun in the nucleus. Lastly, VRK1 phosphorylates P53 (Thr18 that interacts with hdm2).
5.6.2.2 Vaccinia-Related Kinase-2
Vaccinia-related kinase-2 (VRK2) is ubiquitous. Two splice variants exist (VRK2a–VRK2b) [445]. Both full-length VRK2a (VRK2FL; 508 amino acids) and VRK2b (397 amino acids) are regulated by Ran GTPase. Inactive RanGDP inhibits VRK2 kinase activity. In contrast, VRK2 bound to RanGTP is catalytically active. Both VRK2 isozymes undergo autophosphorylation. Human VRK2a protein resides in mitochondrial and endoplasmic reticulum membranes with a preferential perinuclear location. It colocalizes with calnexin and calreticulin. Isoform VRK2b is detected as granular deposits in both cytoplasm and nuclei.
Isoform VRK2a interacts with scaffold Jun N-terminal kinase-interacting protein JIP1 (or MAPK8IP1) in response to either interleukin-1β or hypoxia and represses the signal transmitted by the active complex. Once bound to MAPK8IP1, VRK2 connects to transforming growth factor-β-activated kinase TAK1 (or MAP3K7). The VRK2–MAPK8IP1–MAP3K7 complex can associate with MAP2K7 [445]. On the other hand, VRK2 and MAP3K7 can also interact independently of MAPK8IP1 agent. Both VRK2a and VRK2b phosphorylate P53 factor (Thr18). Substrates of VRK2 also include barrier to autointegration factor.
5.6.2.3 Vaccinia-Related Kinase-3
Unlike other VRKs, vaccinia-related kinase-3 (VRK3) does not possess kinase activity. Pseudokinase VRK3 cannot bind ATP. Protein VRK3 contains a degraded catalytic site, a highly conserved kinase fold, a nuclear localization signal, and a putative regulatory binding site [446]. It has a scaffolding role in the nucleus. It enhances the activity of dual specificity phosphatase DUSP3 that dephosphorylates phosphoERK1 and -2 [447].
Kinase VRK3 interacts with actin monomer-binding protein twinfilin-2.154 Both twinfilin types bind to ADP–Gactin with a higher affinity than ATP–Gactin and prevent actin filament assembly. In mice, twinfilin-1 is the major isoform in embryos and most adult non-muscle cell types, whereas twinfilin-2 is the predominant isoform in the adult heart and skeletal muscles [448]. Whereas actin filament-binding proteins control the nucleation, assembly, disassembly, and crosslinking of actin filaments, ubiquitous actin monomer-binding (-sequestering) proteins, such as profilin, ADF/cofilin, twinfilin, adenylate cyclase-associated protein (CAP), WASP, WAVe, and WASP-interacting protein verprolin, regulate size, localization, and dynamics of the large pool of unpolymerized actin in cells. Twinfilin-2 is involved in neurite outgrowth.
5.6.3 Tau–Tubulin Kinase Family
The microtubule-associated protein Tau, or MAPT, is a microtubule stabilizer encoded by the Mapt gene.155 Its transcript undergoes alternative splicing, thus generating several variant species. It resides mainly at the plasma membrane. Protein Tau promotes microtubule assembly and stability. Its C-terminus binds axonal microtubules; Its N-terminus connects to plasmalemmal components. Its short isoforms (TauS) enables cytoskeleton restructuring. Its long isoforms (TauL) preferentially stabilize microtubules. This constituent of neuronal cytoskeleton binds enzymes and lipoproteins, in addition to microtubules.
Protein Tau is phosphorylated by various kinase types, such as cyclin-dependent protein kinase CDK5, glycogen synthase kinase GSK3β, and Jun N-terminal kinase. Neuronal dysfunction associated with hyperphosphorylation of Tau can result from abnormal activation of CDK5 kinase.
The family of Tau–tubulin kinases that phosphorylate Tau and tubulin includes 2 members (TTbK1–TTbK2).156 Isoform TTbK1 is specifically synthesized in neurons, where it phosphorylates Tau protein. Widespread TTbK2 kinase is involved in spinocerebellar ataxia.
5.6.3.1 TTbK1
Subtype TTbK1 phosphorylates Tau, thereby promoting Tau oligomerization and aggregation. It can activate other Tau kinases, such as microtubule affinity-regulating kinase (MARK), cAMP-dependent protein kinase-A (PKA), and Ca
–calmodulin-dependent protein kinase-2 [449, 450]. Activity of CDK5 depends on inhibitory (Ser9) and activating (Tyr216) phosphorylation. On the other hand, phosphorylation of Tau by TTbK1 (Ser198, Ser199, Ser202, possibly Ser396, and Ser422) may allow Tau phosphorylation by MARK, PKA, or CamK2 (Ser262) [450]. Kinase TTbK1 increases concentrations of CDK5r1 activatory subunit and tubulin polymerization-promoting protein TPPP, improves calpain-1 activity (hence elevated TPPP levels), and reduces the postsynaptic density of hippocampal NMDA-type glutamate receptor GluN2b and GluN2d types.157 Active CDK5 impedes the binding of Src kinase to DLg4 and phosphorylation of GluN2b subunit (Tyr1472) by Src kinase, hence increasing binding of GluN2b to clathrin adaptor AP2 and endocytosis [451].158 Conversely, the phosphorylation of NMDA-type glutamate receptors by Fyn is promoted by Disc large homolog DLg4. The latter bind Src and other SRC family kinases and may facilitate phosphorylation of NMDA-type glutamate receptors by SRC family kinases. Protein CDK5 phosphorylates DLg4, hence controlling the binding of Src to DLg4 agent [451].

Neuron-specific enzyme TTbK1 provokes dissociation of CDK5r1 from Factin, thereby relieving sequestration by Factin [450]. Another activatory subunit, CDK5r2, can bind to the actin cytoskeleton. Actin depolymerization increases of CDK5r2–CDK5 activity [450]. Conversely, CDK5 regulates dendritic remodeling via the regulation of Factin formation that relies on phosphorylation of actin regulators, such as WAVe1, cyclin-dependent kinase inhibitor CKI1b, PP1r9a, and RhoGEF27 agent.
5.6.3.2 TTbK2
Mutations in the gene that encodes Tau–tubulin kinase TTbK2 cause type-11 spinocerebellar ataxia [452]. Autosomal dominant spinocerebellar ataxias constitute a heterogeneous group of neurodegenerative disorders characterized by poor coordination, abnormal eye movements, and impaired speech and swallowing. Spinocerebellar ataxia type 11 is a pure progressive cerebellar ataxia with peripheral neuropathy. SCA11 truncating mutations promote TTbK2 production, suppress kinase activity, and enhance its nuclear localization [453]. Mutations cause premature termination of the TTbK2 protein, thereby eliminating most of the non-catalytic part of the enzyme, but conserving the kinase catalytic domain.
5.7 Family-2 Casein Kinases
Kinase CK2 is a ubiquitous, constitutively active, protein Ser/Thr kinase that is constituted by tetramers formed by CK2α1, CK2α2, and CK2β subunits. The latter is encoded by the CSNK2B gene, whereas CK2α1 and CK2α2 are encoded by the CSNK2A1 and CSNK2A2 genes. Tetramer CK2 consists of 2 catalytic α (2 identical catalytic subunits [2 homodimer types] or CK2α1–CK2α2 heterodimer) and 2 regulatory β subunits that undergo autophosphorylation. The CK2α activation loop is kept in an active conformation without phosphorylation or interaction with regulatory β subunits via unique interactions between N-terminal segment and catalytic core.
Subunits of CK2 either form CK2 heterotetramers or complex with other proteins [454]. Some substrates can only be phosphorylated by free catalytic subunits, whereas others require formation of tetramers [454]. None of the CK2α2 partners exhibit isoform specificity.
In vitro, CK2 is inhibited by polyanionic compounds such as heparin and is stimulated by polycationic compounds, such as polyamines and polybasic peptides [454]. Inositol phosphates can also regulate CK2 enzyme. Kinase CK2 localizes in the nucleus and cytoplasm. It behaves as an ectokinase, as it operates in concert or consecutively with multiple components of cellular structures and organelles, such as plasma membrane, endoplasmic reticulum, Golgi body, cytoskeleton, nuclear matrix, nucleolus, and mitotic spindle [454].
Kinase CK2 contributes to the regulation of various cellular processes, such as transcription, protein translation and processing, cell polarity, transformation, and apoptosis [455]. Casein kinase-2 also participates in cell cycle control and DNA repair, as well as regulates the circadian rhythm. However, CK2α1, but not CK2α2, is phosphorylated in a cell cycle dependent manner. In addition, casein kinase-2 is stimulated by activated Wnt pathway.
5.7.1 Catalytic CK2α1 and CK2α2 Subunits)
Although CK2α1 and CK2α2 are catalytically active in the absence of CK2β, CK2β heightens catalytic activity. Kinase CK2 interacts with numerous molecules. Peptidyl-prolyl cis/trans isomerase Pin1 that regulates proline-directed kinase signaling (e.g., MAPK, CDK, and GSK3), pleckstrin homology domain-containing protein PlekHO1 (or casein kinase-2 interacting protein CKIP1) that connects to SMAD ubiquitination regulatory factor SMURF1 (but not SMURF2) to promote ubiquitination, and PP2 interact with CK2α1, but not CK2α2 [454].
On the other hand, many CK2α1-interacting proteins also bind to CK2α2, such as nucleolin that is involved in the synthesis and maturation of ribosomes, tubulin, ATF1 transcription factor, ubiquitin conjugase UbC3b, and receptor protein tyrosine phosphatase PTPRc [454].
Kinase CK2α2 also targets an accessory factor complex for access to DNA packaged into chromatin, the so-called chromatin-specific transcription elongation factor FACT, a heterodimer that consists of suppressor of Ty 16 homolog SupT16H159 and structure-specific recognition protein SSRP1 and links specifically to histones H2A and H2B for nucleosome disassembly and transcription elongation [455]. The CK2–FACT complex acts as a protein kinase that is activated by certain cell stresses that generate DNA damage.
5.7.2 Regulatory CK2β Subunit
Subunit CK2β has both positive and negative modulatory effects on the activity of the catalytic subunits. Activation is mediated by CK2β C-terminal domain, whereas inhibition is conferred by its N-terminal domain [456].
Subunit CK2β can be involved in many cellular processes, such as transcription, protein translation and processing, cell polarity, cell cycle control, cell transformation, and apoptosis. Subunit CK2β does not necessarily tethers to other subunits to form CK2 tetramers and can then interact with other proteins [456].
Subunit CK2β is phosphorylated both at autophosphorylation sites and at a CDK1 site. Autophosphorylation does not influence the catalytic activity of CK2 but can affect CK2β turnover [456]. Subunit CK2β can operate as a docking element to recruit CK2 tetramers to specific cellular sites or proteic complexes that modulate phosphorylation state of cognate proteins. It can also bind regulators, such as histones, fibroblast growth factor-2, and polyamines that adjust the activity of tetrameric CK2. Lastly, CK2β is also involved in CK2-independent interactions with other proteins [456]. The CK2β interactors include Raf kinase isoform aRaf of the MAPK module, oocyte maturation factor Mos, and ChK1 checkpoint kinase.
5.8 CMGC Superfamily
5.8.1 Cyclin-Dependent Kinase Family
Cyclin-dependent kinases (CDK) are Ser/Thr protein kinases that are activated by association with cyclins (Vol. 2 – Chap. 2. Cell Growth and Proliferation). Cyclin-dependent kinases and corresponding cyclins form heterodimeric enzymes. The Ccn–CDK complexes are regulated by kinases and phosphatases. Cyclin-dependent kinases, except CDK9, regulate the cell cycle as well as transcription and mRNA processing.
Cyclin-dependent kinase CDK5 activated by activator p35 following DNA damage phosphorylates ataxia telangiectasia mutated kinase that coordinates DNA-damage responses, such as cell cycle checkpoint control, DNA repair, and cell apoptosis [457]. The CDK5–ATMK signal regulates phosphorylation, hence function of ATMK targets P53 and histone-2AX.
The CDK9–CcnK complex, but not the CDK9–CcnT complex, functions in a DNA-damage response pathway to maintain genome integrity and promote replication fork recovery from a transient arrest [458].
5.8.2 Homeodomain-Interacting Protein Kinase Family
Homeodomain-interacting protein proline-directed Ser/Thr kinases constitute the HIPK family (HIPK1–HIPK4). They regulate cell proliferation and apoptosis, as they modulate the Wnt and P53 pathways. The HIPK kinases can also regulate gene expression by phosphorylating transcription factors and accessory components of the transcription machinery.
Members of the HIPK family localize to nuclear speckles. They phosphorylate several types of proteins, such as homeodomain transcription factor NKx1-2 and intracellular androgen receptor, in addition to P53 factor. Site-specific phosphorylation of P53 regulates its activity. In humans, HIPK2 phosphorylates P53 (Ser46) and the corepressor C-terminal-binding protein (CTBP; Ser422) to trigger apoptosis; HIPK2 also phosphorylates P53 (Ser9).
5.8.2.1 HIPK1
Four isoforms of P53-binding homeodomain-interacting protein kinase HIPK1 result from alternative splicing. In resting endothelial cells, HIPK1, Disabled homolog-2 (Dab2)-interacting protein (Dab2IP or apoptosis signal-regulating kinase ASK1 [MAP3K5]-interacting protein AIP1), and MAP3K5 lodge primarily in the nucleus, plasma membrane, and cytoplasm, respectively. Cytokine TNFα causes HIPK1 desumoylation and translocation from the nucleus to the cytoplasm [459]. In synergy with Dab2IP, it then activates MAP3K5 by provoking release of its inhibitors (thioredoxin, glutaredoxin, glutathione S-transferase-μ1, heat shock proteins, and 14-3-3 proteins) and launching MAP3K5 oligomerization and autophosphorylation. Subsequently, MAP3K5 stimulates MAP2K3 and MAP2K7 as well as MAP2K4 and MAP2K7 that operate on JNKs and P38MAPKs, respectively [459].
5.8.2.2 HIPK2
Kinase HIPK2 has a high turnover in unstressed cells, as its short half-life results from degradation after ubiquitination by ubiquitin ligases Seven in absentia homolog SIAH1 and SIAH2, WD-repeat and SOCS box-containing protein WSB1, double minute DM2, and Skp1–Cul1–F-box SCF3 [460]. It is mainly a nuclear kinase that acts as both transcriptional activator and repressor, as it phosphorylates transcription factors as well as coactivators and corepressors. Isokinase HIPK2 regulates cell apoptosis in response to DNA damage, particpates in cell response to hypoxia and morphogenetic signals (Wnt, TGFβ, BMP, Notch, and Sonic hedgehog) [460]. The Hipk2 mRNA abounds in human tissues with its highest values in neurons. At protein level, HIPK2 expression is almost undetectable in unstressed cells and increases in the presence of genotoxic stress.
Under normoxia, HIPK2 phosphorylates SIAH2 and subsequently weakens mutual binding [461]. Isozyme HIPK2 is then protected from SIAH2-mediated ubiquitination and subsequent proteasomal degradation. Hypoxia favors association of SIAH2 with prolyl hydroxylating domain-containing proteins PHD1 and PHD3 that leads to proteasomal degradation of these HIF-prolyl hydroxylases, hence avoiding hypoxia-inducible factor elimination. Transcription factor HIF1α can accumulate and trigger gene expression. Furthermore, hypoxia provokes polyubiquitylation of transcription repressor HIPK2 and subsequent proteasomal degradation. Because HIPK2 impedes gene expression, oxygen deprivation causes reprogramming of gene expression pattern. However, a non-interacting pool of kinase HIPK2 escapes from degradation even under hypoxia.
Upon DNA damage that disrupts linkage between HIPK2 and its ubiquitin ligase WSB1, HIPK2 is stabilized by ataxia telangiectasia mutated kinase that phosphorylates SIAH1 and activated by activated protein kinases ATMK and ATRK to trigger apoptosis [460]. In response to severe DNA damage, HIPK2 phosphorylates pro-apoptotic P53 (Ser46) and anti-apoptotic corepressor C-terminal-binding protein CtBP (Ser422), hence priming its proteasomal degradation. It also interacts with histone acetyltransferases CREB-binding protein and P300 for P53 acetylation, thereby raising P53 transcriptional activity. Scaffold Axin forms the Axin–HIPK2–P53 complex to stimulate phosphorylation (activation) by HIPK2 of P53 factor. Axin also bridges Fas (TNFRSF6a)-binding Death-associated protein DAP6 (or Daxx)160 and P53 to augment HIPK2-mediated P53 activation and cause cell death. Transcriptional regulator promyelocytic leukemia (PML) stimulates HIPK2 phosphorylation of P53 factor. Kinase HIPK2 phosphorylates PML (Ser8 and Ser38) to trigger PML sumoylation and its cooperation with HIPK2 for cell death. Moreover, PML stimulates stabilizes HIPK2 and P300, as it inhibits their SCF3-induced ubiquitination and subsequent proteasomal degradation. In addition, HIPK2 activates the pro-apoptotic JNK pathway. During the recovery phase of weak DNA damage, HIPK2, which accumulates upon repression of SIAH1- and WSB1-mediated elimination of HIPK2, is degraded via a P53–SIAH1-dependent mechanism.
According to the molecular context, HIPK2 activates or represses transcription in response to differentiation and development stimuli. Kinase HIPK2 is a corepressor for NK1 and NK3 transcription factors in cooperation with corepressor Groucho [460]. It phosphorylates Paired box Pax6 transcription factor and enhances Pax6–p300 interaction to promote the recruitment of p300 to specific promoters. It also phosphorylates p300 transcriptional regulator. In addition, HIPK2 is involved in Wnt1-dependent phosphorylation and subsequent degradation of MyB transcriptional activator in immature hematopoietic cells. Upon BMP signaling, HIPK2 binds both transcriptional regulators Ski and SMAD1 to inhibit SMAD1–SMAD4-induced transcriptional activation. Kinase HIPK2 also intervenes in TGFβ signaling. Furthermore, HIPK2 promotes hematopoietic gene transcription, as it phosphorylates RUNX1 transcription factor and then p300 histone acetyltransferase. It also forms a corepressor complex with Groucho and HDAC1 histone deacetylase. Kinase HIPK2 also colocalizes with Four and a half LIM domains FHL2 and can form a HIPK2-FHL2–P53 complex.
5.8.2.3 HIPK3
Kinase HIPK3 is involved in basal and cAMP-stimulated (upon binding of the pituitary peptide adrenocorticotropic hormone to its cognate plasmalemmal receptor) production of the steroidogenic CYP11A1 gene that encodes cytochrome-P450-11a1 [462]. Enzyme HIPK3 operates via Jun N-terminal kinase, which phosphorylates Jun transcription factor. In cooperation with JunP, it launches activity of steroidogenic factor-1, or NR5a1. In addition, HIPK3 interacts with another nuclear receptor, androgen receptor, or NR3c4.
5.8.2.4 HIPK4
In humans, HIPK4, which is produced in the lung and white adipose tissue, can phosphorylate P53 (Ser9) for P53-mediated transcriptional repression [463]. Kinase HIPK4 prevents P53-dependent activity of survivin gene promoter, survivin being also called apoptosis inhibitor-4 and baculoviral IAP repeat-containing protein BIRC5.
5.9 TKL Superfamily
5.9.1 Integrin-Linked Kinase
Integrin-linked kinase (ILK or ILK1) is a ubiquitous Ser/Thr kinase that participates, rather as a pseudokinase [168, 465] (Sect. 3.4.3) and a regulator of integrin-mediated signaling,161 in multiple cellular functions, such as cell proliferation, adhesion, and migration, as well as cadherin expression and pericellular fibronectin matrix assembly. It interacts mainly with the cytoplasmic domain of β1-integrins to regulate integrin-mediated signal transduction, especially signaling from growth factors and Wnt morphogens.
Integrin-linked kinase contributes to cell adhesion-dependent cell cycle progression, as it regulates the level or activity of several components of the cell cycle, such as cyclin-A and -D1 and CDK4 [466]. It localizes to the centrosome and can regulate mitotic spindle organization.
Integrin-linked kinase can interact with several focal adhesion proteins, such as β1 integrins, PINCH,162 parvins, and paxillin (Table 5.19). Together with PINCH and NCK2, ILK couples and uncouples regulatory networks of cellular processes, such as cell morphology, migration, and survival [467]. Focal adhesion adaptor PINCH-1 (a.k.a. LIM and senescent cell antigen-like-containing domain proteins LIMS1) thus operates as a coupling–uncoupling platform for diverse cellular processes via its distinct LIM domains.
Table 5.19
Interactome of ILK (Source: [465]; ELMO: engulfment and cell motility; ILKAP: ILK-associated Ser/Thr phosphatase [of the PPM1 family]; kAE: kidney anion exchanger [chloride–bicarbonate exchange in basolateral surface of acid-secreting α intercalated cells in the collecting duct of the nephron]).
Partner | Effect |
---|---|
β1-integrin | Growth |
β3-integrin | Platelet aggregation |
kAE1 | AE1–Actin linkage |
ELMO2 | Cell polarity and migration |
EPHa1 | Cell shape and motility |
ILKAP | Regulation of GSK3β signaling, Wnt signaling |
Kindlin-2 | Recruitment of ILK to focal adhesion |
Parvins | Cell adhesion, mural cell coating of blood vessels |
Paxillin | Recruitment of ILK to focal adhesion |
PINCH-1/2 | Cell spreading and migration |
PKB1 | PKB1 phosphorylation, cell survival |
Rictor | PKB phosphorylation, cell survival |
RuvBL1 | Spindle assembly |
Src | Cofilin phosphorylation, actin organization |
Thymosin-β4 | Actin polymerization, PKB phosphorylation |
Integrin-linked kinase interacts with PINCH adaptors (PINCH1–PINCH2)163 to be connected to receptor Tyr kinases and lipid kinases, especially during cell migration [468].164 The ternary NCK2–PINCH–ILK complex is involved in signaling pathways from growth factors via their cognate receptor Tyr kinase and small GTPases.165 Adaptor PINCH interacts with NCK2 adaptor protein that participates with small GTPases and growth factor receptors in signaling pathways, as it tethers to IRS1, EGFR, and PDGFR [468]. It is activated by phosphatidylinositol (3,4,5)-phosphate synthesized by PI3K kinase. Conversely, it is inhibited by PIP3 dephosphorylation by PTen phosphatase. Integrin-linked kinase can inactivate myosin phosphatase and phosphorylate myosin light chain.
Members of the parvin family, i.e., α- (or actopaxin),166 β- (or affixin) that is primarily expressed in myocytes, especially in the heart and skeletal muscle, and γ-parvin produced in hematopoietic cells, are actin-binding proteins that are associated with integrin clusters, the so-called focal adhesions. Parvin-α is involved in stabilization of vessel walls during angiogenesis as well as in mature vessels by vascular smooth muscle cells. Parvin-α limits the activity of the RhoA–RoCK axis that causes fast, random motility167 to avoid hypercontractility and a loss of directed migration toward developing vessel wall or suitable coverage and permeability of mature vessels [469].
The ternary ILK–PINCH–parvin complex (IPP) couples integrins to the actin cytoskeleton. The assembly of the IPP complex precedes integrin-mediated cell adhesion [465]. The IPP complex contributes to adhesion strengthening and organization of the actin cytoskeleton downstream from integrins. Distinct IPP complexes formed by PINCH and parvin isoforms have specific functions in modulation of integrin signaling. Parvin-α forms a ternary complex with ILK and PINCH adaptor in adhesion sites between cells and the extracellular matrix [470]. The formation of the PINCH–ILK–α-parvin complex, an effector of protein kinase-C, is necessary, but not sufficient for ILK localization to cell–matrix adhesion sites.
5.9.2 Interleukin-1 Receptor-Associated Kinase Family
Interleukin-1 receptor-associated kinases (IRAK1–IRAK4) were first described as signal transducers for interleukin-1. However, they are effectors of other members of the TIR (Toll-like [TLR] and interleukin-1 [IL1R]) receptor family that couple signaling events from plasmalemmal and endosomal receptor complexes to cytosolic signalosomes (Tables 5.20 and 5.21). Toll-like receptors are pattern recognition receptors of the innate immunity involved in pathogen sensing; IL1 cytokine triggers inflammation. Intracellular kinases of the IRAK family participate in innate immune responses via various signaling axes that regulate inflammation, antiviral response, and subsequent activation of the adaptive immunity, as well as in the inhibitory control of autoimmunity and chronic inflammation.
Table 5.20
Interleukin-1 receptor-associated kinases (IRAK) downstream from activated interleukin (ILR) and Toll-like (TLR) receptors (Source: [471]). Toll-like receptors localize either to the plasma membrane, such as TLR1, TLR2, and TLR6, which recognize lipoproteins, and TLR4, which targets lipopolysaccharide (LPS), or to endosomes, such as TLR3, TLR7, TLR8, and TLR9 that bind to double stranded RNA (dsRNA [TLR3]), single stranded RNA (ssRNA [TLR7 and TLR8]), and cytidineP–guanosine (CpG) motifs in DNA (TLR9). Liganded receptors dimerize and recruit TLR–IL1R (TIR) domain-containing adaptors (MAL: MyD88 adaptor-like protein; MyD88: myeloid differentiation primary response gene product-88; SArm: sterile-α- and armadillo motif-containing protein; TRAM: TRIF-related adaptor molecule; TRIF: TIR domain-containing adaptor inducing Ifnβ). Isoform IRAK4 interacts with MyD88 using death domain and may phosphorylate IRAK1 and IRAK2 that then autophosphorylate. Once IRAK1 and IRAK2 are released from the receptor complex, they connect to TNFR-associated factor-6 (TRAF6). Subunit IKKγ binds to ubiquitinated IRAK1 protein. Isoform IRAK2 causes TRAF6 polyubiquitination that enables the recruitment of the MAP3K7–MAP3K7IP2 complex; MAP3K7 activates the IKK complex. It also stimulates MAP2K3 and MAP2K6 that target P38MAPKs as well as MAP2K4 and MAP2K4 that identify JNKs, respectively. Scaffolding proteins TANK (TRAF-associated NFκB activator), NAP1 (NFκB-activating kinase-associated protein), and Sintbad (similar to NAP1 TBK1 [TANK-binding kinase-1] adaptor), assemble TBK1 and IKK complex. The IKK complex phosphorylates NFκB1, a MAP3K8 inhibitor, hence activating MAP3K8 that stimulates MAP2K1 and MAP2K2, which then phosphorylates ERK1 and ERK2 kinases. Kinases TBK1 and IKK phosphorylate interferon (Ifn) regulatory factors IRF3 and IRF7. Therefore, IRAKs provoke activation of NFκB, MAPK modules, and IRF factors.
Receptor | Adaptors and partners | Mediators | Targets |
---|---|---|---|
Plasmalemmal signaling | |||
TLR1/2/6 | MyD88, IRAK1/2/4 | TRAF6, MAP3K7, | MAP2K3/6–P38MAPK |
MAP3K7IP1/2/3 | MAP2K4/7–JNK | ||
Rac, PI3K, PKB, | NFκB, | ||
IKK | MAP3K1/2–ERK1/2 | ||
TLR4 | MyD88, IRAK1/2/4, | TBK1, | Inflammasome, MAPK, |
TRIF, TRAM, BTK | IKKε | NFκB, IRF3 | |
TLR5 | MyD88, IRAK1/2/4 | TRAF6, etc. | NFκB, |
MAP3K3–P38MAPK | |||
IL1R, IL18R | MyD88, IRAK1/2/4 | TRAF6, etc. | NFκB, MAPK |
Endosomal signaling | |||
TLR3 | TRIF, RIPK1, IRAK2 | TANK, TBK1, | NFκB, IRF3/7 |
TRAF3, IKKε, | |||
Sintbad | |||
TLR7/8/9 | MyD88, IRAK1/4, TRAF3/6 | IKKα | NFκB, IRF5/7 |
Table 5.21
Partners of members of the IRAK family (Source: [472]). Some endocytic adaptors enable intracellular signaling. These adaptors are involved in the assembly of signalosomes as well as receptor cargo sorting (BTK: Bruton Tyr kinase; LPS: lipopolysaccharide; MAL: MyD88 adaptor-like protein; MyD88: myeloid differentiation primary response gene product-88; SIGIRR: single immunoglobulin [Ig] domain-containing and Toll-like and interleukin-1 receptor [TIR]-related protein, or TIR receptor TIR8; SOCS: suppressor of cytokine signaling protein; Tollip: Toll-interacting protein). Subtype IRAK4, the most receptor-proximal kinase, acts downstream from MyD88 and MAL and upstream from TRAF6 in TIR signaling. It phosphorylates (activates) only IRAK1 among members of the IRAK family. Whereas IRAK1 is an IRAK4 stimulator and IRAK2a and IRAK2b enhance IRAK4-mediated signaling, IRAK2c, IRAK2d, and IRAK3 are IRAK4 inhibitors.
Partner | Role and events |
---|---|
BTK | Interaction with MyD88, MAL, and IRAK1 during LPS |
stimulation | |
IRAKs | IRAK2a/2b stimulates, IRAK2c/2d/3 inhibits IRAK4 |
MyD88 | Inhibition of IRAK4 recruitment to the receptor complex |
Mediation of apoptosis with IRAK2 | |
Pellino-1–3 | Ubiquitin–protein ligases |
Component of the Pellino–IRAK1–IRAK4–TRAF6 complex | |
NFκB activation in response to IL1 | |
SOCS1 | Suppression of TLR4 signaling |
Inhibition of IRAK4 | |
Tollip | Component of the sorting machinery for ubiquitinated |
proteins in the endosome, IRAK1 degradation |
5.9.2.1 IRAK Interactors
Members of the IRAK family interact with many molecules involved in signal transduction downstream from TLR–IL1R (TIR) receptors [472] (Table 5.21). Type-1 interleukin-1 receptor (IL1RI) is a master regulator of inflammation and innate immunity. Once it is bound to IL1β, IL1R1 complexes with IL1R-associated protein and forms a plasmalemmal signalosome that potently activates signaling cascades. Once IL1R1 is internalized, activated receptors are often sorted via endosomes and delivered to lysosomes for degradation due to labeling ensured by ubiquitination of cargos as well as protein-sorting complexes.
Toll-like receptor-1, -2, and -4 to -6 lodge on the cell surface; their ectodomains bind pathogen-associated molecular patterns, such as lipopolysaccharide and flagellin, which are exposed on the exterior of invading microbes. Other TLRs (TLR3 and TLR7 to TLR9) reside in endosomes, on which they detect nucleic acids from pathogens. Stimulation of TLR by pathogen-associated molecular patterns permits the recruitment of the IRAK1–IRAK4 complex as well as inhibitory IRAKs to the signaling complex. Inhibitory IRAKs preclude the release of IRAK1–IRAK4 subcomplex from the TLR signaling complex. The engagement of TLR ectodomains by cognate ligands also enables the recruitment of TIR domain-containing adaptors to the cytoplasmic TIR domains of TLRs. This process triggers coordinated activation of transcription factors, such as nuclear factor-κB and interferon regulatory factors (IRF), as well as mitogen-activated protein kinase (MAPK) modules for efficient elimination of invading microorganisms.
Myd88
Both myeloid differentiation primary response protein MyD88 and its splice variant MyD88S are ubiquitous. Adaptor MyD88 impedes IRAK4 recruitment to the receptor complex. In TLR signaling, MyD88 supports cell death together with IRAK2, whereas IRAK1 and TRAF6 are involved in the survival pathway that leads to NFκB activation (Sect. 10.9.1) via the TRAF6–IKK complex [473].
BTK
TIR domain-binding kinase BTK intervenes in B-cell maturation as well as mastocyte activation via high-affinity IgE receptor. Bruton Tyr kinase connects in particular to the TIR domain of TLR4, TLR6, TLR8, and TLR9. During BCR-mediated signaling, BTK is activated by members of the SRC family, such as Fyn, Lyn, and HCK kinases [474]. It binds PIP3 and subsequently phosphorylates phospholipase-C that, in turn, hydrolyzes PIP2 into IP3 and DAG messagers. Moreover, it interacts with MyD88, MAL, and IRAK1 during stimulation by lipopolysaccharides [473].
TRAF and Pellino Ubiquitin Ligases
Ubiquitination contributes to the regulation of mediators involved proteins in signal transduction cascades triggered by ILRs and TLRs that activate proper transcription factors and MAPK modules. In addition to the Ub ligases Tumor-necrosis factor receptor (TNFR)-associated factors (TRAF), Pellino Ub ligases catalyze polyubiquitination of IRAK molecules. Synthesis of Pellino-1 relies on interferon regulatory factor IRF3 [475].
According to the type of ubiquitin conjugase that works in combination with Pellino-1, the latter can form Lys48- or Lys11-linked polyubiquitin chains [476]. However, when Pellino proteins act with IRAK1, they only generate Lys63-linked polyubiquitination of IRAK1 kinase.
Pseudokinases of the IRAK family (e.g., IRAK1b, a splice variant of IRAK1, IRAK2, and IRAK3) may fail to interact with Pellino proteins.
Pellino-1 and -2 that interact with IRAK1 are required for NFκB activation during TIR signaling [474]. Pellino-1 and -2 are also substrates of IRAK4 [476]. Once Pellino isoforms are phosphorylated by IRAK1 and IRAK4, they act as ubiquitin ligases.
Active IRAK kinases can promote polyubiquitination of Pellino proteins, thus causing Pellino degradation. In addition, both IKKεand TBK1 phosphorylate (Ser76, Thr288, and Ser293; activate) Pellino-1. Pellino proteins can also interact with TRAF6 and MAP3K7 [476].
Stimulated TLRs or IL1R promote the association of Pellino proteins with IRAK1 and TRAF6 effectors. Kinase IRAK1 phosphorylates (activates) Pellino proteins, thereby causing Lys63-linked polyubiquitination of IRAK1, which can then binds to IKKγ [476]. Lys63-linked polyubiquitination of TRAF6 facilitates recruitment and activation of the MAP3K7–MAP3K7IP1/2/3 complex. The IKK and MAP3K7 complexes are thus bridged. Enzyme MAP3K7 activates IKK, hence stimulating NFκB and releasing MAP3K8 that launches the ERK1/2 cascade. In addition, MAP3K7 can also boost the P38MAPK and JNK pathways via corresponding MAP2Ks.
Once connection of IRAK1 and TRAF6 to the receptor complex is disrupted, TRAF6 interacts with TRAF-interacting protein with a forkhead-associated (FHA) domain (TIFA), which promotes TRAF6 oligomerization and ubiquitination, as TRAF6 also connects to a suitable ubiquitin conjugase [476]. Whereas the TRAF6–MAP3K7–MAP3K7IP complex moves to the cytosol, IRAK1 remains at the plasma membrane and is subsequently degraded.
SIGIRR
Widespread (but not ubiquitous as it lacks in macrophages), transmembrane protein Single immunoglobulin (Ig) domain-containing and Toll-like and IL1 receptor (TIR)-related protein SIGIRR, or TIR receptor TIR8, links to both IRAK1 and TRAF6 agents. It may prevent signaling from the IRAK1–TRAF6 complex [473].
SOCS1
Suppressor of cytokine signaling SOCS1 can suppress TLR4 signaling, as it interacts directly or not with IRAKs. It regulates protein turnover, as it targets proteins for polyubiquitination and degradation.
Tollip
Toll-interacting protein — Tollip — is an adaptor and substrate for IRAK1. In resting cells, Tollip forms a complex with members of the IRAK family, thereby preventing NFκB activation, as it hinders IRAK1 phosphorylation. After receptor activation, Tollip–IRAK1 complex is recruited to the receptor, thereby causing the rapid IRAK1 autophosphorylation and its dissociation from the receptor. In addition, Tollip is phosphorylated by IRAK1 upon receptor engagement. Once it is activated, Tollip precludes IRAK1 activity (negative feedback loop). Ubiquitination of IRAK1 is controlled by Tollip-interacting protein, Target of MyB1 (TOM1), which is also a ubiquitin- and clathrin-binding protein. Tollip is required for sorting of IL1R1 to late endosomes. Agent TOM1 interacts with IL1R1 to avoid accumulation of IL1R1 in late endosomes [473]. Tollip may act as an endosomal adaptor that links IL1R1 via TOM1 to the endosomal degradation machinery.
5.9.2.2 IRAK Proteins in Cell Signaling
Functional domains of IRAK proteins can comprise a death domain for MyD88 linkage, a proST motif rich in prolines, serines, and threonines, which is required for autophosphorylation and can be hyperphosphorylated, a kinase site, and a C-terminus for interaction with TRAF6 ubiquitin ligase. Subtype IRAK1 contains 3 TRAF6-binding motifs, IRAK2 2, IRAK3 1; IRAK4 lacks this motif [471].
Once interleukin-1 binds to type-1 IL1 receptor (IL1R1), the latter connects to IL1R1 accessory protein (IL1RAcP) that converts silent IL1R1 into an active receptor. The IL1R1–IL1RAcP (receptor–coreceptor) complex then recruits adaptor MyD88 that attracts IRAKs [477, 478]. Kinase IRAK is highly phosphorylated, interacts with ubiquitin ligase TRAF6, then leaves the receptor complex. Agent TRAF6 bears autoubiquitination (Lys63). The IRAK–TRAF6 complex initiates the assembly of a signalosome that includes MAP3K7 (TAK1). The MAP3K7 (TAK1)-binding proteins MAP3K7IP2 (TAB2) and/or MAP3K7IP3 (TAB3) binds ubiquitinated TRAF6 agent. Kinase MAP3K7, in turn, activates IκB and Jun N-terminal kinases that phosphorylate IκB and Jun, respectively. Receptor TLR4 also primes interactions between MyD88, IRAK, and TRAF6 proteins.
Among the 4 known members of the IRAK family, only IRAK1 has a potent kinase activity. Yet, IRAK1 can act primarily as an adaptor for TRAF6. Whereas IRAK1, IRAK2, and IRAK4 are ubiquitous, inducible IRAK3 is mainly detected in monocytes and macrophages (hence its other alias IRAKm) [477]. Kinase IRAK4 can act as an IRAK1 activator [479]. Isozyme IRAK4 actually interacts with IRAK1 and TRAF6 to activate NFκB transcription factor and MAPK pathways. Isoform IRAK4 tethers to adaptor MyD88 to form the so-called myddosome (IRAK4–MyD88 complex) via their death domains [481].
Phosphatidylinositol 3-kinase controlled by membrane IP3 and its activating effector PKB positively or negatively regulate transcription factor NFκB activity in a cell- and ligand-specific manner. Protein kinase-B phosphorylates IKKα subunit of IκB kinase (IKK; i.e., IKKα–IKKβ–IKKγ complex), but not IKKβ. However, PI3K can act without stimulating IKK. Tumor-necrosis factor-α, interleukin-1, insulin growth factor-2, platelet-derived growth factor, bradykinin, interferon-α and -β, and N-formylmethionyl-leucyl-phenylalanine168 provoke activation of IκB kinase that phosphorylates inhibitor of NFκB (IκB) for degradation, hence eliciting NFκB activity via the PI3K–PKB axis together with other mediators. On the other hand, lipopolysaccharide- or cytokine-induced stimulation of production of inducible nitric oxide synthase (NOS2) that is controlled by NFκB is hampered by PI3K–PKB signaling. Protein kinase-B can be phosphorylated (activated) by calcium–calmodulin-dependent Cam2Kα to phosphorylate IRAK1, thereby reducing its association with MyD88 and precluding IL1β activation of NFκB via IκB kinase-β excitation [478].
Upon stimulation of interleukin-1 and some Toll-like receptors, IRAK1 and TRAF6 are ubiquitinated. Ubiquitin ligase TRAF6 causes Lys63-linked polyubiquitination of IRAK1 that is required for IKK recruitment and activation [480]. Only polyubiquitinated IRAK1 (Lys134 and Lys180) actually binds the regulatory IKKγ subunit of IκB kinase to activate NFκB.
5.9.2.3 IRAK1
In humans, ubiquitous IRAK1 has 3 splice variants. It interacts with MyD88 adaptor and can homodimerizes [471]. It is phosphorylated by IRAK4 kinase. Once it is phosphorylated and autophosphorylated, IRAK1 is released from the receptor complex and binds to TRAF6, thereby enabling NFκB activation. It is also ubiquitinated and sumoylated. Its Lys48-linked polyubiquitination causes its rapid degradation. On the other hand, Lys63-linked polyubiquitination allows interaction with IKKγ subunit.
Subtype IRAK1 regulates ubiquitination by TRAF6 of IRF5 [471]. It cooperates with STAT3 and tethers to the IL10 promoter. It also contributes to STAT1 phosphorylation during IL1 stimulation.
5.9.2.4 IRAK2
Isoform IRAK2 interacts with MyD88 and MAL adaptors as well as TRAF6 Ub ligase. It functions redundantly with IRAK1 in early signaling, but plays an important role in late, sustained NFκB and MAPK activation [471]. Subtype IRAK4 phosphorylates IRAK2 to prime its kinase activity.
5.9.2.5 IRAK3
In humans, IRAK3 is produced in monocytes and macrophages. It contributes to the regulation of the NFκB axis. In addition, IRAK3 prevents P38MAPK activation by TLR2, but not JNK or ERK activation [471]. It operates independently of IRAK1 via dual-specificity phosphatase DUSP1, or MAPK phosphatase MKP1. In addition, IRAK3 represses the non-canonical, MAP3K14-dpendent NFκB pathway [471].
5.9.2.6 IRAK4
Isoform IRAK4 also interacts with MyD88 to form the myddosome (7–8 MyD88 complexed to 4 IRAK4 proteins), which may recruit IRAK2 or IRAK3 [471]. Subtype IRAK4 phosphorylates IRAK1, thereby enabling IRAK1 autophosphorylation (activation). However, IRAK4 also primes IRAK1 degradation to regulate the MyD88-mediated signaling (negative feedback loop) [471]. In addition, IRAK4 interacts with IRAK2 isoform. Activity of IRAK4 kinase is necessary for activation of the MAPK module and JNK enzymes downstream from IL1R, TLR2, TLR4, and TLR7 [471].
5.9.3 LIM Domain-Containing Kinase Subfamily(LISK [LIMK–TESK] Family)
The LIMK subfamily includes 2 paralogs (LIMK1–LIMK2). These LIM domain-containing kinase isoforms regulate actin polymerization via phosphorylation (inactivation) of actin-depolymerizing factors cofilin-1 and -2 and cofilin-related destrin to accumulate actin filaments.
Ubiquitous LIMK1 and LIMK2 can form heterodimers with potential transphosphorylation. Both paralogs have splice variants for augmented diversity.
5.9.3.1 LIMK1
The activity of LIMK1 is regulated by small GTPases Rho, Rac, and CDC42 via their effector kinases RoCK and P21-activated kinases PAK1 and PAK4 that phosphorylate LIMK1 (Thr508 of kinase domain) [482]. In addition to its action on actin dynamics, LIMK1 participates in the regulation of microtubule dynamics, as it phosphorylates (inhibits) tubulin polymerization-promoting protein (TPPP), hence provoking microtubule disassembly.
Stability of LIMK1 is enhanced by transphosphorylation. Binding of heat shock protein Hsp90 to LIMK1 promotes its homodimerization and transphosphorylation, thereby heightening its stability and activity [482]. Paralog LIMK1 is also phosphorylated (Ser323; activated) by mitogen-activated protein kinase-activated protein kinase MAPKAPK2 during VEGF stimulation of endothelial cells. Last, but not least, LIMK1 phosphorylates transcription factor CREB1 and interacts with nuclear receptor-related factor NR4a2 (NuRR1).
Many factors depress LIMK1 ability to phosphorylate cofilin [482]: slingshot phosphatase Ssh1, Large tumor suppressor Lats1, a Ser/Thr kinase, interaction with unbound bone morphogenetic protein receptor BMPR2, nischarin, LIMK1-short splice variant, and ubiquitin ligases RNF6 and Parkin.
5.9.3.2 LIMK2
Paralog LIMK2 is activated by Rho GTPase effector kinases RoCK1, PAK1, and myotonic dystrophy kinase-related CDC42-binding kinase MRCKα once they are actuated by CDC42, Rac, and Rho [482]. Activated LIMK2 phosphorylates (inactivates) the 3 members of the cofilin family, like its paralog LIMK1, hence stabilizing accumulated actin filaments (single known LIMK2 function).
Whereas LIMK1 colocalizes with actin stress fibers and focal adhesions, LIMK2 is found in punctae that resemble endosomes [482]. Isoform LIMK2, but not LIMK1, is inhibited by protein partitioning defective Par3 to impede cofilin phosphorylation and tight junction assembly. Like LIMK1, heat shock protein HSP90 provokes LIMK2 dimerization.
Nuclear import of LIMK2 is suppressed after phosphorylation by PKCδ (Ser283 and Thr494). Transforming growth factor-β1 induces LIMK2 phosphorylation by RoCK kinase. Slingshot phosphatase Ssh1 dephosphorylates (inactivates) LIMK2.
5.9.4 Testis-Specific Kinase Subfamily (LISK [LIMK–TESK] Family)
Testicular protein kinases (TesK) form a subfamily that phosphorylate (deactivate) cofilin, like members of the LIMK subfamily. Kinases LIMK1 and LIMK2 are ubiquitous, whereas TesK family members are produced most abundantly in the testis.
The LIMK kinases are phosphorylated (activated; LIMK1: Thr508; LIMK2: Thr505) by distinct Rho GTPase pathways [483]: (1) CDC42 and Rac act via P21-activated kinases PAK1 and PAK4; (2) CDC42 via myotonic dystrophy-related CDC42-binding kinase; and (3) RhoA via RoCK kinase. On the other hand, TesK activation depends on integrin engagement upon cell attachment. It occurs independently of PAK1 and RoCK activation.
5.9.4.1 Testis-Specific Kinase-1
Testis-specific kinase-1 (TesK1) is a protein Ser/Thr kinase that possesses an N-terminal protein kinase region and a C-terminal proline-rich domain. Its kinase domain is closely related to those of the LIM (Lin11/Isl1/Mec3) motif-containing protein kinases. Therefore, kinases of the LIMK and TESK subfamilies constitute the LIMK/TESK family of protein Ser/Thr kinases. It is encoded by the TESK1 gene. It can phosphorylate myelin and histone.
5.9.4.2 Testis-Specific Kinase-2
Testis-specific kinase-2 (TesK2) is a protein Ser/Thr kinase that contains an N-terminal kinase domain structurally similar to that of TesK1 and LIMK kinases. Its encoded by the TESK2 gene that is predominantly expressed in the testis and prostate.
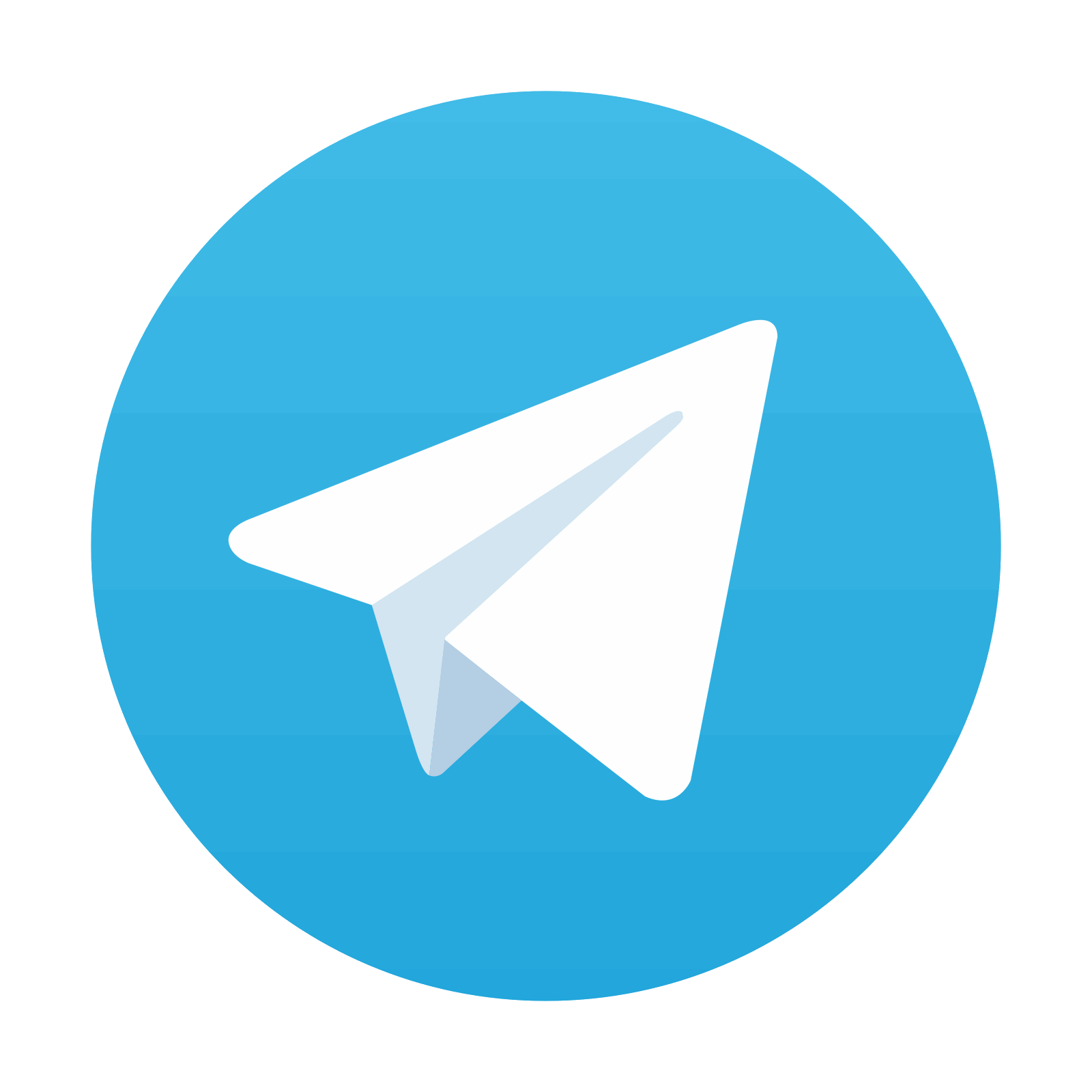
Stay updated, free articles. Join our Telegram channel

Full access? Get Clinical Tree
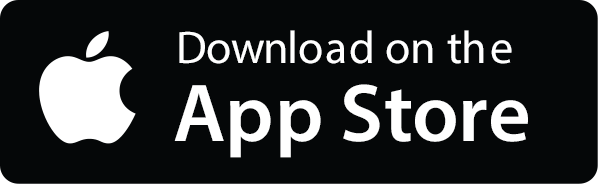
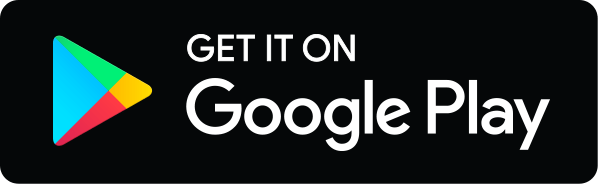
