Heterozygous familial hypercholesterolemia (HeFH) can be associated with early coronary artery disease (CAD) in asymptomatic patients. The objectives of the present study were to assess the prevalence and magnitude of subclinical CAD in patients with HeFH using coronary computed tomographic angiography (CCTA) and to determine the clinical and genetic profile of those at the greatest risk of CAD. The study included 50 consecutive patients with HeFH diagnosed according to the Dutch Lipid Clinic Network criteria and a control group of 70 healthy subjects. The findings from CCTA for the patients with HeFH were compared with those from the control group, who had been referred for CCTA as a part of a preventive medical examination. In 82% of the patients with HeFH, genetic DNA was screened for low-density lipoprotein receptor (LDLR) gene mutations using a microarray. CCTA revealed a significantly greater Agatston calcium score in the study group than in the control group (260 vs 46; p = 0.002). The prevalence of CAD in the patients with HeFH was 48%. It was significant in 26%, involving mainly the proximal segments of the coronary arteries. In the control group, the prevalence of CAD was 33% and was significant in 5% (p <0.05 for prevalence and severity of CAD compared to patients with HeFH). In those with HeFH, increased age, null allele LDLR mutations, and low high-density lipoprotein blood levels at diagnosis showed a statistically significant association with CAD (p <0.05). In conclusion, patients with HeFH present with a greater prevalence, extension, and severity of subclinical CAD than the general population. Increased age, low high-density lipoprotein levels, and LDLR null allele mutations are related to the occurrence of CAD. CCTA has emerged as a useful technique for the screening of subclinical CAD in patients with HeFH.
Heterozygous familial hypercholesterolemia (HeFH) has an autosomic, dominant inheritance. It is generally monogenic and is characterized by high blood cholesterol levels, xanthomas, and early and severe atherosclerotic disease. The prevalence of the disease has been estimated at 1 in 500 persons worldwide. This could represent 2,000,000 cases in Europe and lead to a mean of 30,000 to 40,000 myocardial infarcts annually, generally at a young age (30 to 40 years). Only 10% of HeFH cases are diagnosed, with a mere 25% of patients receiving lipid-lowering therapy, even though lipid-lowering therapy decreases the cardiovascular risk and improves survival by 10 to 30 years, with an excellent cost/benefit ratio.
Phenotypic expression can be caused by several mutations. These include a gene encoding the low-density lipoprotein receptor (LDLR), B-100 apolipoprotein (ApoB-100), lipoprotein-convertase type 9, and LDLR adaptor protein 1. The latter has an autosomic recessive pattern of inheritance. Until February 2012, 1,741 allelic variants of LDLR had been identified, 21 for apoB-100, 163 for lipoprotein-convertase type 9, and 39 for LDLR adaptor protein 1.
The clinical diagnosis of HeFH is made from focused questionnaires such as the Dutch Lipid Clinic Network (DLCN), Simon Broom Register, or Utah Make Early Diagnosis to Prevent Early Deaths (MEDPED) criteria. The cardiovascular risk in these patients is systematically underestimated using standard clinical scores, such as the Framingham or Systematic COronary Risk Evaluation Risk Charts (SCORE) risk charts. Screening for subclinical coronary artery disease (CAD) in patients with HeFH has been based on stress-induced myocardial ischemia tests or the assessment of noncoronary vascular disease. During the past decade, noninvasive techniques for the direct visualization of coronary arteries have allowed the assessment of CAD in these patients, either by study of coronary artery calcification or direct examination of the vessel lumen using coronary computed tomographic angiography (CCTA). CCTA has shown a diagnostic capability comparable to that of invasive methods, with a high negative predictive value.
With these considerations, the objectives of the present study were to assess the prevalence and magnitude of subclinical CAD in patients with HeFH using CCTA and comparing their findings with those from the general population. Also, we sought to determine those variables (clinical, genetic, or analytical) associated with the findings from CCTA and, finally, to determine the profile of patients with HeFH who would benefit from screening for subclinical CAD using CCTA.
Methods
A total of 50 consecutive patients (50% men, mean age 48 ± 13 years) with HeFH diagnosed using the DLCN criteria in the lipid unit of our institution and without clinical data of previous CAD were prospectively included as the study group. The DLCN criteria are useful as a probabilistic scale to diagnose HeFH clinically but requires confirmation by genetic tests and sequencing. Using these tests, a definite diagnosis of the patient and also of their relatives is possible in nearly 72% of cases. A total of 70 healthy subjects (44% men, mean age 47 ± 14 years) who were referred for CCTA as a part of a preventive medical examination from a private company were consecutively included as the control group. All patients and subjects were in normal sinus rhythm at the moment of the examination and none was receiving lipid-lowering treatment. No patients presented with a contraindication for CCTA (body mass index >40 kg/m 2 , allergy to iodinated contrast, estimated glomerular rate <30 ml/kg/1.73 m 2 , or inability to perform a 10-second apnea). A clinical and analytical follow-up examination was performed within 12 to 24 months for all patients from the study group. Our study complied with the Declaration of Helsinki, and our ethics committee approved the study. All patients provided written informed consent.
CCTA was performed using a 64-slice Toshiba Aquilion system (Toshiba Medical Systems, Otawara, Japan). A noncontrast-enhanced scan was performed before CCTA to assess the total coronary calcium burden. This scan was prospectively triggered at 75% of the RR interval and performed using the following scan parameters: collimation, 4 × 3.0 mm; gantry rotation time, 400 to 450 ms; tube voltage, 120 kV; and tube current, 200 mA.
For the contrast-enhanced scan, the collimation was 64 × 0.5 mm, and the rotation time was 400 or 450 ms, depending on the heart rate. The tube current and voltage was 320 mA (range 300 to 400) and 100 to 120 kV (120 kV in patients with a body mass index >30 kg/m 2 ), respectively. The average dose of contrast (Xenetix 350, Guerbet, Aulnay-sous-Bois, France) was 73.5 ml (range 50 to 120), followed by a saline flush of 40 ml, both injected at a rate of 5 to 6 ml/s. All patients with a heart rate >70 beats/min received intravenous β-blocker therapy (metoprolol tartrate 2.5 to 10 mg) before the computed tomographic scan, if no contraindications were present. Care was taken to minimize the radiation dose by using adequate kilovoltage (100 kV) and mA (300 mA) values in patients with a body weight <90 kg. The average effective radiation dose was 14.2 mSv.
Coronary computed tomographic angiographic analysis was performed according to a 17-segment modified American Heart Association classification. All data sets were analyzed on an off-line workstation (Vitrea2, Vital Images, Plymouth, Minnesota). The readers were required to meet the criteria for level 3 cardiac computed tomographic training. We considered significant coronary stenosis to be a reduction in luminal diameter >70% of the reference luminal diameter.
The clinical diagnosis of HeFH was according to the DLCN criteria. In 41 of 50 patients (82%), the genetic DNA was screened for LDLR gene defects using a microarray (Lipochip, Progenika Biopharma, Derio, Spain) designed to detect prevalent mutations in the Spanish population that is periodically updated as new mutations are identified. The seventh version of this Lipochip test simultaneously measures >240 mutations in the LDLR, 3 major mutations in ApoB-100, and 6 mutations in lipoprotein-convertase type 9. The diagnostic accuracy to detect any of these 240 mutations is close to 100%. The nomenclature of each mutation was according to the Human Genome Variation Society recommendations. The chromosome location, mutation type, protein expression and “in vitro” functional effect of each mutation were assessed. The functional effect of each mutation was classified into 2 categories according to the “in silico” biosynthetic and functional studies with strains of fibroblasts using PolyPhen (polymorphism phenotyping) and SIFT (sorting intolerant from tolerant) predictions. These mutations have been classified into 2 groups:
Null allele LDLR mutations (<15% “in vitro” functionality in homozygotes, <55% functionality in heterozygotes), which include promoter mutations, large rearrangements, nonsense and frameshift mutations, or missense mutations, resulting in a deletion of the translation initiation signal and early stop codons, which result in no protein synthesis.
Defective allele LDLR mutations (>15% “in vitro” functionality in homozygotes, >55% “in vitro” functionality in heterozygotes), which include transcription and missense mutations without proven alteration of LDLR function and residual receptor activity.
Continuous variables with a symmetric distribution are expressed as the mean ± SD and those with an asymmetric distribution as the mean and interquartile range. Categorical variables are expressed as percentages. For quantitative variables, which satisfied the normal and homogeneity of variance assumptions, a t test for 2 independent groups and analysis of variance test for >2 independent groups was applied. For categorical variables, the chi-square test with the corresponding contingency table was used. To assess the relation between the clinical cardiovascular risk and CCTA variables that did not satisfy the normal assumptions, the nonparametric test (Mann-Whitney U test) was used.
Multivariate analysis with stepwise logistic regression analysis was performed to assess the independent variables associated with an abnormal CCTA study. Changes in the lipid profile during the follow-up period were compared in those patients with and without subclinical CAD using a general linear model. To assess the different response to treatment between defective and null allele carriers, we used a nonparametric test (Mann-Whitney U test). p Values ≤0.05, 2-tailed approach, and power of 80% were used in all tests. Statistical analysis was performed using SPSS software for Windows, version 18 (SPSS, Chicago, Illinois).
Results
The patients from the study and control groups were comparable in terms of clinical and demographic data, except for the presence of xanthoma, a corneal arch, and a family history of CAD ( Table 1 ). The clinical and laboratory data at inclusion from the HeFH group are listed in Table 2 . CCTA showed a significantly greater Agatston calcium score in the study group than that in the control group (204.2 vs 46; p = 0.002). The prevalence of CAD in the patients with HeFH was 48% (27 of 50) and was significant in 26% (13 of 50; Table 3 ). Atherosclerotic lesions were more prevalent in the proximal segments of the epicardial coronary arteries ( Figure 1 ), with a distribution, from the most to least common, of left anterior descending artery, right coronary artery, left circumflex artery, and left main artery ( Figure 2 ). In the control group, the prevalence of CAD was 32% (23 of 70) and was significant in 5% (4 of 70). Normal coronary computed tomographic angiographic findings were more prevalent (62% vs 50%, p = 0.041) in the control group, and the prevalence of significant obstructive coronary lesions was greater in patients with HeFH (26% vs 5%, p = 0.032).
Variable | Control Group (n = 70) | HeFH Group (n = 50) | p Value |
---|---|---|---|
Age (yrs) | 48.6 ±12.6 | 47.6 ± 14 | 0.755 |
Men | 44% (31/70) | 50% (25/50) | 0.581 |
Smokers | 45.7% (32/70) | 54% (27/50) | 0.215 |
Hypertension | 11.4% (8/70) | 8% (4/50) | 0.772 |
Diabetes mellitus | 2.9% (2/70) | 1.7% (1/50) | 0.502 |
Body mass index (kg/m 2 ) | 25.6 ± 5.2 | 26.8 ± 4.2 | 0.234 |
Xanthoma | 0% (0/70) | 25% (12/48) | 0.000 |
Xanthelasma | 0% (0/70) | 4% (2/48) | 0.197 |
Corneal arch | 0% (0/70) | 8% (4/48) | 0.037 |
Vasculopathy ∗ | 0% (0/70) | 2% (1/47) | 0.446 |
Stroke | 0% (0/70) | 4% (2/48) | 0.056 |
Family history of coronary artery disease | 1.4% (1/70) | 58% (28/48) | 0.000 |
∗ Intermittent claudication in the lower limbs as a clinical presentation of peripheral artery disease, ankle-brachial index <0.9.
Variable | Value |
---|---|
Interval from heterozygous familial hypercholesterolemia diagnosis (mo) | 162 ± 88 |
Treatment duration (mo) | 157 ± 90 |
Total cholesterol | |
mmol/L | 8.4 ± 2 |
mg/dl | 321 ± 75 |
Low-density lipoprotein | |
mmol/L | 6.4 ± 2.1 |
mg/dl | 246 ± 79 |
High-density lipoprotein | |
mmol/L | 1.4 ± 0.4 |
mg/dl | 54 ± 14 |
Very-low-density lipoprotein | |
mmol/L | 0.6 ± 0.3 |
mg/dl | 21 ± 13 |
Total cholesterol/high-density lipoprotein ratio | 6 ± 2.1 |
Triglycerides (mmol/L) | 1.2 ± 0.6 |
Urate (mmol/L) | 298 ± 76 |
Creatinine (μmol/L) | 82 ± 13.4 |
Apolipoprotein B-100 (mmol/L) | 1.7 ± 0.4 |
Lipoprotein (a) (mmol/L) | 376 ± 457 |
Erythrocyte sedimentation rate (mm/1 h) | 15 ± 10.5 |
Control Group | HeFH Group | p Value | |
---|---|---|---|
Agatston score | 46 (45-76) | 204.2 (35-450) | 0.002 |
Coronary computed tomographic angiographic findings | |||
Normal | 62% (44/70) | 50% (25/50) | 0.041 |
Nonobstructive lesions | 27% (19/70) | 22% (11/50) | 0.314 |
Obstructive lesions | 5% (4/70) | 26% (13/50) | 0.032 |
Not conclusive | 4% (3/70) | 2% (1/50) | 0.775 |
Logistic regression analysis was performed to determine whether any demographic, clinical, genetic, or analytical variable was related to CAD detected by CCTA ( Table 4 ). Age, null allele LDLR mutations, and low high-density lipoprotein blood levels at diagnosis showed a statistically significant association with CAD (p <0.05 bilateral). In both genders, the probability of having CAD increased about 11% per year of age, although male patients had a sixfold probability of CAD compared to female patients (odds ratio 6.6). Otherwise, those who did not have family history of ischemic heart disease had an 80% chance of not having CAD.
Variable | Bilateral Statistical Significance (p Value) | |
---|---|---|
Univariate Analysis | Multivariate Analysis (Logistic Regression) | |
Age | 0.01 | 0.030 |
Gender | 0.256 | 0.159 |
Smoker | 0.535 | 0.558 |
Hypertension | 0.497 | 0.999 |
Diabetes mellitus | — | 0.527 |
Vasculopathy | — | 0.572 |
Xanthomas | 0.488 | 0.414 |
Xanthelasmas | 1.000 | 0.856 |
Corneal arch | 0.246 | 0.999 |
Body mass index | 0.434 | 0.168 |
Total cholesterol | 0.433 | 0.249 |
Low-density lipoprotein | 0.467 | 0.257 |
High-density lipoprotein | 0.050 | 0.048 |
Very-low-density lipoprotein | 0.353 | 0.247 |
Triglycerides | 0.365 | 0.337 |
Total cholesterol/high-density lipoprotein ratio | 0.193 | 0.123 |
Apolipoprotein B-100 | 0.844 | 0.915 |
Lipoprotein (a) | 0.789 | 0.773 |
Hyperuricemia | 1.000 | 0.858 |
Agatston score | 0.013 | 0.113 |
Null allele low-density lipoprotein receptor mutations | 0.049 | 0.019 |
We performed a second analytical determination 12 to 24 months after diagnosis, with optimal lipid-lowering therapy, to determine whether any difference was present in the treatment response to differentiate patients with or without subclinical CAD ( Figure 1 in Supplementary Data section ). The total cholesterol, high-density lipoprotein, low-density lipoprotein, lipoprotein (a), ApoB-100, and total cholesterol/high-density lipoprotein ratio decreased equally in both groups without significant differences. Only very-low-density lipoprotein, triglycerides, and urate showed a different trend between groups, although these differences did not reach statistical significance (p = 0.3, p = 0.13, and p = 0.3, respectively).
As previous studies have reported, our subjects with more severe types of LDLR mutations (null alleles) had a worse response to lipid-lowering treatment than subjects with defective mutations ( Table 5 ).
Variable | Defective Alleles | Null Alleles | p Value |
---|---|---|---|
Total cholesterol (mg/dl) | |||
Before treatment | 310.2 ± 96.1 | 342 ± 73 | 0.16 |
After treatment | 191.5 ± 30.8 | 252.4 ± 79.2 | 0.01 ∗ |
Low-density lipoprotein (mg/dl) | |||
Before treatment | 241.8 ± 99 | 269.8 ± 67.3 | 0.21 |
After treatment | 106.42 ± 24.1 | 150.6 ± 66.6 | 0.01 ∗ |
High-density lipoprotein (mg/dl) | |||
Before treatment | 47 ± 10.9 | 50.6 ± 15.6 | 0.48 |
After treatment | 48.6 ± 9.8 | 49.3 ± 11.2 | 0.88 |
Very-low-density lipoprotein (mg/dl) | |||
Before treatment | 18.4 ± 11.2 | 18 ± 7.8 | 0.71 |
After treatment | |||
16.3 ± 9.8 | 18 ± 9.9 | 0.41 | |
Triglycerides (mmol/L) | |||
Before treatment | 1.14 ± 0.65 | 1.15 ± 0.53 | 0.65 |
After treatment | 0.97 ± 0.4 | 1.06 ± 0.95 | 0.43 |
Apolipoprotein B-100 (mmol/L) | |||
Before treatment | 1.83 ± 0.33 | 1.65 ± 0.51 | 0.52 |
After treatment | 1.63 ± 0.33 | 1.66 ± 0.41 | 0.94 |
Lipoprotein (a) (mmol/L) | |||
Before treatment | 449.7 ± 622.9 | 412.75 ± 474.2 | 0.38 |
After treatment | 77.23 ± 10.1 | 97.2 ± 30.43 | 0.07 |
Total cholesterol/high-density lipoprotein ratio | |||
Before treatment | 5.9 ± 2.59 | 6.13 ± 2.31 | 0.72 |
After treatment | 3.65 ± 0.85 | 4.58 ± 1.78 | 0.18 |
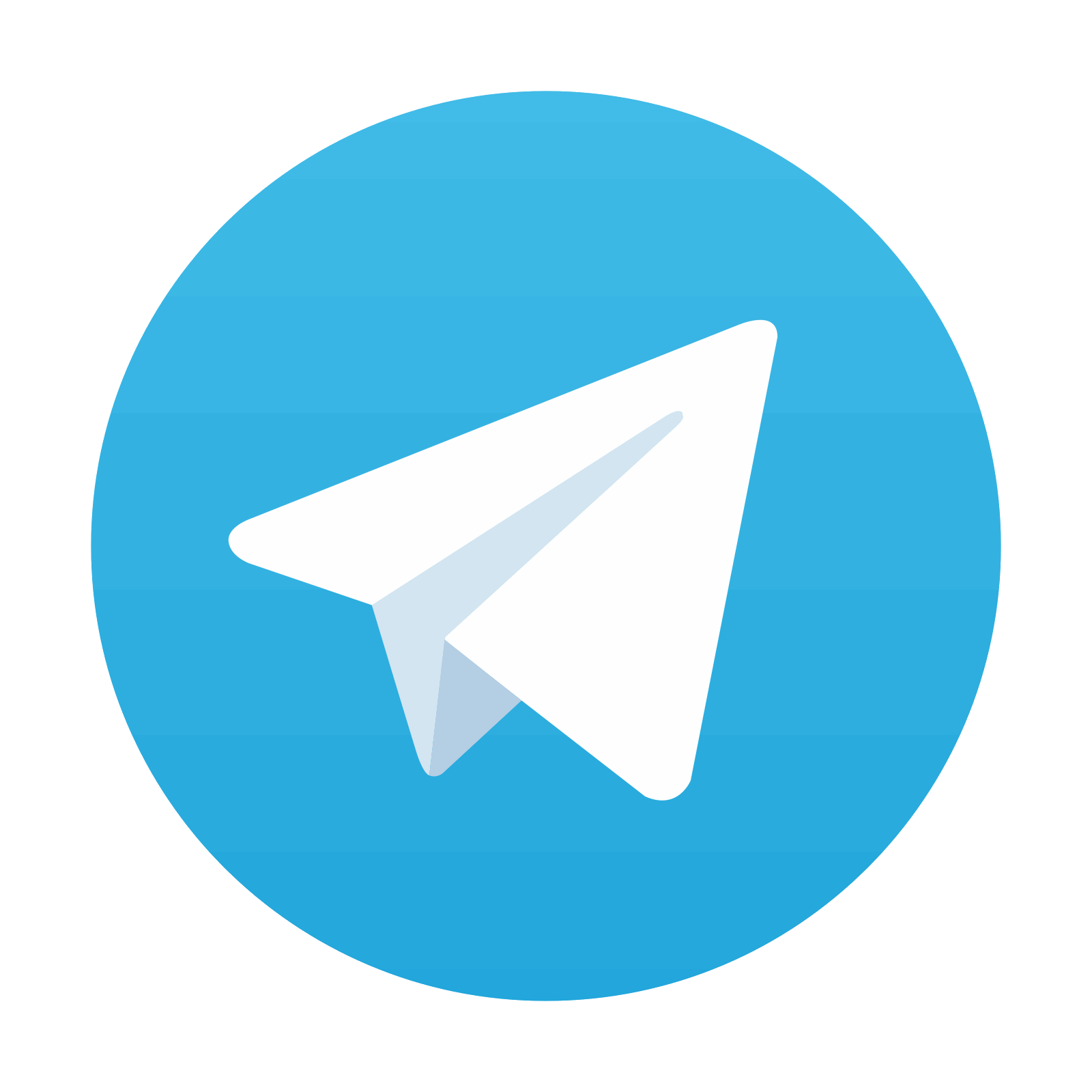
Stay updated, free articles. Join our Telegram channel

Full access? Get Clinical Tree
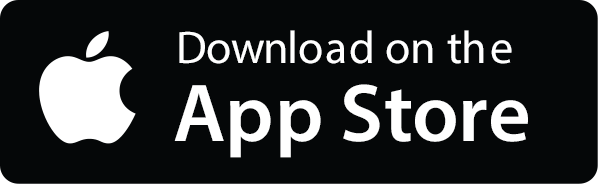
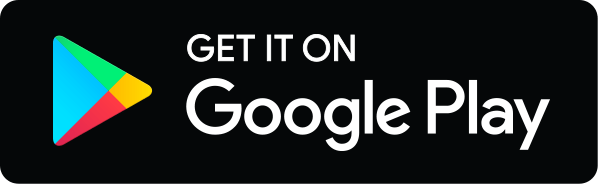
