Fig. 7.1
Acute chest pain with normal ECG in a 55 year old female with hypertension and hypercholesterolemia as coronary risk factors. The patient is rapidly discharged after computed tomography coronary angiography shows absence of coronary atherosclerosis in the early triage
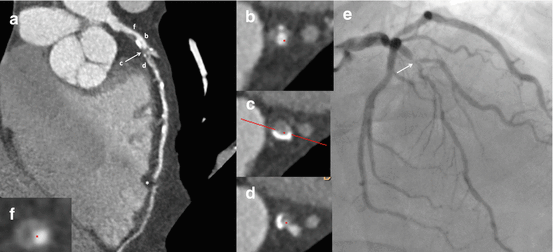
Fig. 7.2
Atypical acute chest pain in a 58 year old male with hypercholesterolemia and smoking as coronary risk factors, and non-specific repolarization abnormalities. Computed tomography coronary angiography is performed as part of the early triage (curved multiplanar reconstructions), showing a critical lesion at the mid left anterior descending (LAD) artery, with compromise of a large diagonal branch (panel a, arrow). The asterisk refers to a motion artifact attributed to prospective ECG-gating (effective dose 4.1 mSv in a patient with a body mass index of 29 kg/m2). An orthogonal view of the lesion depicts the subtotal occlusion, with mild eccentric calcification (c), whereas panels (b, d) show the immediately proximal and distal reference sites with extensive disease. Invasive angiography confirms the findings (panel e). Of note, a large eccentric non-calcified plaque with positive remodeling, low attenuation, and napkin-ring sign (panel f); all characteristics of plaque vulnerability, is observed at the proximal LAD
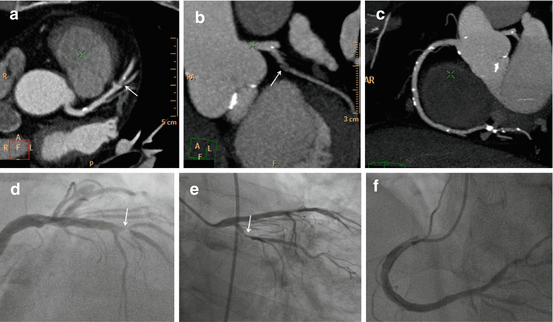
Fig. 7.3
Sixty eight year old male with hypertension and hypercholesterolemia as coronary risk factors. Typical rest angina with normal ECG and normal first set of cardiac enzyme levels. Computed tomography coronary angiography is performed as part of the early triage (maximum intensity projections area shown), demonstrating a critical lesion at the mid left anterior descending artery (panel a, arrow), and a severe lesion at the proximal left circumflex (panel b, arrow); whereas mild calcifications are seen at the right coronary artery (panel c). Invasive angiography confirmed the findings (panels d–f)
In the past decade, the safety, promptness, and high negative predictive value of CTCA has driven its incorporation as an alternative for the triage of patients with acute chest pain at low to intermediate risk of CAD. Notwithstanding, several controversies persist, particularly concerns regarding whether this technique might lead to an increase in downstream costs and in revascularization rates.
Computed Tomography Coronary Angiography (CTCA)
CTCA is more closely related to intravascular ultrasound than to ICA [16]. Based on that principle, CTCA has significantly contributed to visualize atherosclerosis in the earlier phases and has therefore contributed to a better understanding of the blind gap between the onset of coronary atherosclerosis and the development of obstructive CAD [10–15]. This feature of CTCA has potential important clinical implications since most acute thrombotic coronary events arise from angiographically non significant lesions [17–19]. As a matter of fact, until recently, only ex vivo and catheter-based diagnostic tools enabled the assessment of the anatomic substrate of presumed high risk plaques [20–25].
During the past decade, CTCA has earned a more active role in different diagnostic algorithms of patients suspected of CAD. Several studies have explored the role of CTCA for the detection of obstructive de novo lesions [26–28]. These studies have demonstrated that CTCA has a high diagnostic accuracy to detect obstructive CAD in diverse populations, although patients at intermediate risk appear as the best scenario. A number of multicenter studies have confirmed these earlier findings, with sensitivities ranging between 85 and 95 %, specificities between 83 and 90 %, and similar diagnostic accuracy compared to ICA regarding the ability to predict short term revascularization. Despite slight differences in study design and populations, there is robust evidence that positions CTCA as the non invasive diagnostic tool with the highest negative predictive value (Fig. 7.1) [26–28].
In the past decade, great technical advances in the field of CTCA have been witnessed, that have a major effect in effective dose radiation (EDR), image quality, scan duration, physiological assessment, iodine volume load, and myocardial perfusion imaging. Numerous tools have been successfully elaborated in order to reduce radiation dose associated with CTCA, such as iterative reconstruction, tube modulation, prospective ECG-gating, and high-pitch spiral acquisitions [29–31]. Using a combination of these techniques enable high quality acquisitions with radiation dose levels lower than 3 mSv for CTCA with prospective triggering and below 1 mSv for high-pitch helical mode studies using dual source scanners [29, 30, 32]. For instance, high pitch scans can be performed in 258 ms with a mean EDR of barely 0.87 msV [31]. Nevertheless, the Prospective Multicenter Study on Radiation Dose Estimates of Cardiac CT Angiography I and II (PROTECTION I and II) reported a wide range of effective radiation doses according to the acquisition technique. Therefore, the use of one or more of the aforementioned dose reduction techniques should be encouraged [33, 34].It should be noted that prospective ECG triggering should not be considered as a first choice in obese patients, and if heart rate is high (≥70 bpm) or irregular.
Despite the considerable technical developments of the past decades, the high sensitivity and negative predictive value of CTCA has remained consistently over 90 % and relatively unaffected since the 16-row CTCA generation. On the contrary, the specificity and the positive value has significantly improved with the incorporation of newer generations of scanners [35]. From a clinical standpoint, this has been translated into a reduced number of normal downstream invasive diagnostic procedures (~14 % to ~4 %) [26, 27].
Role of CTCA in Patients with Acute Chest Pain
Recently, CTCA has emerged as an alternative for patient triage with acute chest pain and low to intermediate probability of CAD. Three large randomized multicenter studies (CT-STAT, ACRIN-PA, and ROMICAT II) have shown that CTCA is a safe strategy that allows a significant reduction in diagnostic time, thereby leading to a larger percentage of patients with discharge from the emergency department (ED). Importantly, this benefit derived a significant reduction in costs [36–38].
The CT-STAT trial randomized 749 patients with low-risk acute chest pain to CTCA or standard of care (SOC). Despite a similar diagnostic performance between the two strategies, patients assigned to CTCA had a significant reduction in diagnostic time (54 %; 2.9 h vs. 6.2 h; p < 0.001) as well as in total cost (38 %; USD $2137 vs. USD $3458, p < 0.001) [36]. The ACRIN-PA trial randomized 1370 patients with low to intermediate risk to CTCA or SOC. In this study, CTCA had significantly higher rates of discharge from the ED (50 % vs. 23 %, p < 0.001), and a significantly lower number of unnecessary (false positive) invasive angiograms (p < 0.05). Moreover, it is worth mentioning that of the 640 patients with a negative CTCA, none had an adverse event at 30 days follow-up [37]. Finally, the ROMICAT II trial randomized 1000 patients with acute chest pain and no ischemic ECG changes to CTCA or SOC. Patients assigned to CTCA showed a significant reduction in diagnostic time (5.8 h vs. 21.0 h; p < 0.001), and a significantly higher rates of discharge from the ED (47 % vs. 12 %, p < 0.001). In addition, no differences were observed regarding costs, even though it was pre-specified in the protocol that diagnostic tools within the control group were used at the treating physician’s discretion [38]. Hulten et al. recently published a meta-analysis of studies that explored the role of CTCA for the triage of patients with acute chest pain and concluded that CTCA is a safe strategy, improving patient management in the ED, reducing costs and hospital admission rates [39]. In addition, patients with acute chest pain with negative or mild CT findings can be safely and rapidly discharged without further testing, with excellent 30 day event-free survival [40]. The aforementioned evidence has lead to the integration of CTCA in a number of guidelines of clinical practice for acute chest pain management [41–44].
The potential role of cardiac CT in the triage of acute chest pain is not only related to the ability of CTCA to identify obstructive CAD. During the past decade, major technological advances in the field of CT have enabled a comprehensive assessment of both CTCA and stress myocardial perfusion during a single procedure [45]. Several clinical studies have validated this application using different scanners, pharmacological agents, and acquisition protocols [46, 47]. A recent prospective study compared two strategies to evaluate myocardial perfusion by CT (CTP) for the assessment of acute chest pain in patients who were clinically referred to stress/rest SPECT and cardiac MRI: real-time adenosine stress CTP using second generation dual-source CT (group A), and adenosine-stress first-pass dual energy CTP (group B). Compared to stress cardiac magnetic resonance, real-time CTP (versus SPECT) had a 86 % sensitivity, 98 % specificity, 94 % positive predictive value, and 96 % negative predictive value for the detection of myocardial perfusion defects. Furthermore, dual energy CTP had 93 % sensitivity, 99 % specificity, 92 % positive predictive value, and 96 % negative predictive value for detecting perfusion defects [48].
The incremental value of dual-energy CTP over CTCA for the detection of obstructive CAD has recently been considered. Wang et al. reported that the combination of dual energy CTP and CTCA may improve diagnostic performance compared to CTA alone for the diagnosis of significant coronary stenosis [49]. Furthermore, Bettencourt et al. showed that an integrated protocol including CTCA and CTP has an excellent sensitivity and very good specificity for detecting obstructive CAD (assessed by invasive fractional flow reserve), and that CTP provides a significant incremental value over CTCA in patients with intermediate to high pre-test probability [50]. Finally, rest CTP simultaneously evaluated during routine CTCA might aid the identification of perfusion defects in the presence of acute coronary occlusion [51]. Indeed, in a recent study that included patients presenting with chest pain to the ED, the evaluation of rest myocardial CTP showed a high diagnostic performance as compared with SPECT-MPI, and improved the accuracy of CTCA mainly by reducing rates of false-positive findings [52]. It should be stressed that most patients with acute chest pain arrive at the CT scanner with an IV line in and heart rate control medications already administrated. Therefore, the exam is usually fast and straightforward. Patients with high or irregular heart rates, pregnant woman, and those with renal failure should avoid this strategy.
Stress Myocardial Perfusion Imaging for the Triage of Acute Chest Pain
Low-risk patients for ACS (negative or unremarkable ECG, and negative initial troponin levels) require confirmatory functional tests including exercise treadmill testing or cardiac imaging evaluation. Early exercise testing in patients who present with chest pain in the ED has been shown to provide limited prognostic information over established clinical scores [53]. Furthermore, exercise testing is precluded in patients with uninterpretable ECG and in patients unable to exercise [54]. Stress imaging such as myocardial perfusion scintigraphy or dobutamine stress echocardiography (DSE) have been positioned in the past two decades as valuable tools with improved prognostic value [55, 56]. Among low risk patients with normal or non-diagnostic ECG and negative initial cardiac enzymes, stress myocardial perfusion imaging (MPI) by means of single photon emission CT (SPECT) has been convincingly established as an effective strategy to safely discharge patients; with a very high negative predictive value to predict myocardial infarction (99 %) or future adverse cardiac events (97 %) [57]. Furthermore, patients with a normal stress-only SPECT have similar mortality rates than those with normal rest-stress studies. Accordingly, rest imaging can be avoided in patients with normal stress, resulting in a significant reduction in radiation exposure [58].
Rest myocardial perfusion imaging in these patients has shown to improve ED triage decision making, with a high negative predictive value that results in reducing unnecessary hospitalizations [59, 60]. Nevertheless, the specificity and positive predictive value of rest MPI is fairly low, because perfusion defects at rest cannot discriminate between acute or chronic ischemia, and can also be due to attenuation artifacts. Accordingly, the strength of rest MPI is mainly its very high negative predictive value, with a 30-day rate of adverse cardiac events <1 %.
Several studies have demonstrated that adding stress myocardial perfusion imaging to conventional SOC strategy (clinical evaluation, serial ECGs, and cardiac markers) for patients presenting with acute chest pain improves clinical decision making by significantly reducing the need for hospitalization, without an increment in adverse event rates at 30 days or 1 year [61].
However, there is evidence suggesting that clinical risk scores (GRACE and TIMI risk scores) but not stress imaging predict long-term cardiovascular mortality in patients with normal or non-diagnostic electrocardiogram after ruling out ACS [62].
It could be hypothesized that aside from the ischemic burden, the anatomic burden of CAD might play an important role in these patients. A recent subanalysis of the COURAGE (Clinical Outcomes Utilizing Revascularization and Aggressive Drug Evaluation) trial showed that when both anatomic burden and ischemic burden of disease at baseline are considered concomitantly, the anatomic burden and the left ventricular ejection fraction, but not the ischemic burden were independent predictors of death, myocardial infarction, or non-ST elevation myocardial infarction [63]. These results are in line with recently published data both from COURAGE indicating that the extent of site-defined ischemia did not predict events and did not modify treatment effectiveness [64]; and from the STICH (Comparison of Surgical and Medical Treatment of Congestive Heart Failure and Coronary artery Disease) trial, showing that in patients with severely reduced systolic function, inducible ischemia did not identify those with worse prognosis or those whom would have obtained greater benefit from revascularization over optimal medical treatment [65].
In the near future, these novel diagnostic methods might lead to identification of three different groups of patients, possibly with dissimilar prognoses: (1) patients with CAD (obstructive disease); (2) patients with coronary atherosclerosis but normal stress tests; and (3) patients without epicardial coronary atherosclerosis but with microvascular disease. Indeed, a recent analysis of the CONFIRM registry (COronary CT Angiography EvaluatioN For Clinical Outcomes: An InteRnational Multicenter Registry) validated previous data showing that the extent of plaque burden, regardless of stenosis severity, has an independent prognostic value [66].
Does CTCA Lead to an Increment in Downstream Costs and More Revascularizations?
As discussed above, the use of CTCA for the triage of patients with acute chest pain is safe, reduces the time to diagnosis and the admission rates, and does not lead to an increment in costs. Nevertheless, there is concern that CTCA might lead to an increment in downstream costs mainly related to an increase in the rates of ICA and revascularization [39]. These have been raised mainly by the aforementioned meta-analysis of Hulten et al., although several issues should be addressed in this regard. This meta-analysis included 3266 patients, 1869 undergoing CTCA and 1397 undergoing SOC. The authors concluded that the use of CTCA for the triage of low-to intermediate risk patients in the ED is safe and reduces the length of stay. However, they found a mild (2 %) but significant differences in the rates of invasive angiography and coronary revascularization [39]. None of the individual studies found significant differences in this regard. Furthermore, the pooled rate of ICA was 8.4 % versus 6.3 % [OR 1.36 (CI 95 % 1.03–1.80)], and of revascularization, 4.6 % versus 2.6 % [OR 1.81 (CI 95 % 1.20–2.72)] for CTCA and SOC, respectively.
The clinical significance of such small difference is uncertain. The diagnostic yield of coronary angiograms induced by CTCA (54 %) was higher than those induced by SOC (41 %). Accordingly, is the question that CTCA leads to increased rate of revascularizations, or does SOC lead to fewer revascularizations? Furthermore, are patients with obstructive disease discharged without a diagnosis?
It might be believed that CTCA could eventually lead to an increment in downstream costs driven by the higher rates of ICA and revascularization. Nevertheless, since more than 90 % of patients did not undergo ICA, the major source of cost is the diagnostic workup per se. Moreover, the decision to revascularize depends on the interventional cardiologist, not on the CTCA that leads to it. Finally, it is noteworthy that two of the studies included in the meta-analysis performed SPECT in all patients with stenoses >25 % or calcium score (Agatston) >100. This very conservative strategy has probably leaded to increased rates of ICA in the CTCA group [36, 67].
The CT-STAT trial provided a head-to-head comparison of CTCA versus stress-rest SPECT in patients with acute chest pain presenting at the ED, and thus deserves a comment. In this study, the rates of ICA during the index visit (CTCA 6.7 %, SPECT 6.2 %, p = 0.80) and the total revascularization rates (CTCA 3.6 %, SPECT 2.4 %, p = 0.34) were similar between groups. Furthermore, the cumulative event rates were comparable (CTCA 5.2 %, SPECT 3.7 %, p = 0.36) [36].Clinical outcome studies in this population are warranted, although they would require very large sample sizes given the low event rate.
Further data regarding downstream cost utilization arises from a recent large multicenter registry (CONFIRM) that included 155,207 CTCA patients at intermediate risk of CAD and demonstrated that patients without CAD and those with only mild disease had low rates of ICA (2.5 % vs. 8.3 %, respectively), and very low revascularization rates (0.3 % vs. 2.5 %, respectively), after a 2.3 year follow-up [68].
Most of the aforementioned favorable evidence of CTCA is related to its unsurpassed negative predictive value, positioning the technique as a fast, safe, and possibly cost effective means to exclude CAD as the cause of an ACS in the ED. Such negative predictive value provides an unparalleled long-term event-free safety window, with zero events in a large cohort of patients with normal coronary arteries after a mean 52-month follow-up [69].
A recent decision analytic model possibly summarizes the concept of this chapter that one method is not meant to exclude the other. In this study that included patients with acute chest pain at low risk of CAD, CTCA plus confirmatory SPECT for indeterminate results might be the most cost effective strategy compared to stress ECG, echocardiography, and SPECT. This combination also reduced hospitalization of patients with an initially false-positive CTA [70]. Furthermore, two recently published meta-analyses indicate that the utilization of CTCA is a cost- and time-effective strategy for evaluation of low and intermediate risk acute chest pain patients in ED and can be used for safe exclusion of acute coronary syndrome (ACS) [39, 71].
Nevertheless, it should be stressed that results from these trials cannot be directly applied to the real world. Only highly trained physicians should read CTCA scans and patients presenting to the ED during evening or weekends were excluded. An attempt to incorporate CTCA in patients with acute chest pain and TIMI risk score ≤2 in a large urban health care system yielded promising results, analogous to the findings from randomized studies. Cury et al. proposed to risk stratification based on the following groups of risk of ACS: 0, low (negative CTA findings); 1, mild (1–49 % stenosis); 2, moderate (50–69 % stenosis); or 3, severe (≥70 % stenosis). Patients with negative CTCA were discharged, those with mild disease were discharged but followed up at the outpatient clinic, those with moderate stenosis underwent further stress testing, and patients with severe stenosis underwent ICA. The rate of MACEs in patients with stenosis ≥70 % (8.3 %) was significantly higher than in patients with negative CTA findings (0 %) or those with mild stenosis (0.2 %). Moreover, a 51 % decreased length of stay from 28.8 to 14.0 h (p < 0.0001) was observed after implementation of the dedicated chest pain protocol [40].
< div class='tao-gold-member'>
Only gold members can continue reading. Log In or Register a > to continue
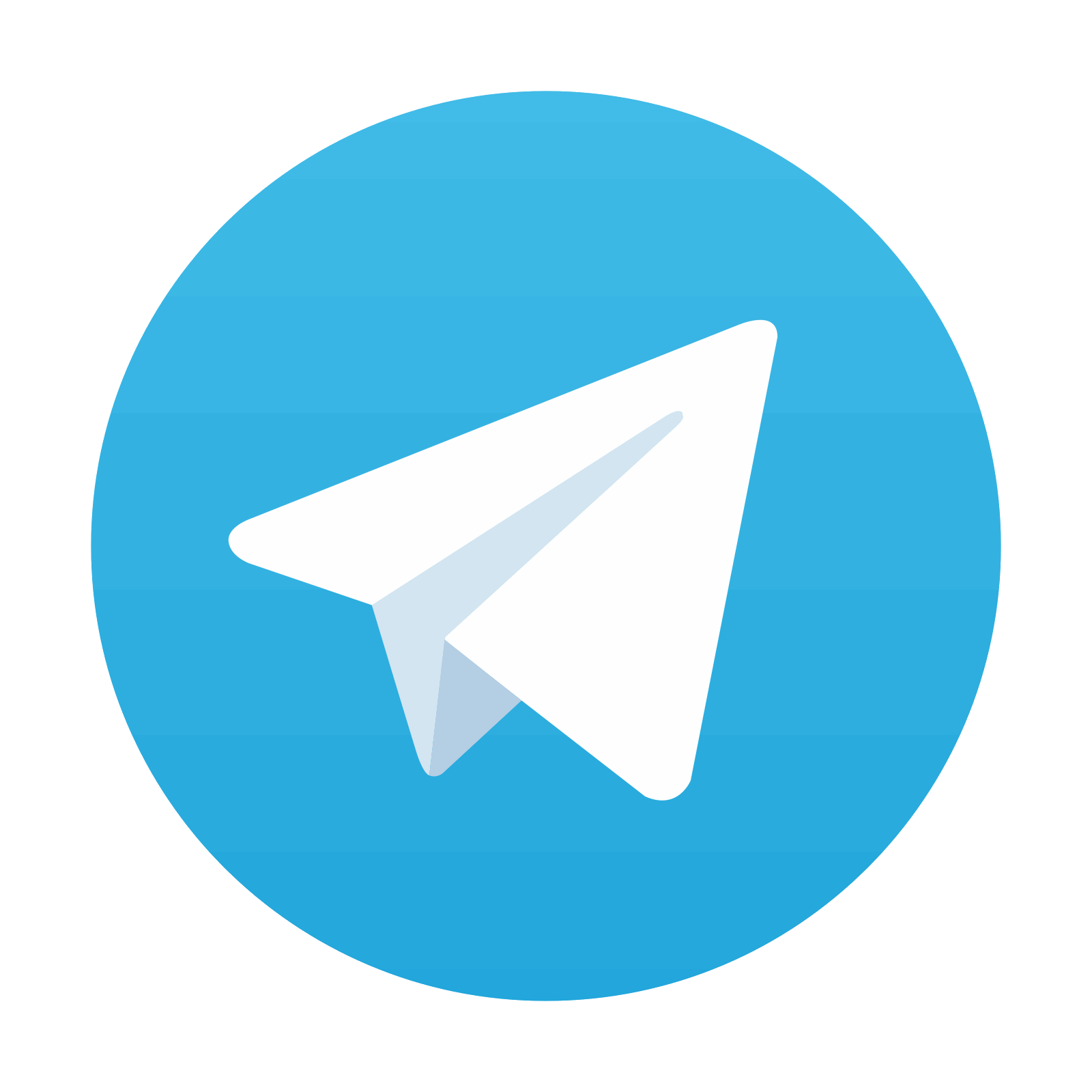
Stay updated, free articles. Join our Telegram channel

Full access? Get Clinical Tree
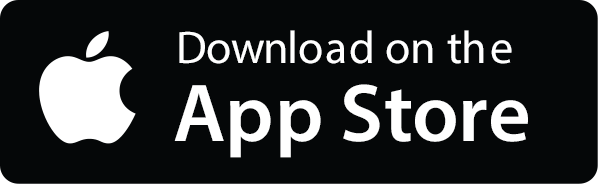
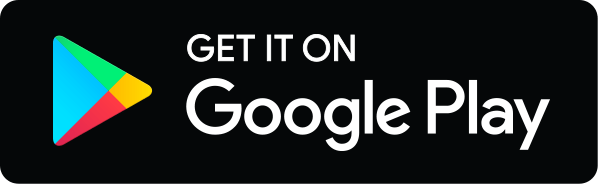