Background
The aim of this study was to test the hypothesis that prognosis in patients with cardiac amyloidosis is closely coupled with amyloid burden in all four cardiac chambers. The goal was to evaluate longitudinal strain (LS) in each cardiac chamber and to determine whether LS in specific cardiac chambers is preferentially associated with prognosis over conventional two-dimensional echocardiographic parameters in patients with cardiac amyloidosis.
Methods
Patients with two phenotypes of left ventricular (LV) hypertrophy (cardiac amyloidosis in 55 patients and nonobstructive hypertrophic cardiomyopathy in 40 patients) and 55 healthy subjects were retrospectively enrolled for the simultaneous assessment of LS of all four cardiac chambers in the apical four-chamber view. Patients with cardiac amyloidosis were followed up to record major adverse cardiovascular events, including cardiac death, heart transplantation, nonfatal myocardial infarction, ventricular tachyarrhythmia, and exacerbation of heart failure requiring hospitalization.
Results
LS in each chamber was significantly depressed in patients with both LV hypertrophy phenotypes compared with healthy subjects. Right atrial LS was significantly lower in patients with cardiac amyloidosis than those with nonobstructive hypertrophic cardiomyopathy after adjusting for LV ejection fraction and LV mass index. During a median follow-up period of 10 months, major adverse cardiovascular events developed in 22 patients with cardiac amyloidosis. Four-chamber LS were significantly associated with major adverse cardiovascular events, with incremental value over traditional echocardiographic parameters.
Conclusions
Cardiac amyloidosis involves all cardiac chambers, and thus, chamber-specific strain analysis may be useful to assess the total cumulative burden of cardiac dysfunction.
Highlights
- •
Simultaneous assessment of LS in all four cardiac chambers was performed in patients with cardiac amyloidosis, those with nonobstructive HCM, and control subjects.
- •
LS in each chamber was significantly depressed in patients with both LVH phenotypes compared with control subjects.
- •
Four-chamber LS was significantly associated with MACEs in patients with cardiac amyloidosis.
- •
Chamber-specific strain analysis may be useful to assess the total cumulative burden of cardiac dysfunction in patients with cardiac amyloidosis.
Amyloidosis is characterized by extracellular deposition of amyloid fibrils in multiple systemic organs. Because cardiac amyloidosis is a major cause of death, several echocardiographic parameters have been demonstrated to be significant predictors of poor prognosis. Although clinical outcome is generally related to the type of amyloidosis, the prognosis of each patient remains variable. Therefore, the identification of patients at increased risk for cardiac events remains challenging.
Strain and strain rate are useful parameters for quantifying cardiac chamber mechanics, and their prognostic value has been demonstrated in several cardiovascular diseases. Previous studies have reported the utility of tissue Doppler or speckle-tracking analysis, revealing that impaired global left ventricular (LV) longitudinal strain (LS) and basal LS were more closely associated with worse clinical outcomes compared with conventional echocardiographic parameters in patients with cardiac amyloidosis. Myocardial infiltration in cardiac amyloidosis involves not only the left ventricle but also other cardiac chambers. The severity and extent of cardiac involvement are associated with worse outcomes. We hypothesized that prognosis in patients with cardiac amyloidosis is closely coupled to the amount of amyloid burden in all four cardiac chambers, and thus, assessment of LS in each chamber with two-dimensional (2D) speckle-tracking analysis has the potential to identify high-risk patients for future cardiac events. Recently, a novel vendor-independent 2D speckle-tracking software package (Research; Epsilon Imaging, Ann Arbor, MI) has enabled simultaneous measurements of LS in all cardiac chambers with the use of the apical four-chamber view.
The purposes of this study were (1) to compare chamber-specific LS in patients with cardiac amyloidosis and another phenotype of LV hypertrophy (LVH), hypertrophic cardiomyopathy (HCM), and control subjects; (2) to determine if simultaneous assessment of four-chamber LS is associated with cardiac events in patients with cardiac amyloidosis; and (3) to determine whether chamber-specific LS adds incrementally to risk stratification compared with clinical and conventional echocardiographic parameters.
Methods
Study Subjects
We retrospectively searched for patients with high probabilities of cardiac amyloidosis who were referred to the echocardiography laboratories of four university hospitals (the University of Occupational and Environmental Health, the University of Chicago, the University of Tsukuba, and Gunma University). The diagnosis of cardiac amyloidosis was confirmed either by demonstrating amyloid deposits on endomyocardial biopsy or, in patients with the typical echocardiographic features of cardiac amyloidosis (mean LV thickness ≥ 12 mm), by demonstrating amyloid depositions on histologic examination of a biopsy from other tissues (e.g., abdominal fat pad, rectum). The type of amyloidosis was then assessed by immunohistochemistry. Among 106 patients screened, 51 patients were excluded because biopsy was not performed ( n = 19), biopsy was performed but there was no amyloid deposition or histology could not determine the type of amyloidosis ( n = 21), echocardiographic image quality was poor ( n = 2), or no follow-up information was available ( n = 9), leaving 55 patients in the final study group. We also enrolled 40 age- and gender-matched patients with nonobstructive HCM and 55 healthy subjects. Diagnosis of HCM was defined as the presence of increased LV wall thickening (≥15 mm) in the LV wall in the absence of any identifiable cause. Healthy subjects were selected from the database, which had been used in a previous publication. The study, for which the requirement to obtain written informed consent was waived, adhered to the Declaration of Helsinki and was approved by the human research and ethics committee of each hospital.
Echocardiography
All patients underwent standard echocardiographic evaluation following the guidelines of the American Society of Echocardiography using commercially available ultrasound systems (iE33 [Philips Medical System, Andover, MA] or Vivid 7 [GE Vingmed Ultrasound AS, Horten, Norway]). The Digital Imaging and Communications in Medicine–formatted files were then exported to a personal computer, and data were analyzed using vendor-independent 2D speckle-tracking software (EchoInsight; Epsilon Imaging) by an experienced observer. For the simultaneous measurement of LS in all chambers throughout the same cardiac cycle, an apical four-chamber view was recorded. Care was taken to include all cardiac chambers in the data acquisition. The endocardial and epicardial borders of both the left and right ventricles were manually traced in the end-diastolic frame, and the region of interest adjusted to track all myocardial segments adequately. Subsequently, the software (EchoInsight for Research, Epsilon Imaging) divided the right ventricular (RV) and LV myocardium into six regions and performed speckle-tracking analysis, resulting in the generation of averaged LS curves of the LV and RV segments. For the calculation of left atrial (LA) and right atrial (RA) LS, the same software was used after tracing both the endocardial and epicardial borders of the atrial wall in the end-systolic frame ( Supplemental Figure 1 ). The peak LS of each chamber was obtained from the individual average strain curve. The interventricular septum was included in the LS measurements for both the left and right ventricles. The interatrial septum was likewise included in the LS measurements for both the left and right atria. To assess LV volumes and ejection fraction, the region of interest was manually traced in the three apical views at the end-diastolic frame using a different software (EchoInsight for Cardio Oncology; Epsilon Imaging). The software automatically performed speckle-tracking analysis on a frame-by-frame basis and generated time-domain regional LS curves. After completion of speckle-tracking in all three apical views, the software provided LV global LS. The software also provided LV end-diastolic and end-systolic volumes and LV ejection fraction using the biplane Simpson formula. Although both LV LS and LV global LS were obtained, we used only LV LS in the analysis. Tricuspid annular plane systolic excursion and RV fractional area change were obtained by a RV-focused view (EchoInsight for RV; Epsilon Imaging). Because the negative values of LV LS and RV LS can cause confusion, the absolute values of LV LS and RV LS are instead reported in the text, figures, and tables. Thus, higher LV and RV strain values represent improved LV and RV function.
LV mass was calculated from measurements of LV wall thickness and chamber dimension, and LA volumes were calculated using the biplane area-length method. Both LV and LA volumes and LV mass were subsequently indexed to body surface area. The early filling (E-wave) and peak late diastolic (A-wave) velocities were obtained from the transmitral flow. The peak systolic (s′), early diastolic (e′), and late diastolic (a′) mitral annular velocities were recorded at the septal corner of the mitral annulus. The ratio of early mitral diastolic inflow velocity to early diastolic mitral annular velocity (E/e′) was also calculated. Diastolic dysfunction was categorized into four groups according to previous reports (normal, impaired relaxation, pseudonormal, or restrictive).
Observer Variability
The reproducibility of strain measurements (LV, RV, LA, and RA LS) was assessed in 15 randomly selected patients. Intra- and interobserver variabilities were evaluated after the same observer and another reader repeated the analysis 3 months later and are reported as percentage variability, defined as the absolute difference in percentage of the mean of repeated measurements and intraclass correlation coefficient.
Follow-Up
In patients with cardiac amyloidosis, follow-up information was obtained by clinical visit or telephone interview. The primary end point was a composite of major adverse cardiovascular events (MACEs), including cardiac death, cardiac transplantation, nonfatal myocardial infarction, ventricular tachycardia or fibrillation, and hospitalization for worsening heart failure. The secondary end point was a composite of cardiac death and heart transplantation.
Statistical Analysis
All continuous variables are expressed as mean ± SD or as median and interquartile range (IQR) according to data distribution. Normality was evaluated using the Shapiro-Wilk W test. Intergroup differences were assessed using χ 2 , one-way analysis-of-variance, or Kruskal-Wallis tests. Then, multiple comparisons using the Tukey test or Bonferroni corrections were performed. In the first cross-sectional study, we compared echocardiographic parameters among patients with amyloidosis, those with HCM, and healthy subjects. We used a regression model to adjust for the effects of LV ejection fraction and LV mass index on the differences in baseline characteristics between patients with cardiac amyloidosis and those with nonobstructive HCM. Next, the association between four-chamber LS and worse outcome in patients with cardiac amyloidosis was assessed. MACE-free survival was then evaluated using Kaplan-Meier analysis, whereas the associations between echocardiographic variables and worse outcome were assessed using a Cox proportional-hazards model. For patients with multiple events, the first MACE was considered an event. Because the number of events was limited, the robustness of strain variables was tested using progressive models, as previously described. The proportional-hazards assumption, assessed by the goodness-of-fit approach for each independent variable, was not violated. The incremental value was determined by comparing χ 2 values for each model. A two-sided P value < .05 was considered to indicate statistical significance. All data were statistically analyzed using SPSS version 23.0 (SPSS, Chicago, IL) and JMP version 11.0 (SAS Institute, Cary, NC).
Results
Baseline Clinical Characteristics
Table 1 shows the baseline clinical characteristics of the study patients. There were no significant differences in age and gender among the three groups. Amyloid light chain (AL) was the dominant type of amyloidosis, followed by transthyretin-related (ATTR) and amyloid A protein amyloidosis.
Variable | Normal ( n = 55) | HCM ( n = 40) | Amyloidosis ( n = 55) | P |
---|---|---|---|---|
Clinical characteristics | ||||
Age (y) | 64 (58–69) | 67 (59–76) | 66 (56–77) | .477 |
Women | 38% | 38% | 38% | .997 |
Body surface area (m 2 ) | 1.72 ± 0.23 | 1.55 ± 0.22 ∗ | 1.70 ± 0.25 † | .002 ‡ |
Systolic blood pressure (mm Hg) | 131 ± 15 | 136 ± 25 | 116 ± 22 ∗ † | <.001 ‡ |
Diastolic blood pressure (mm Hg) | 73 ± 9 | 76 ± 13 | 68 ± 13 † | .005 ‡ |
Heart rate (beats/min) | 63 (59–70) | 67 (56–71) | 80 (66–93) ∗ † | <.001 § |
Type of amyloidosis | ||||
AL/AA/ATTR | — | — | 69%/4%/27% | — |
LV volume and function | ||||
Septal wall thickness, mm | 8 (7–9) | 16 (14–17) ∗ | 14 (13–16) ∗ | <.001 § |
Posterior wall thickness, mm | 8 (7–9) | 10 (9–12) ∗ | 14 (13–16) ∗ † | <.001 § |
LV mass index (g/m 2 ) | 69 ± 21 | 141 ± 35 ∗ | 137 ± 32 ∗ | <.001 ‡ |
LV end-diastolic diameter (mm) | 45 ± 7 | 42 ± 5 | 41 ± 5 ∗ | <.001 ‡ |
LV end-systolic diameter (mm) | 30 ± 7 | 27 ± 4 | 30 ± 7 | .037 ‡ |
LV end-diastolic volume index (mL/m 2 ) | 52 (45–63) | 51 (42–64) | 59 (52–71) | .038 § |
LV end-systolic volume index (mL/m 2 ) | 21 (17–26) | 22 (16–28) | 29 (24–39) ∗ † | <.001 § |
LV stroke volume index (mL/m 2 ) | 31 (28–38) | 29 (24–35) | 28 (20–31) ∗ | .006 § |
LV ejection fraction (%) | 60 (57–65) | 58 (52–62) | 48 (38–55) ∗ † | <.001 § |
Diastolic dysfunction | ||||
E wave (cm/sec) | 74 (58–87) | 58 (45–70) ∗ | 83 (69–105) ∗ † | <.001 § |
A wave (cm/sec) | 69 (58–94) | 71 (50–86) | 47 (25–79) ∗ † | .001 § |
E/A ratio | 0.97 (0.79–1.21) | 0.76 (0.57–1.03) | 1.69 (0.82–2.93) ∗ † | <.001 § |
e′ velocity (cm/sec) | 7.5 (6.2–8.5) | 3.5 (3.0–4.6) ∗ | 4.1 (2.7–5.2) ∗ | <.001 § |
s′ velocity (cm/sec) | 7.8 (6.7–8.7) | 5.7 (4.4–6.8) ∗ | 5.0 (3.3–6.1) ∗ | <.001 § |
E/e′ ratio | 10.0 (7.9–12.7) | 14.5 (10.2–20.0) ∗ | 22.8 (17.4–28.1) ∗ † | <.001 § |
Maximal LAVI (mL/m 2 ) | 28 (24–32) | 43 (34–62) ∗ | 38 (31–48) ∗ | <.001 § |
PASP (mm Hg) | 28 ± 7 | 34 ± 7 ∗ | 36 ± 11 ∗ | <.001 ‡ |
Four-chamber strain (%) | ||||
LV LS | 16.6 (15.3–18.8) | 12.3 (8.3–14.5) ∗ | 9.0 (6.4–12.5) ∗ | <.001 § |
RV LS | 17.1 (14.5–20.2) | 12.0 (7.9–16.6) ∗ | 10.2 (6.9–14.0) ∗ | <.001 § |
LA LS | 30.1 (23.5–37.7) | 15.4 (11.3–20.7) ∗ | 8.1 (4.7–14.7) ∗ † | <.001 § |
RA LS | 35.9 (25.3–44.0) | 21.1 (16.0–30.4) ∗ | 12.6 (6.1–20.8) ∗ † | <.001 § |
∗ P < .05 in pairwise comparison with control subjects based on Tukey or Bonferroni test.
† P < .05 in pairwise comparison with patients with HCM based on Tukey or Bonferroni test.
‡ P values are from overall omnibus tests using one-way analysis of variance.
§ P values are from overall omnibus tests using Kruskal-Wallis test.
Echocardiographic Parameters
Table 1 also depicts echocardiographic findings among the three groups. Compared with healthy subjects, LV wall thickness and LV mass index were significantly increased in patients with the two LVH phenotypes. LV end-systolic volume index was significantly larger but LV ejection fraction was significantly lower in patients with cardiac amyloidosis compared with those with HCM. More depressed diastolic dysfunction, characterized by increased E-wave velocity, decreased A-wave velocity, and higher E/A and E/e′ ratios, was also observed in patients with cardiac amyloidosis compared with healthy control subjects and patients with HCM. Patients with cardiac amyloidosis had severe diastolic dysfunction: normal in 0%, impaired relaxation in 2%, pseudonormal in 47%, restrictive in 49%, and indeterminate in 2%. However, no significant difference in maximum LA volume index was noted between patients with cardiac amyloidosis and those with HCM.
Regarding four-chamber LS, LS in each chamber was severely depressed in patients with HCM and those with cardiac amyloidosis compared with healthy subjects ( Figure 1 ). LA LS and RA LS were significantly depressed in patients with cardiac amyloidosis compared with those with HCM, whereas LV LS and RV LS were not different between the two groups. RA LS remained significantly lower in patients with amyloidosis than those with HCM after adjusting for LV ejection fraction and LV mass index ( P = .013), while the difference in LA LS was not statistically significant ( P = .058).
The associations between type of amyloidosis and four-chamber LS were also assessed. Because the number of patients with amyloid A protein amyloidosis was quite small, we compared LS values between those with AL amyloidosis and those with ATTR amyloidosis. There were no significant differences in LV ejection fraction (AL vs ATTR, 48% [IQR, 39%–54%] vs 43% [IQR, 34%–55%], P = .503) and RA LS (AL vs ATTR, 15.7% [IQR, 7.4%–23.9%] vs 9.6% [IQR, 5.2%–13.6%], P = .052) between the two groups. However, LV mass index was significantly larger (131 ± 31 vs 154 ± 31 g/m 2 , P = .022), and LV LS (9.9% [IQR, 6.4%–13.1%] vs 7.5% [IQR, 6.1%–9.2%], P = .037), RV LS (10.4% [IQR, 7.5%–15.5%] vs 7.7% [IQR, 5.6%–10.7%], P = .045), and LA LS (10.4% [IQR, 5.2%–16.2%] vs 5.5% [IQR, 2.9%–7.5%], P = .003) were significantly depressed in patients with ATTR amyloidosis.
Clinical Outcomes and Four-Chamber Strain
During a median follow-up period of 10 months (IQR, 3–20 months), MACEs developed in 22 patients, including 15 cardiac deaths, one cardiac transplantation, one nonfatal myocardial infarction, one ventricular fibrillation, and four hospitalizations because of heart failure. Type of amyloidosis was not significantly associated with MACEs ( P = .279). Table 2 depicts the results of univariate Cox proportional-hazards analysis to assess the association of clinical and echocardiographic parameters with MACEs in patients with cardiac amyloidosis. Tricuspid annular plane systolic excursion, e′, s′, E/A ratio, E/e′ ratio, and maximal LA volume index were significantly associated with MACEs. Among four-chamber LS, all four-chamber LS values were related to increased risk for events. Figure 2 shows Kaplan-Meier survival analyses for the two groups stratified by median values of LV LS, LA LS, RV LS, and RA LS. All of these parameters could be stratified in high-risk patients who developed MACEs. Figure 3 depicts Kaplan-Meier survival analysis for the four groups according to cutoff values of LV LS and RA LS. MACE-free survival in patients with the combination of LV LS > 9% and RA LS > 12.6% was significantly better than those with LV LS ≤ 9% and RA LS ≤ 12.6% ( P < .001). Interestingly, MACE-free survival was similarly worse in patients if either LV LS or RA LS was below the cutoff values.
Variable | Hazard ratio (95% CI) | P |
---|---|---|
Age, per 1-y increase | 1.05 (1.00–1.10) | .035 |
Female gender | 0.52 (0.20–1.35) | .179 |
LV end-diastolic volume index, per 1 mL/m 2 increase | 0.99 (0.97–1.02) | .634 |
LV end-systolic volume index, per 1 mL/m 2 increase | 1.00 (0.98–1.03) | .889 |
LV stroke volume index, per 1 mL/m 2 increase | 0.96 (0.91–1.02) | .196 |
LV ejection fraction, per 1% increase | 0.99 (0.95–1.02) | .424 |
LV mass index, per 1 g/m 2 increase | 1.01 (0.99–1.02) | .095 |
E wave, per 1 cm/sec increase | 0.99 (0.97–1.01) | .412 |
A wave, per 1 cm/sec increase | 0.99 (0.97–1.01) | .173 |
e′ velocity, per 1 cm/sec increase | 0.68 (0.48–0.96) | .027 |
s′ velocity, per 1 cm/sec increase | 0.61 (0.44–0.83) | .002 |
E/A ratio, per 1-unit increase | 1.06 (1.01–1.11) | .027 |
E/e′ ratio, per 1-unit increase | 1.03 (1.00–1.05) | .023 |
Maximal LAVI, per 1 mL/m 2 increase | 1.04 (1.01–1.07) | .006 |
PASP, per 1 mm Hg increase | 1.00 (0.96–1.04) | .984 |
TAPSE, per 1-cm increase | 0.12 (0.03–0.50) | .003 |
RV fractional area change, per 1% increase | 0.97 (0.92–1.02) | .242 |
Restrictive filling | 0.91 (0.38–2.14) | .823 |
LV LS, per 1% increase | 0.75 (0.63–0.89) | .001 |
RV LS, per 1% increase | 0.89 (0.81–0.98) | .020 |
LA LS, per 1% increase | 0.88 (0.81–0.97) | .007 |
RA LS, per 1% increase | 0.89 (0.84–0.95) | <.001 |
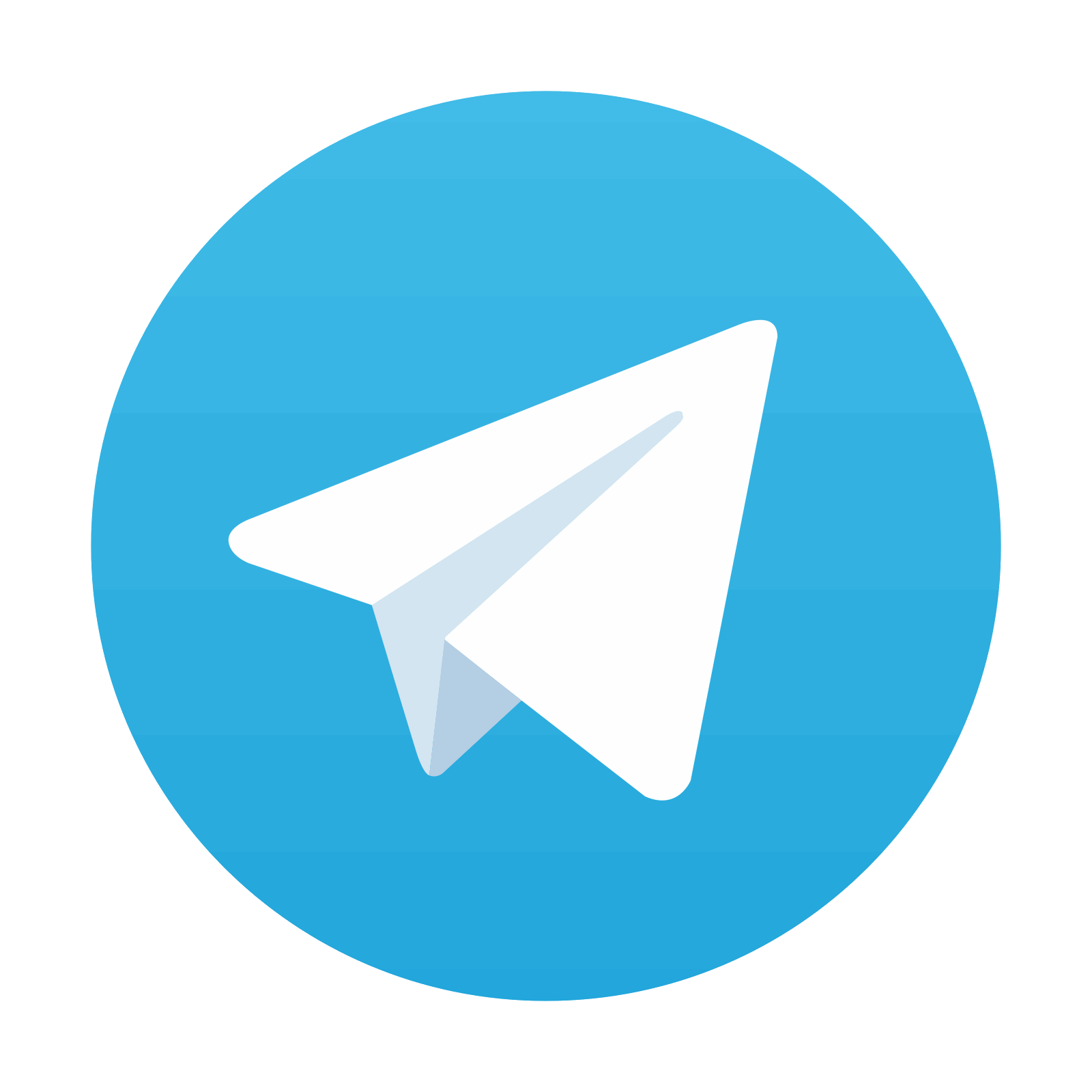
Stay updated, free articles. Join our Telegram channel

Full access? Get Clinical Tree
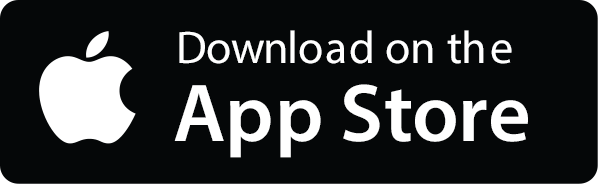
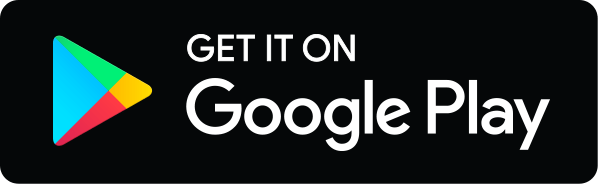
