Cross Talk Signaling and Networking—Improving Outcomes in the Cardiometabolic Patient
Andrew Heyman, MD, MHSA
James B. LaValle, RPh, DHM, MS, CCN, ND (trad)
Cardiovascular disease (CVD) is the leading cause of death in the United States and many parts of the world. A recent press release by the American Heart Association states that nearly 50% of all people have some form of CVD.1 There are about 2200 deaths a day, averaging one death every 40 seconds, with almost one of every three deaths resulting from CVD.2
Considered a risk factor for CVD, the cardiometabolic syndrome is a metabolic disorder correlated in epidemiological studies with an increased risk of coronary heart disease, ischemic stroke, cardiovascular mortality, and total mortality. Cardiometabolic syndrome includes insulin resistance (prediabetes), impaired glucose tolerance, lipid imbalances, high blood pressure, and increased central adiposity. There is also evidence that the cardiometabolic syndrome is a risk factor for abnormalities in myocardial metabolism, cardiac dysfunction, and arrhythmias such as atrial fibrillation.3
Abdominal obesity is a key component of cardiometabolic (CM) risk, increasing the incidence of insulin resistance, vascular inflammation, dyslipidemia, and hypertension. Adipose tissue is recognized as an endocrine organ along with associated hormonal products. Adipose tissue appears to play a significant role in the regulation of fat and glucose metabolism throughout the body, and these mechanisms are clearly involved in the development of cardiovascular and CM disease.
The challenges of a strategy to treat the CM patient and to modify their risks are ever-evolving and should not be limited to drug therapy and surgery. On the surface, potentially modifiable risk factors include discontinuing tobacco use; improving physical inactivity; using drug therapy to control hypertension, blood glucose, and lipid imbalances; and changing some dietary habits. However, the CM patients generally have a cluster of symptoms that are also related to network biology problems that may not be so apparent initially, including involvement of insulin resistance and insulin signaling problems, hypothalamic-pituitary-adrenal (HPA) axis imbalances, and gut-heart axis (also encompasses the gut-immune-brain axis) involvement.
Understanding the effects and impact of systems biology networks, called TRIADs, on the cardiovascular system is essential in managing the CM patient. When these and other systems biology factors are taken into consideration, you can turn up the dial of response to treatments in these individuals, improving cardiovascular outcomes and improving the quality of life.
Background—What Are the TRIADs?
TRIADS are “categories” that group important organs/organ systems in your body together in “3s.” The reason for the grouping is to show the interrelationship of various network biology systems that have a significant impact on your overall metabolic health. Understanding and acknowledging the interconnectivity of all the metabolic networks is essential in treating the cardiovascular and CM patient. Although any construct of relationships described is somewhat artificial because all organ systems and networks potentially can affect all others leading to metabolic disruption, the networks described are commonly published and described in the medical literature. There are five TRIADs in the Network Biology approach to medicine:
TRIAD 1—consists of the adrenal, thyroid, and pancreas. TRIAD 1 encompasses HPA axis imbalances (stress and adrenals), sugar metabolism, and thyroid involvement in energy production and central network regulators of physiology. Imbalances manifest as fatigue, loss of vitality, and obesity.
TRIAD 2—consists of the Gut-brain-immune network, their interrelationship and effects on metabolism. TRIAD 2 encompasses command and control, digestion and absorption of nutrients, defense and repair, memory and cognitive function, sleep regulation, mood, memory, and inflammatory responses. Imbalances include weight gain and overeating, digestive complaints, inflammatory bowel diseases, allergies/food intolerances, sinus/colds/flu, immune and autoimmune imbalances, chronic inflammatory responses, sleep disorders, and mood and memory problems.
TRIAD 3—includes cardiovascular, pulmonary (cardiopulmonary), and neurovascular. TRIAD 3 includes the cardiopulmonary unit, autonomic and central nervous systems, and vascular tree. This triad reflects the relationship of cardiovascular health, cognition, mood, and stress. When TRIAD 3 organ systems are out of sync, the result is hypercholesterolemia, hypertension, increased resting heart rate, loss of vagal tone, increased fatigue, dyspnea or other pulmonary issues, and endothelial dysfunction. Of note, imbalances in TRIAD 1 and 2 in many cases will be the trigger to TRIAD 3 disturbances. In essence, chronic stress, elevated blood sugar, and low thyroid function lead to the eventual vasculopathy. The Network Biology approach helps map the complicated interconnected pathways of the brain-heart connection or in other words TRIAD 2 stacking with TRIAD 3. Again, looking at the metabolic network disturbances is essential.
TRIAD 4 includes the organs of detoxification—the liver and gallbladder, lymphatic system, and kidneys. Imbalances will lead to fatigue, fluid retention, reduced detoxification capacity, sluggish metabolism, intolerance to fats, edema, body odor and halitosis, and profuse sweating even in cold temperatures. TRIAD 4 organs of detoxification imbalances such as nonalcoholic fatty liver disease (NAFLD) can lead to cardiovascular consequences.4
TRIAD 5 is the metabolic network of the sex hormones—testosterone, estrogens, and progesterone. This TRIAD is potency and fertility. Imbalances can lead to impact on other networks, such as the cardiovascular and neurovascular. Weight gain, loss of muscle mass and strength, poor glucose regulation, inflammation, and disproportionate fat distribution are just a few of the issues when TRIAD 5 hormones are not in balance.
Cardiometabolic Disease—Underlying Factors
Obesity has been an epidemic for over a decade in both developed and developing nations and is the sixth most important risk factor contributing to the overall burden of disease worldwide.5 The average American consumes over 135 lb of sugar per person per year, whereas the average German ingests 75 lb per person per year.6 Central adiposity plays a critical role in the development of CM disease, and the extensive metabolic activity of visceral fat is increasingly recognized in the medical literature. Once thought of as merely a repository for excess calories, adipose tissue is now viewed as an endocrine gland capable of secreting numerous bioactive substances, including hormones, growth factors, and cytokines, that contribute directly to the development of heart disease and type 2 diabetes.7,8 Additionally, research links these same biochemical factors, and in particular insulin resistance, with cancer.9,10
CM risk is an expansion of underlying risk factors linked to metabolic syndrome (MetS). Insulin resistance and visceral obesity are the hallmarks of MetS, with hypertension and hypertriglyceridemia (typically with abnormalities in high-density lipoprotein (HDL) cholesterol) also serving as important co-contributors. CM risk is also amplified by other known cardiovascular markers such as high-sensitivity C-reactive protein (hs-CRP), Lpa and Lp-PLA2, oxidized low-density lipoprotein (LDL) and LDL particle size, fibrinogen, myeloperoxidase and markers of oxidative stress, including 8-hydroxyguanosine (8-OHdG), ApoB, F2 isoprostanes, superoxide dismutase (SOD), and methyl malonic acid. Although a unified model of CM disease remains incomplete, it is now clear that inflammation, hormonal derangements, stress, central obesity, environmental burden, nutritional deficiencies, and high oxidative stress underlie the progression toward insulin resistance, diabetes, and heart disease.
Prevalence of Cardiometabolic Disease
The term CM disease is relatively new, and accurate estimates on prevalence are not well defined in the literature. In contrast, the statistics on MetS, diabetes, and heart disease provide insight into the problem because they act as proxies for the underlying features of CM risk. It is estimated that 20% of the US population has MetS and 50% of adults in the United States have prediabetes (insulin resistance) or type 2 diabetes. The Centers for Disease Control and Prevention report that diabetes is the sixth leading cause of death in the United States. Additionally, heart disease continues to be the primary cause of morbidity and mortality in the United States. The 2006 estimates show that 80 million people in the United States had one or more forms of CVD.3
Cardiometabolic Risk Key Considerations
Visceral fat
Insulin resistance
Atherogenic dyslipidemia (triglycerides, ox-LDL, small dense LDL)
Hypertension
Glucose intolerance (IGT)
Impaired fibrinolysis (plasminogen activator inhibitor-1 [PAI-1], fibrinogen)
Inflammation (hs-CRP)
Increased oxidative stress (8-OHdG, F2 isoprostanes, glutathione—total and reduced)
Male—clinically or subclinically (trending) low free and total testosterone
Female—polycystic ovary syndrome (PCOS) (sex hormone binding globulin [SHBG], free testosterone)
NAFLD
Integrative Medicine
Nearly half of the US adult population utilizes integrative therapies to treat various medical conditions and modify disease risk, including risks for CM disease.11 Patients demonstrate reliance on these modalities out of philosophical interest in “natural approaches” to augment conventional medical therapy and to improve their levels of well-being. Unfortunately, because of the negative stigma associated with “nontraditional” treatment approaches, 70% of patients do not tell their physician about use of integrative approaches.9
Integrative medicine is an evidence-based combination of conventional, alternative, and complementary therapies. Integrative medicine makes use of conventional medicine, surgery, and treatments in combination with herbs, supplements, manual therapies, mind-body techniques, and other modalities to allow patients to achieve optimal health and healing while being actively involved in their own care.12
Over 45 academic centers in the United States have established integrative medicine programs, and 75% of medical schools now teach these approaches to medical students. The annual budget of the National Center for Complementary and Alternative Medicine at the National Institutes of Health is now over $120 million.13
Pathophysiology of Cardiometabolic Disease
CM disease results from a complex combination of etiologies derived from hormonal derangements in principally insulin, cortisol, thyroid, and sex hormones. In addition, inflammatory mediators (eg, interleukin (IL)-6, tumor necrosis factor alpha (TNFα), leptin, resistin, transforming growth factor (TGF) beta-1, hs-CRP), nutritional deficiencies (magnesium, omega fatty acids, chromium, vitamin C and D, and coenzyme Q10), hypercoagulability (PAI-1,fibrinogen), oxidative stress, environmental toxins, genetic polymorphisms, poor lifestyle habits, disrupted sleep and apnea, and psychosocial stress also play an important role and many times are acting simultaneously on the individual. These pages will focus on the more influential hormonal derangements of insulin, cortisol, and testosterone amplifying the underlying CM risk and central obesity.
Visceral Obesity, Insulin Resistance, and Inflammation
Visceral adipocytes release inflammatory markers known as adipocytokines into the circulation. These adipocytokines include TNFα, IL-6, leptin, resistin, angiotensinogen, and nonesterified fatty acids (NEFAs), all of which contribute to insulin resistance and cardiovascular risk.14 White adipose tissue is located around the viscera and has been shown to generate higher amounts of adipocytokines compared with subcutaneous fat, thereby highlighting its role in the development of central obesity and consequently type 2 diabetes and heart disease.15
Both hyperinsulinemia and insulin resistance have been implicated in CM disease. Elevated serum insulin is a well-recognized precursor to diabetes and heart disease, and it may be a stronger predictor of CVD and MetS than insulin resistance alone.14 Perpetuation of receptor tissue resistance to insulin causes increased insulin production as a compensatory mechanism to sustain normoglycemia. Excess NEFAs are transported to the liver where they are converted into very-low-density lipoproteins and triglycerides, thereby inducing hyperlipidemia. Insulin elevation also stimulates the sympathetic nervous system thereby leading to sodium and water retention and vasoconstriction resulting in an increase in blood pressure.9
Insulin resistance in the myocardium can generate damage by at least three different mechanisms: (1) signal transduction alteration, (2) impaired regulation of substrate metabolism, and (3) altered delivery of substrates to the myocardium.16
Accumulation of adipose tissue in the abdominal area is associated with CVD as well.3,17 Adipocytokines generated by these centralized adipocytes create a state of chronic systemic and local vascular inflammation, enhanced coagulation, and endothelial dysfunction.3 Inflammatory markers contributing additional risk for atherogenesis include hs-CRP, PAI-1, ox-LDL, and fibrinogen. When combined with modifiable traditional risk factors such as hyperlipidemia, smoking, and hypertension, the potent mixture of visceral obesity, insulin abnormalities, and inflammation contribute directly to the development of CM disease.
Stress and Hypothalamic-Pituitary-Adrenal Axis Dysregulation
Stress is a critical mediator of increasing CM risk. Integrity of HPA axis function is essential for maintaining homeostasis.18,19,20 Exposures to any stressors results in the stimulation of the “stress system” thereby inducing a myriad of adaptive hormonal responses designed to reestablish disrupted homeostasis and promote survival. These “fight or flight” responses are intended to ensure survival of the organism. Stress-induced HPA axis dysfunction has been reported to intensify insulin resistance, inflammation, endothelial dysfunction, neurological damage, and immunological damage.21,22
Under normal conditions, the HPA axis maintains a predictable pattern of diurnal variation under the influence of many factors. Cortisol is integral to normal HPA axis function and typically peaks before awakening and gradually decreases during the course of the day.23 A growing body of research has positively correlated an association between alterations in rhythmicity of cortisol release and various comorbid conditions including posttraumatic stress disorder
(PTSD),24 depression,25 chronic fatigue syndrome/fibromyalgia,26 metabolic syndrome,27 CM disease (MetS),28 cancer,29 and memory impairment.30
(PTSD),24 depression,25 chronic fatigue syndrome/fibromyalgia,26 metabolic syndrome,27 CM disease (MetS),28 cancer,29 and memory impairment.30
It is not only the loss of the normal diurnal pattern of cortisol release but also flattening of the normal diurnal cortisol curve that negatively impacts multiple physiological systems, including the cardiovascular system, metabolic regulation of energy balance, and sites of fat deposition.19,31,32,33
Chronic stress has been linked to the development and/or exacerbation of diabetes and heart disease. Cortisol directly affects insulin release and glucose regulation.19,33 Cortisol increases insulin levels, and the coelevation of these two hormones promotes visceral adipose deposition. Visceral fat has abundant glucocorticoid receptors and is very sensitive to the effects of cortisol and insulin.34,35 This cycle of events inevitably plays a role in the development of insulin resistance, hyperlipidemia, hypertension, and ultimately CM disease.3,36
Although many of the deleterious effects of chronic stress have been attributed to elevated levels of cortisol, there is ample medical evidence also suggesting a link between chronic stress, hypocortisolism, and/or low cortisol releasing hormone (CRH) release.3,37 Hypocortisolism is the paradoxical suppression of the HPA axis under conditions of chronic stress and is usually reported by patients as generalized fatigue. More commonly found in adults, hypocortisolism is thought to represent the cumulative effects of chronic stressors and mediates the progression of major chronic diseases such as CVD, cancer, cognitive and mood disorders, autoimmune diseases, as well as overall vitality and energy.18
Low cortisol production in response to chronic stress leads to loss of glucocorticoid receptor ligand activity3 and an increase in proinflammatory cytokines, including IL-6.38 The increase in inflammatory signaling due to loss of counterregulation by normal glucocorticoid activity is a crucial physiologic component of CM disease.
Although stress has long been associated with the development of diabetes and CVDs, the links between cortisol, insulin resistance, visceral adiposity, and increased inflammation have only recently been more fully appreciated. For the individual it does not matter whether they trigger high cortisol as a result of high insulin output due to excessive sugar and carbohydrate intake or trigger their metabolic dysfunction due to stress, the net result is the same as it relates to CM disruption. In the instance of systems biology network signaling, cortisol can lower the available free thyroid hormone, which in turns lowers signaling of insulin receptors as well as alterations in deposition of fat.
Testing for HPA Dysregulation
Although serum and urinary testing of cortisol and its metabolites has been traditionally utilized to diagnose the extremes of adrenal gland dysfunction, salivary cortisol testing has emerged as the preferred method of routine diagnostic testing.39 Salivary cortisol levels have been reported to correlate predictably with serum levels and allow determination of free cortisol levels by eliminating interference from serum cortisol binding globulin that precludes determination of the serum free cortisol fraction. Advantageous aspects to salivary testing include its noninvasive, convenient, and “stress-free” method of collection.40 Typically, salivary testing includes four samples during the course of a single day to determine the slope of cortisol production. A 24-hour AM urine cortisol testing may also be used; however, this method does not allow for observation of the diurnal variation in cortisol. Of note is the issue of chronic elevation of cortisol and its impact on gonadotrophin releasing hormone, which in turn causes a downregulation of sex hormones. The downstream effect leads to hypertension, insulin dysregulation, bone and muscle loss, and hypogonadism.
Testosterone Dysregulation
Testosterone dysregulation in both men and women can lead to increased CM risk. For men, testosterone deficiency (free and total) is a significant contributing risk factor to CM disease.41,42,43 It can result from any number of causes and affects men of all ages. The clinical signs and symptoms of this disorder depend on the age of the patient at onset and the degree of the deficiency. Testosterone deficiency in men typically manifests as symptoms of decreased erectile function and libido. Physiologically, testosterone plays a critical role in the regulation of normal growth, bone metabolism, and body composition.44
Testosterone in Men
Qualitative computed tomography (CT) imaging has confirmed that testosterone-deficient men have both a systemic increase in adipocyte deposits as well as alterations in adipocyte deposition. Studies have confirmed the significant relationship between serum free testosterone levels and the volume and distribution of body fat in both healthy and obese male subjects.45 Free testosterone levels have been reported to be inversely correlated with obesity in a number of studies, and the link between low testosterone and central obesity has been particularly striking.46,47
The relationship between central obesity and total testosterone is even more pronounced when combined with the presence of low SHBG levels. Because SHBG binds free serum testosterone, an increase in serum SHBG leads to even lower free testosterone levels in obese men. In a subgroup of the men in the HERITAGE family study, low total testosterone and SHBG were predictors of greater obesity and visceral fat levels on CT scan.48
Hypogonadism in men plays an important adjunctive role with central adiposity in the development of glucose intolerance and cardiovascular risk.49 Low serum testosterone levels are associated with both worsening insulin resistance as well as progression of atherosclerosis. Men with low testosterone levels have also been reported to be at higher risk for coronary artery disease, hypertension, and myocardial infarction.50
The use of total testosterone level as the initial assessment of hypogonadism in men has been advocated by the Institute of Medicine and in several recent guidelines.51 No formal consensus exists on the presence of CM disease and how it is correlated with measurements of total testosterone, although low testosterone is present in 15% to 30% of men with obesity and diabetes. But free testosterone is the bioavailable testosterone.
The diagnostic threshold of hypogonadism in men remains a subject of debate. The American Association of Endocrinologists recommends 300 ng/dL (10.4 nmol/L) as the lower limit, and initiation of testosterone therapy is recommended when levels are 200 ng/dL (8 nmol/L) or less. Other guidelines recommend initiating testosterone replacement when serum testosterone levels are between 8 and 12 nmol/L and clinical signs and symptoms warrant therapy.52 Serum testosterone levels, like the other sex hormones, have a circadian rhythm. Because testosterone levels tend to be the highest in the morning, it is recommended that testing be conducted at this time. However, several studies have shown that the circadian rhythm is often lost in elderly men.53
An assessment of serum SHBG levels is also important, particularly in elderly men, obese men, and men with underlying CM disease. The SHBG can then be used to calculate bioavailable testosterone levels when total testosterone levels are known. The bioavailable testosterone levels provide an assessment of both the free testosterone as well as the weakly bound to albumin portion that is readily available in tissue when needed.53 Additional hormonal evaluations should include measurements of follicle stimulating hormone (FSH), luteinizing hormone (LH), and prolactin as indicated. If testosterone therapy is initiated, a baseline prostate specific antigen (PSA) and prostate examination should be performed. At the 3-month follow-up visit, repeat testing is recommended of total or bioavailable testosterone and SHBG, hematocrit, and PSA (free and total).54
Testosterone in Women
In women, testosterone also plays a key role in cardiovascular systems networking. A higher testosterone-to-estrogen ratio could be a strong risk factor for future CVD, coronary heart disease, and heart failure among postmenopausal women.55 High free testosterone in women and low SHBG can lead to symptoms of polycystic ovary disease or PCOS.56 PCOS increases CM risk, including hypertension, insulin resistance and insulin signaling problems, dyslipidemia, and obesity.57,58
A 2018 study in the American Journal of Cardiology reported these results from an average follow-up of 12.1 years in 2834 postmenopausal women participating in the MESA (Multi-Ethnic Study of Atherosclerosis) 2000 to 2002, using Cox hazard models to evaluate associations of sex hormones with CV outcomes55:
Each standard deviation (SD) increase in total testosterone levels was associated with risk increases of 14% for CVD, 20% for coronary heart disease, and 9% for heart failure.
Each SD increase in estradiol (an estrogen steroid hormone) was associated with risk reductions of 6% for CVD, 23% for coronary heart disease, and 22% for heart failure.
Each SD increase in testosterone-estradiol ratio was linked to risk increases of 19% for CVD, 45% for coronary heart disease, and 31% for heart failure.
Another group used the MESA of 2000 to 2002 using 2759 postmenopausal women (ages 65 + 9 years) and reported that a more androgenic hormones profile in these women, higher free testosterone and lower SHBG, is associated with an increase in coronary artery calcification up to 10 years and a greater increase in left ventricular mass in men and women.59 Women with higher free testosterone and SHBG also reported an increase in mass:volume ratio over the course of 9 years.60
Exogenous estrogen, particularly when administered orally, increases SHBG, which, in turn, reduces free T and estradiol (E2). Lower levels of testosterone in women can lead to clinical symptoms such as:
Fatigue
Decreased feeling of well-being
Low motivation
Muscle weakness
Sleep problems
Weight gain
Decreased sexual libido
DIND/DIMD and Cardiometabolic Implications
One of the potential challenges facing heath care professionals today is the problem of drug-induced disease, including drug-induced nutrient depletion (DIND) and drug-induced microbiome disruption (DIMD).
DIND and DIMD in patients, including the cardiovascular patient, are important issues for the 21st century clinician to embrace and implement corrective measures into practice, which can be as simple as a multiple vitamin/mineral supplement. With over 48% of people in the United States taking prescription medications (up from 44% the previous decade), patients with CVD are placed a much higher risk for developing comorbid conditions and for increasing disease pathologies.61 Polypharmacy prescribing is now occurring in younger and younger populations, so it is becoming increasingly important to assess nutrient depletion risks as they relate to increased drug side effects, future symptoms, comorbid health condition development, or progression of the underlying disease itself.
It is clear that a viable and thriving gut microbiome is essential for homeostasis. When this balance is disrupted, called dysbiosis, homeostasis is lost. One example of this is circulating endotoxin. As microflora is destroyed owing to reduced blood perfusion, high fat, low-fiber diets, or drug therapy, the cell wall fragments known as lipopolysaccharide (endotoxin) enter circulation potentially
crossing the blood-brain barrier along with attaching to receptors of cells on other tissues. This triggers nuclear factor kappa-B and the full cascade of inflammatory cytokines. Elevated serum endotoxin has been associated with the progression of metabolic syndrome as well as an increased risk for cardiovascular events. An example of this could be elevated cortisol signaling from the brain, increased catecholamines, decreased blood perfusion to the gut leading to vascular inflammation, and reduced pliability. The network of brain-gut-heart becomes a driver for progression of CM disease.
crossing the blood-brain barrier along with attaching to receptors of cells on other tissues. This triggers nuclear factor kappa-B and the full cascade of inflammatory cytokines. Elevated serum endotoxin has been associated with the progression of metabolic syndrome as well as an increased risk for cardiovascular events. An example of this could be elevated cortisol signaling from the brain, increased catecholamines, decreased blood perfusion to the gut leading to vascular inflammation, and reduced pliability. The network of brain-gut-heart becomes a driver for progression of CM disease.
The gut is now being called the “second brain.” The gut-immune-brain axis helps control and is controlled by many body system networks, including the HPA axis, blood glucose and insulin regulation, sex hormone axes, detoxification systems, neurological disorders, and the cardiovascular system. Strong evidence exists supporting the fact that many chronic health conditions that are seen clinically are related to microbiome disruption—inflammatory bowel disease and other gastrointestinal (GI) conditions, liver cirrhosis, rheumatoid arthritis and autoimmune conditions (chronic inflammation), insulin resistance and type 2 diabetes, cognitive decline, depression, anxiety, schizophrenia and psychosis, cardiovascular conditions, and cancer.
There are many ways by which the gut microbiome network can become disrupted—poor food choices (high sugar/refined carb content, chemical food additives/preservatives/dyes, pesticide residues, acidic pH foods, and inflammatory foods like red meats), high levels of stress, infections, and many oral drugs that are taken can lead to dysbiosis.
In the medical management of individuals with CVD or comorbid conditions, several classes of prescription drugs may be employed with the potential for depletions of nutrients, which could induce metabolic changes and further the progression of any component of the underlying cardiovascular problems or comorbidities. Medications that are commonly used in the management of a patient with CVD include:
Diuretics
Angiotensin-converting enzyme inhibitors
Angiotensin II receptor blockers
Beta-blockers
Calcium channel blockers
Hypocholesterolemic agents
Antidiabetic medications
Anticoagulant/antiplatelet agents
Nitrates
Digitalis
Pain medications, including opiates, acetaminophen, and nonsteroidal anti-inflammatory drugs
Acid-reducing drugs, including H2 blockers or proton-pump inhibitors (PPIs)
Antianxiety/sleep medications
Discussion of DIND is covered in another chapter, but an example of DIMD are PPIs. PPIs also are reported to significantly disrupt the human gut microbiome. PPIs lower gut acidity, which can lead to imbalances in the normal microflora—more species of nonhost microbes can thrive. Meta-analyses have reported that use of PPIs was associated with an increased incidence of Clostridium difficile infection if not treated aggressively.62 Higher rates of C difficile recurrence in hospitalized patients and increased risk of other enteric infections and community-acquired Streptococcus pneumonia are also contributed to by the overuse of PPIs.63,64 A recent study of 400 cirrhotic patients reported that PPI prescription was an independent predictor of infection.64 Other studies suggest PPI microbiome disruption can lead to increases in esophageal and gastric cancers.65
Diagnosis and Evaluation of the CM Patient: A New Perspective
Evaluation of patients who may be at risk for CM disease includes not only identifying the presence of traditional cardiac risk factors but also delineation of underlying hormonal status. Identification of important hormonal abnormalities including insulin resistance and hyperinsulinemia, HPA axis dysregulation, and sex hormone (testosterone deficiency, estrogen imbalances) is essential. Furthermore, in patients who already have metabolic syndrome, evaluation of these hormones is particularly important in CM risk stratification. In essence it is important to get a thorough picture of what type of proinflammatory chemistry is present and more importantly what the systems biology factors driving the metabolic expression are.
Systems biology networking should be considered using laboratory analysis. These considerations are in addition to advanced markers directly related to CM risk such as Lpa, MPO, oxLDL, Kif 6, LpPLA2, LDL-p, and other markers mentioned throughout the text.
Stress levels (using five-point salivary cortisol OR in the least 8 AM serum cortisol) compared with DHEAs is important to determine HPA axis involvement.
Thyroid profile, including TSH, free and total T3 and T4, thyroid antibodies (ThyrAb), or thyroid peroxidase.
Fasting blood glucose, HbA1c and insulin, cystatin C. HDL, lactate dehydrogenase (LDH), vitamin D, red blood cell (RBC) chromium and magnesium, and serum potassium also impact blood glucose regulation.
Gut assessment—does the microbiome need support? Is the patient currently taking medications that can alter the microbiome? Are the patient’s symptoms diarrhea/constipation, belching/burping, abdominal discomfort, feeling tired after eating large meals, weight gain, mental fogginess, immune dysregulation? Evaluating GI microflora and endotoxin may provide valuable insight into the inflammatory process.
Sex hormone analysis for men and women—serum free and total testosterone, estradiol, estrone, SHBG, DHT, and urinary metabolites of estrogen 4OH and 16-alpha derivatives.
Integrative Treatment Protocols
Once a patient with MetS has been identified by presenting with underlying hormonal derangements of insulin, cortisol, and/or testosterone, several integrative medicine treatment options can be considered. Conventional hormone replacement and medications may be considered as part of an overall treatment plan if necessary. However, bioavailable hormonal replacement therapies (HRTs) are preferred owing to side effects that can occur with the use of synthetics. Patients report greater satisfaction when using bioavailable HRT, especially with progesterone replacement, and not synthetic progestins.66 Studies of both bioidentical estrogens and progesterone suggest a reduced risk of blood clots compared with nonbioidentical preparations.67
Dietary Supplements
Dietary supplementation that includes botanicals, nutrients, and nutraceuticals can help improve TRIAD balance and improve CM patient outcomes. Always use quality products from reputable manufacturers that follow strict good manufacturing practices.
TRIAD Network 1 Adrenal-Thyroid-Pancreas
Alpha-Lipoic Acid
α-Lipoic acid, also known as lipoic acid (ALA) or thioctic acid, and its reduced form, dihydrolipoic acid, are potent antioxidants and help improve glutathione levels. ALA is an essential cofactor for mitochondrial bioenergetic enzymes and functions as an antioxidant and anti-inflammatory agent.68 ALA is reported in clinical studies to improve insulin sensitivity, improve glycemic control, and help improve symptoms and incidence of neuropathies.69,70 ALA is reported to help activate AMP-K, which upregulates PGC-1 alpha, reducing insulin secretion, improving fatty acid and glucose utilization, and regulating cell growth.71,72 ALA is also reported in laboratory animal studies to reduce the neurotoxic effects of heavy metal exposure, including lead, mercury, and cadmium.73,74,75
A general dose of ALA is 300 to 1200 mg daily.
Bitter Melon
Bitter melon (Momordica charantia) is a green, bitter vegetable from the gourd family, grown in tropical and subtropical regions throughout the Amazon, East Africa, Asia, the Caribbean, and South America and used as a food for diabetes and blood sugar control in these areas. Extracts are reported to contain poly-peptide P, similar in structure to bovine insulin. Laboratory studies have found that bitter melon may enhance insulin secretion by the islets of Langerhans, reduces glycogenesis in liver tissue, enhances peripheral glucose utilization, and increases serum protein levels.76,77 Bitter melon activates PPAR gamma, an important metabolic pathway in glucose and weight control.78 Clinical conditions for which bitter melon extracts are currently being used include diabetes, dyslipidemia, and microbial infections. It was found to decrease blood sugar levels when injected subcutaneously into type 1 diabetic patients.79 Bitter melon supplements also help with obesity and symptoms of metabolic syndrome.
The dose is generally at 250 to 500 mg two times daily standardized to 10% charantins. Bitter melon is usually combined with other blood glucose supportive nutrients, including chromium, cinnamon, ALA, benfotiamine (a special form of thiamin B1), and pyrroloquinoline quinolone (PQQ, helps with mitochondrial energy production).
Chromium
Chromium supplementation has been reported in clinical trials for over 5 decades to improve insulin regulation and glucose tolerance in people with type 1 and 2 diabetes, gestational diabetes, and steroid-induced diabetes.80,81 Chromium depletion can lead to hyperlipidemia, insulin resistance, fatigue, accelerated atherosclerosis, hypertension, anxiety, impaired wound healing, decreased glucose tolerance, and possibly infertility.82 Some studies have reported negative clinical outcomes in patients supplementing chromium. However, low doses and poorly absorbable forms of chromium used in these studies may have negatively affected the therapeutic outcomes. Chromium is not found in sufficient amounts in food to replenish tissue stores or to affect clinically significant improvement in blood glucose control.82
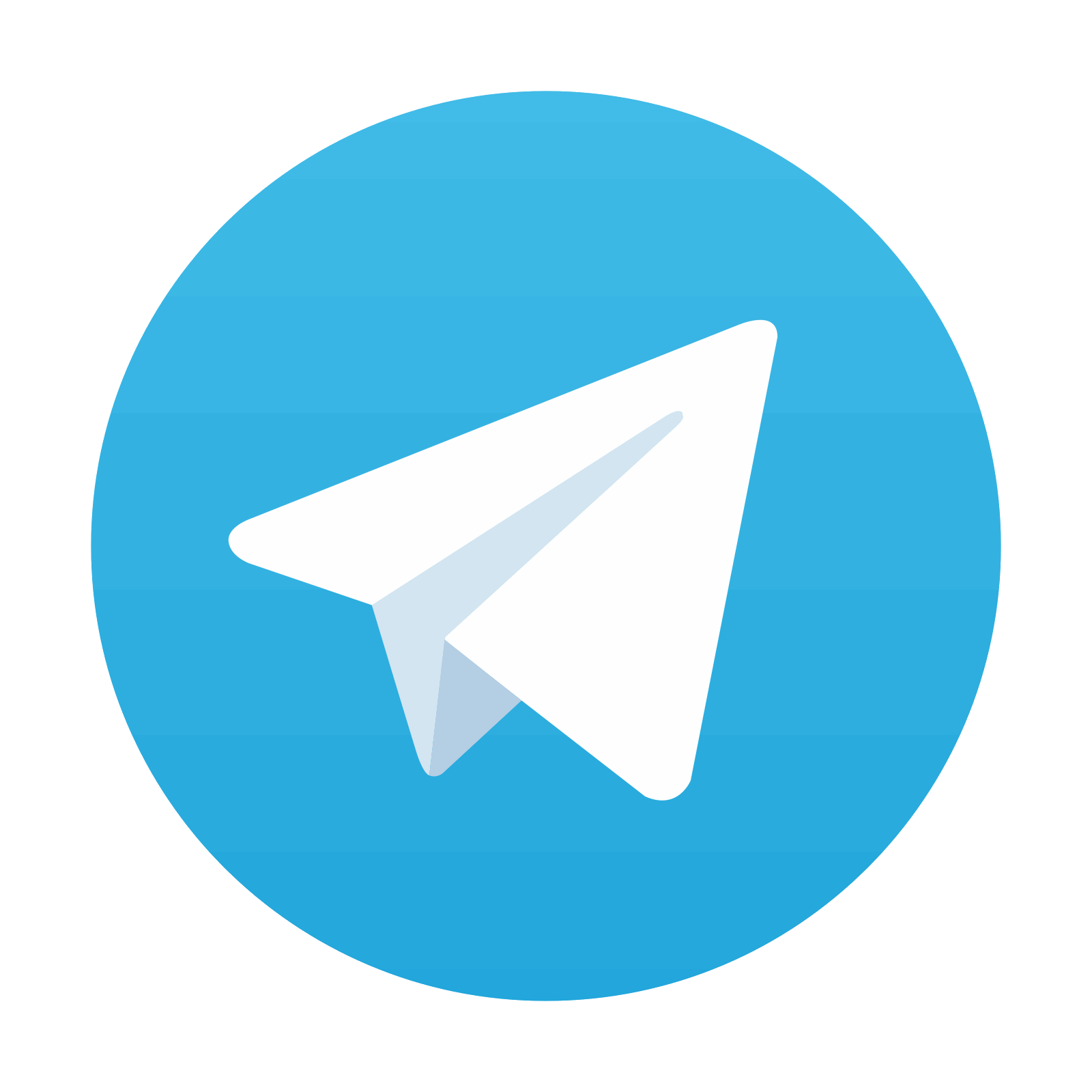
Stay updated, free articles. Join our Telegram channel

Full access? Get Clinical Tree
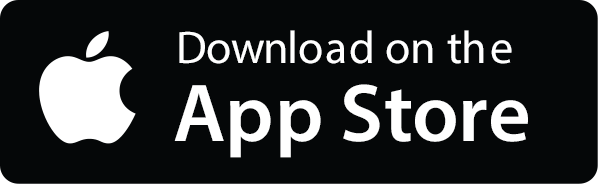
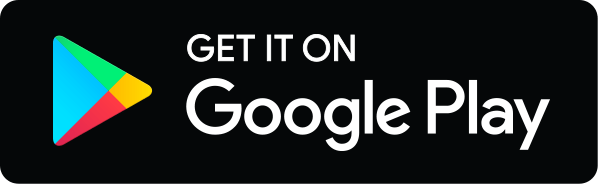