Coronary reimplantation during arterial switch operation (ASO) may affect coronary artery growth and function during childhood. The purpose of this study was to assess coronary artery diameter and regional myocardial blood flow (MBF) and myocardial flow reserve (MFR) in children after neonatal ASO. We measured proximal diameters of left anterior descending (LAD), left circumflex, and posterior descending coronary arteries on coronary angiogram in 12 children (median age 11 years, range 7.6 to 15.1) with a history of neonatal ASO. These children then underwent cardiac positron emission tomographic imaging using nitrogen-13 ammonia to assess MBF at baseline and during intravenous adenosine hyperemia in regions supplied by these 3 coronary arteries. Coronary artery z-scores were within normal range (−2.0 to 2.0) for 32 of 36 coronary arteries. MFR (ratio of hyperemic to basal MBF) was normal (>2.5) in all myocardial regions in 10 of 12 patients. The remaining 2 patients, 1 with a dual LAD and 1 with LAD origin from the right coronary artery, had generalized impairment of hyperemic MBF (<2.0 ml/g/min) and low MFR (<2.5). Coronary artery z-scores and MFR in corresponding myocardial territories were not correlated (r = 0.15, p = 0.36). In conclusion, coronary growth and function appear to be normal in most children after neonatal ASO. Children with anatomic LAD abnormalities may be at increased risk of impaired MFR.
Neonatal arterial switch operation (ASO) is the standard surgical procedure for transposition of the great arteries. Translocation and reimplantation of the coronary arteries to the neoaorta is essential in this surgery. The effect of neonatal coronary manipulation on coronary artery growth and on coronary vascular function remains a source of concern. Indeed, previous studies using quantitative coronary angiography have suggested that the left coronary artery system may be smaller in children after ASO than in age-matched controls. These anatomic coronary abnormalities may explain some of the myocardial perfusion abnormalities observed in these children.
Cardiac positron emission tomographic (PET) imaging allows noninvasive quantification of regional myocardial blood flow (MBF). Quantification of MBF using nitrogen-13 ammonia as a tissue blood flow tracer and PET imaging has been extensively validated and is useful in assessing adults with coronary artery disease. Previous studies in children after ASO using PET imaging have reported lower myocardial flow reserve (MFR) in these children compared to young adults as controls. These studies did not describe anatomic (coronary angiographic) and physiologic (MFR) findings in the same cohort of ASO survivors. In this study, we measured proximal diameters of the major coronary arteries (left anterior descending [LAD], left circumflex, and posterior descending) using quantitative coronary angiography in children with a history of neonatal ASO and assessed MBF and MFR in corresponding myocardial regions in these children using cardiac PET imaging.
Methods
The goal of this study was to assess regional MFR and to relate it to anatomic coronary findings in children with a history of neonatal ASO for transposition of the great arteries. Children who had undergone a routine cardiac catheterization for clinical follow-up of ASO were approached for cardiac PET imaging in a research protocol. The study was approved by the human investigation committee at Wayne State University School of Medicine (Detroit, Michigan). Parental consent and subject consent/assent, as applicable, were obtained for all patients.
Children ≥8 years of age with a history of neonatal ASO for transposition of the great arteries at Children’s Hospital of Michigan (Detroit, Michigan) were eligible for participation in the PET study if they had undergone cardiac catheterization during the previous 2 years. Exclusion criteria were (1) presence of a ventricular paced rhythm, (2) patch closure of a muscular ventricular septal defect, (3) more than mild mitral or aortic valve insufficiency, and (4) more than mild supravalvar pulmonary stenosis with right ventricular systolic pressure >1/2 left ventricular systolic pressure. All children underwent selective coronary angiography during their cardiac catheterization. Coronary angiograms were evaluated by an investigator blinded to PET imaging data. Proximal diameters of the LAD and left circumflex and posterior descending coronary arteries, before the origin of any branches, were measured using a digital caliper and the known catheter diameter for calibration. These diameters were converted to standardized values (z-scores) using published normal coronary branch diameter dimensions in children. In addition, abnormalities of coronary artery anatomy (such as stenosis, occlusion, atypical origin, or branching pattern), if present, were noted.
MBF was assessed at rest and during adenosine hyperemia using nitrogen-13 ammonia as a tracer of tissue blood flow and cardiac PET imaging. All subjects were instructed to refrain from caffeine-containing beverages or theophylline-containing medications for 24 hours before the study. PET imaging was performed using a whole-body positron emission tomograph (CTI EXACT HR, Siemens, Knoxville, Tennessee). The scanner acquires 47 contiguous transaxial planes and covers a 15-cm axial field of view. Images were reconstructed using a Hanning filter with 1.1 cycles/cm cut-off frequency, yielding an isotropic resolution of about 6 mm full width half-maximum.
A 15-minute transmission scan was acquired for correction of photon attenuation. Beginning with a slow intravenous bolus administration of nitrogen-13 ammonia (0.286 mCi/kg body weight) over 1 minute, serial images were acquired for 20 minutes (12 frames of 10 seconds each, 4 frames of 30 seconds, 1 frame of 60 seconds, and 1 frame of 900 seconds). Thirty minutes later, after physical decay of nitrogen-13 ammonia, intravenous adenosine 140 μg/kg/min was infused over 4 minutes. Two minutes after initiation of adenosine infusion (maximal hyperemia), a second dose of nitrogen-13 ammonia (0.286 mCi/kg) was injected and images recorded in the same acquisition sequence. Heart rate, blood pressure, and electrocardiogram were monitored throughout the study. An investigator blinded to coronary angiographic data analyzed MBF data using dynamic PET studies. In each PET study, the 47 tomographic slices were reoriented into 12 short-axis slices of the heart extending from the apex to the base of the left ventricle using a SUN workstation (SUN Microsystems, Inc., Palo Alto, California). To quantify regional MBF, myocardial sectors of interest encompassing the LAD and left circumflex, and posterior descending coronary artery territories were automatically assigned to each of the 4 midventricular short-axis slices of the nitrogen-13 ammonia images. An additional, small, circular region of interest was manually placed in the center of the left ventricular blood pool to obtain arterial input function. Regions of interest were then copied to the entire nitrogen-13 ammonia dynamic image sequence and regional myocardial tissue and blood pool time–activity curves obtained. In each vascular territory, a single time–activity curve was obtained by averaging corresponding nitrogen-13 ammonia data in adjacent ventricular planes. Time–activity curves were then fitted with a previously validated 3-compartment tracer kinetic model. Because MBF at rest is related to rate–pressure product, an index of cardiac work, we corrected basal MBF for rate–pressure product for each patient. MFR for each coronary territory (LAD, left circumflex, and posterior descending) was defined as the ratio between hyperemic and corrected basal MBF.
Patient data are presented as mean ± SD or median (range) for continuous variables. Changes in hemodynamics (heart rate, systolic blood pressure, rate–pressure product) and regional MBF from baseline to maximal hyperemia were assessed using paired Student’s t test. Assessment of homogeneity of flow in the 3 myocardial regions was performed using repeated measures analysis of variance. The relation between coronary artery diameter expressed as a z-score and MFR was examined using a correlation analysis. A p value <0.05 defined statistical significance.
Results
Twelve children participated in the study. Their median ages were 11.0 years (range 7.6 to 15.1) at the time of coronary angiography and 11.7 years (range 8.1 to 16) at the time of their PET study. Median duration between cardiac catheterization and PET study was 3.6 months (range 0 to 19). All subjects had normal left ventricular function at rest by echocardiography. None of the subjects were on any cardiac medication. Five children had the usual separate origins of the left and right coronary arteries (type A), 4 had left circumflex origin from the right coronary artery (type D), and 2 had LAD origin from their right coronary arteries (type E). In 1 child, the LAD had a dual origin—from the left and right coronary arteries ( Figure 1 ). Distributions of the LAD and left circumflex and posterior descending coronary artery diameter z-scores in study subjects are illustrated in Figure 2 . Coronary artery z-scores were normal (−2.0 to 2.0) for 32 of 36 coronary arteries. All coronary artery z-scores were normal in 9 of 12 patients. Two patients had a posterior descending artery z-score <2.0 as an isolated abnormality. The remaining patient had a distal occlusion of the left circumflex coronary artery, a small proximal left circumflex coronary artery (z-score −2.38), and a compensatory dilation of the posterior descending coronary artery (z-score 3.0).
Table 1 presents hemodynamic changes in study subjects during adenosine infusion. Heart rate, systolic blood pressure, and rate–pressure product increased significantly during intravenous adenosine, but mean blood pressure (a measurement of coronary perfusion pressure) did not change significantly from baseline.
Variable | Baseline | Adenosine | p Value ⁎ |
---|---|---|---|
Heart rate (beats/min) | 67 ± 9 | 109 ± 12 | <0.001 |
Systolic blood pressure (mm Hg) | 109 ± 13 | 116 ± 15 | 0.025 |
Mean blood pressure (mm Hg) | 77 ± 9 | 82 ± 15 | 0.065 |
Rate–pressure product † | 7,254 ± 1,000 | 12,767 ± 2,481 | <0.001 |
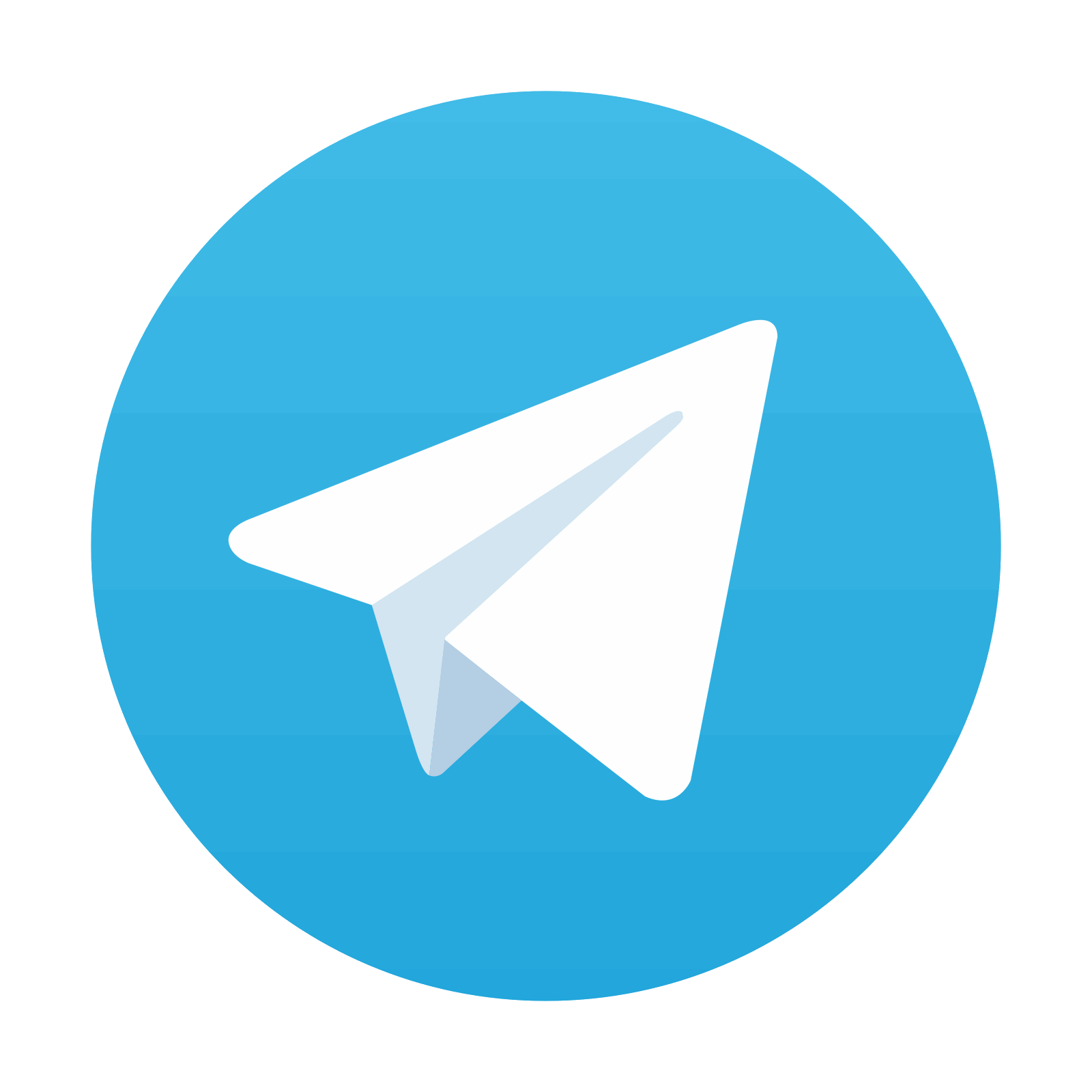
Stay updated, free articles. Join our Telegram channel

Full access? Get Clinical Tree
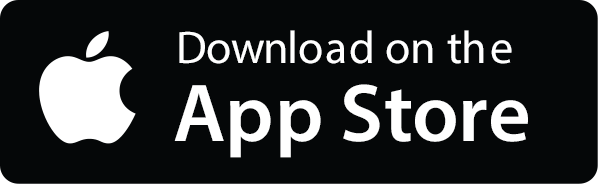
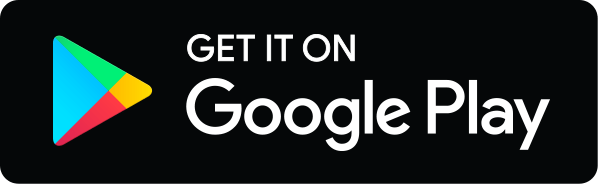
