Coronary artery calcium (CAC) scoring and cardiac computed tomographic angiography (CCTA) are comparatively new noninvasive imaging modalities that have experienced a rapid accumulation of scientific evidence for their clinical utility in the diagnosis, prognosis, and therapeutic planning for coronary heart disease. This technology offers a wide range of clinical applications and has value for a diverse range of patients.
Quantitation of coronary artery calcification by noncontrast cardiac computed tomography (CCT) for CAC scoring predicts future cardiovascular events in asymptomatic patients beyond what is conferred by assessment using traditional clinical risk-score calculators. The evaluation of coronary artery calcification has been widely accepted by the medical community as a powerful noninvasive screening tool due to the fact that atherosclerosis remains the only pathology known to be associated with coronary calcification (Fig. 28-1).1 Furthermore, the correlation between the degree of vessel calcification and the overall atherosclerotic burden is well established, both through histopathologic determination and invasive imaging modalities such as intravascular ultrasound imaging.2–5
CAC quantification may be performed by noncontrast electron beam computed tomography (EBCT) or multidetector row computed tomography (MDCT) scanners using electrocardiographic (ECG) gating. The standardized CAC protocol calls for axial imaging with prospective ECG triggering, with tube voltage of 120 kVp and tube current set at 120 to 150 milliampere-seconds (mAs), with the field of view limited to the heart and lungs using a scan length defined by the carina and the base of the heart. CAC scoring requires only a few seconds of scanning time and the overall study may be performed in 10 to 15 minutes. CAC scoring generally remains a screening tool; as such, patients selected for CAC evaluation with MDCT scanners should have minimal radiation exposure. The use of prospective ECG triggering enables performance of CAC scoring with very low-radiation doses that are less than the environmental exposure to radiation due to background radon exposure for an individual living at sea level for a year. The dose–length product (DLP) and effective radiation dose (E) should be maintained at less than 200 mGy × cm and 3.0 mSv, respectively.6 Performed within guideline-suggested parameters, imaging results in a total radiation exposure that should average 1 to 1.5 mSv.2
The Agatston score is the most commonly used tool for CAC scoring and best supported by the literature. The Agatston score is a semi-automated tool to calculate a weighted sum of the area of coronary calcification, wherein each calcified area is multiplied by a local density factor determined by the Hounsfield unit (HU) of the calcium (0:0–129 HU; 1:130–199 HU; 2:200–299 HU, 3:300–399 HU; 4:>400 HU). There may be significant interscan variability in the Agatston score due to partial-volume effects and differences in attenuation coefficients between scanners. Other CAC scoring methods, such as the volume and mass scores, exhibit less-interscan variability, but have yet to be prognostically validated in large-scale clinical studies.2 One potential mitigating factor to the routine use of Agatston CAC scoring is the integration of coronary calcium relative to HU density into the overall score. Prior studies have observed that lower-density calcifications confer greater risk for future major adverse cardiovascular events (MACE), while higher-density calcifications may somehow be protective. In this regard, the inability to extricate the HU density from the CAC score may lessen the CAC score as calculated by the method of Agatston.7,8 Nevertheless, the Agatston CAC score has been validated for its predictive ability in an array of studies, and presently serves as the prognostic “gold standard” for CAC scoring. Importantly, technological improvements in newer-generation scanners have resulted in adequate CAC score reproducibility despite the technical limitations of the Agatston method.9 CAC scoring has also been evaluated as an “add-on” for other imaging methods. In particular, single-photon emission computed tomography (SPECT) and positron emission tomography (PET) often use very low-dose CT scanning for attenuation correction. Despite these images not being acquired specifically for CAC scores, they nevertheless enable the quantification of CAC scores with generally high fidelity.10 CAC scores are often similarly classified across clinical studies, with the following categorizations generally accepted for absent (CAC = 0 Agatston units), mild (CAC = 1–100 Agatston units), moderate (CAC = 101–400 Agatston units), and severe (CAC >400 Agatston units) coronary calcification. Based on the population-based multi-ethnic study of atherosclerosis (MESA), a CAC score of >300 Agatston units can also be considered to be severe.11–13
As a noncontrast study, CAC is unable to visualize noncalcified plaque or luminal stenosis encroachment by atherosclerotic plaque, and is thus unsuited for diagnosis of obstructive coronary artery disease (CAD). As a marker of overall atherosclerosis, severely elevated CAC score is associated with a higher likelihood of obstructive CAD among patients undergoing evaluation for stable chest pain. As an example, in a recent study evaluating the MESA population cohort of 6814 individuals, the mean CAC mass score for patients with nonobstructive (<50% stenosis), moderately obstructive (50–70% stenosis), and obstructive (>70% stenosis) CAD in the left anterior descending (LAD) artery were 105, 175, and 302 mg. A proportional association of total Agatston CAC score was observed for the number of obstructive stenosis in coronary arteries.14 The negative predictive value (NPV) of the CAC score for the exclusion of obstructive CAD is excellent, as a CAC score of 0 carries an NPV of 99% for greater than 70% stenosis.15 In distinction, for symptomatic patients, a negligible CAC score does not exclude the presence of obstructive stenosis. It has been shown that up to a fifth of patients referred for angiography had obstructive CAD despite having no coronary calcium detected by noninvasive imaging.16,17
CAC scores are population dependent and thus vary with age, gender, race, ethnicity, and socioeconomic status.7,18,19 Yet in the MESA study, a similar overall relationship was observed for CAC in all populations, with CAC consistently associated with adverse clinical outcomes. This study, designed to investigate the prevalence, correlates and progression of subclinical cardiovascular disease in white, black, Hispanic, and Asian Americans of Chinese descent, demonstrated the consistent prognostic value of CAC score across each subgroup examined.20 Agatston CAC scores of 0 predicted very low rates of downstream major coronary events, with a 4.6-year follow-up of 0.2%. In contrast, those individuals exhibiting a CAC 1 to 100, 101 to 300, and >300 experienced major coronary event rates of 1.4%, 3.2%, and 3.8%; these correlated to increased hazard ratios of 3.89, 7.08, and 6.84. Importantly, the prognostic utility of CAC scores is an independent factor incremental to traditional cardiovascular risk factors, and can correctly risk-stratify, discriminate, and reclassify patients with CAD. Indeed, the net reclassification improvement (NRI) based on CAC can be used to reclassify individuals to low-risk categories as well as high-risk categories. Individuals with CAC <100 can be reclassified as low risk 21.7% of the time, while individuals with CAC ≥400 can be reclassified as high risk 30.6% of the time.21
On the basis of the large body of evidence supporting its clinical utility, CAC scoring has been adopted as a useful risk-stratification tool in preventive cardiology guidelines. Notably, CAC scoring is considered useful in the following patient populations: (1) asymptomatic adults with intermediate-cardiovascular risk (class IIa); (2) asymptomatic adults with low–intermediate risk (class IIb); (3) asymptomatic diabetics age >40 (class IIa). In the recent risk-stratification guidelines proposed by the ACCF/American Heart Association (AHA), it is recommended that CAC scoring may be considered in the decision to prescribe statins for patients with an estimated cardiovascular event rate between 5% and 7.5%, calculated using the pooled-cohort equations, while patient groups with either higher- or lower-estimated event rates are recommended for treatment strategies regardless of CAC scores.22–24 Such recommendations have also been echoed by the 2013 appropriate use criteria (AUC), which state that it may be appropriate to perform a CAC score in the evaluation of asymptomatic patients determined to be at intermediate-to-high risk for CAD.25
CAC scoring has also proven to be useful in select populations at a particularly high risk of adverse cardiovascular events. As an example, in asymptomatic diabetics, it has recently been shown that a CAC = 0 carries a very favorable 5-year prognosis, similar to that of nondiabetics. However, the mortality risk at 15 years of follow-up increases dramatically regardless of the initial prognosis, suggesting a limited “warranty period” for this high-risk patient population.26
Finally, while CAC progression has correlated with traditional cardiovascular risk factors, serial measurements of CAC are currently not recommended because the clinical understanding of progression of coronary calcification is not clearly understood, nor is it apparent what the effect of pharmacologic or behavioral modification of cardiovascular risk is on CAC progression.12 In addition, such measurements involve increased costs and exposure (albeit small) to radiation, with unclear management implications. Among asymptomatic patients, the “warranty period” of a CAC = 0 transitioning to a CAC >0 appears to be approximately 4 years, with the clinical “warranty period” of event-free survival extending to nearly 15 years.27
Computed tomography (CT) has been in clinical use since the 1970s, when Sir Godfrey Hounsfield utilized x-ray CT to construct axial images of the human body.28 During the ensuing decade, CT was employed in evaluating noncardiac organs, given the need for greater temporal, spatial, and contrast resolution in imaging a moving organ. In 1981, ECG-gated cardiac CT was first utilized to directly image the myocardium and the chambers of the heart and in 1997, coronary luminal visualization was achieved using ECG-gated contrast-enhanced electron beam CT (EBCT).29,30 Since the introduction of 64-detector row CT scanners in 2005, coronary CT angiography (CCTA) has been established as the definitive noninvasive imaging modality for visualization of native coronary arteries and coronary artery bypass grafts.
Cardiac CT images have traditionally been acquired through either EBCT or multidetector (MDCT) scanners. The clinical application of EBCT is limited to the noninvasive detection and evaluation of CAC. EBCT scanners have low spatial resolution, are expensive, and consequently are no longer used.31 MDCT scanners, on the other hand, allow for superior imaging of the cardiovascular system through improved spatial resolution, as well as the ability to acquire volumetric data that now allow for image acquisition of the entire heart within 1 second. While inferior to the temporal resolution of EBCT scanners, MDCT methods have improved the temporal resolution, with the most contemporary scanners achieving resolutions as low as 70 milliseconds. Technological advances for improving temporal resolution are achieved with faster gantry rotation as well as different methods of image acquisition that do not require full helical imaging. The pitch, defined as the table movement in one 360-degree gantry rotation divided by the slice (or beam) width, can also be increased to this effect (e.g., fast-pitch helical modes). Furthermore, spatial resolution has been improved using different detector elements to achieve sub-millimeter isotropic voxels. In addition, whole-volume coverage of MDCT using 320-detector row scanners for 1-second cardiac imaging minimizes misregistration artifacts that are common in 64-detector row MDCT scanners.
CCTA is well tolerated by most patients, and generally requires minimal preparation. It is recommended that patients abstain from caffeinated beverages for at least 12 hours prior to imaging in order to prevent a rise in heart rate and avoid diuresis. Some institutions also recommend that patients undergoing CCTA abstain from food for 2 or more hours prior to imaging due to the risk of contrast-induced nausea, vomiting, and subsequent aspiration. While patients should continue to use their routinely prescribed medications on the day of examination, those on metformin and phosphodiesterase type 5 (PDE5) inhibitors are instructed to abstain from them due to their unique side-effect profile. Though rare, there is concern for the development of lactic acidosis as a result of contrast-induced nephropathy with metformin use. Likewise, PDE5 inhibitors are contraindicated due to the risk of hypotension when combined with nitroglycerin. Indeed, in the hours prior to imaging, patients typically receive an oral beta-blocker, such as metoprolol, to reduce the heart rate. Administration of this agent is then followed by use of sublingual nitroglycerin in the minutes immediately preceding initiation of the scan. While in the scanner, patients are instructed to hold their breath to prevent the development of image artifact. Nevertheless, despite such measures, imaging quality can be limited as a result of significant heart rate variability or ectopy (such as atrial fibrillation or the presence of premature contractions). In addition, the presence of advanced renal insufficiency or contrast intolerance/allergy limits the ability to administer contrast and reduces the quality of the acquired images.
The primary application of MDCT cardiac imaging involves characterization of coronary anatomy for assessment of stenosis severity as well as atherosclerosis extent, severity, and location (Fig. 28-2). These findings are useful for aiding the prediction of future adverse cardiovascular events.32,33
Figure 28-2
Coronary computed tomography angiography use in delineation of atherosclerotic disease burden. Curved multiplanar reformatted images demonstrating: normal coronary artery (A), mildly stenotic calcified plaque (B), moderately stenotic partially calcified plaque (C), and (D), a noncalcified occlusive plaque with bridging collaterals (red arrow).

Based on the recommendations of the 2008 AHA scientific statement on noninvasive coronary artery imaging, the 2010 Expert Consensus Document on CCTA and the 2012 American College of Cardiology (ACC)/AHA stable ischemic heart disease (SIHD) guidelines34–36:
CCTA should not be used to screen for CAD in the absence of signs or symptoms suggestive of CAD.
CCTA is not recommended in patients with either low pretest likelihood of CAD (due to concerns of radiation exposure) or a high pretest likelihood of CAD (such patients are likely to require invasive coronary angiography anyway).
CCTA is a reasonable option for patients with intermediate pretest probability of CAD who are unable to perform other noninvasive modalities (exercise-stress test, nuclear perfusion scar, or echocardiography).
CCTA might be reasonable for symptomatic patients who are at intermediate risk for CAD after initial risk stratification.
CCTA or cardiac MRI is suggested to evaluate patients with congenital or acquired coronary anomalies.
Importantly, in a departure from prior recommendations, the 2013 appropriate use criteria (AUC) guidelines deemed CCTA appropriate for follow-up testing within 90 days in the setting of uncertain prior ECG or stress imaging study results. In addition, the recommendation for the use of CCTA in symptomatic and preoperative patients has been weakened, in accordance with the most recent perioperative and SIHD guidelines.25
ECG gating is applied in cardiac CT to synchronize image acquisition to the cardiac cycle, in order to minimize motion artifact in the beating heart. ECG gating can be performed prospectively or retrospectively (Fig. 28-3).33 Prospective ECG gating involves initiation of image acquisition at a prespecified interval during the cardiac cycle (typically diastole). As a result, the projection data are acquired for only a short part of the complete gantry rotation. Once the desired data are acquired, the table is shifted to the next bed position and the scanner acquires more projections. This cycle repeats until the entire scan length is covered. In contrast, retrospective ECG gating involves continuous image acquisition throughout the cardiac cycle with significant overlap. Retrospective gating typically involves low scan pitch, defined as the relationship of the scan coverage to the table speed, which ensures contiguous sampling with the absence of gaps. In addition, retrospective gating permits image reconstruction at multiple points in the cardiac cycle, as opposed to the fixed point of acquisition used with prospective gating protocols.
Figure 28-3
ECG-gating techniques. ECG-gated image acquisition can be performed prospectively (A,C) or retrospectively (D,E). Prospective gating involves data acquisition during a specified portion of the cardiac cycle (A), with the ability to narrow (B) or widen (C) the acquisition interval. Retrospective gating involves data acquisition throughout the cardiac cycle (D), with the existence of techniques to reduce radiation exposure (E).

The number of detector rows is an important aspect of image acquisition, since an increase in the number of detectors allows for a large portion of the heart to be covered per gantry rotation. For example, each detector row acquires data simultaneously in the z direction with each gantry rotation in multidetector CT scanners. In a 64-slice detector CT with each detector measuring 0.625 mm in width, a typical cardiac region ranging between 120 and 150 mm in length can be covered in three to four gantry rotations (with each gantry rotation scanning approximately 40 mm). As a result, less acquisition time is required, decreasing the time required for breath holding and minimizing motion artifacts (which are major limitations in hospitalized patients).
The rise of cardiac CT during a period of dramatic increase in radiation exposure from medical procedures, coupled with an increased recognition of the associated stochastic and deterministic effects of radiation, has led to intense scrutiny of the radiation burden associated with this new technology (see Chapter 7). For example, a chest x-ray results in an effective dose exposure of 0.1 mSv, while CCTA results in an effective exposure of 3 to 4 mSv (and can be as high as 8–22 mSv in retrospectively gated scans) and 8 to 12 mSv for technetium myocardial perfusion scans. The 2011 Society of Cardiovascular Computed Tomography (SCCT) guidelines provide recommendations regarding interpretation of radiation dose indices and predictors of risk, appropriate use of scanner acquisition modes and settings (Fig. 28-3), development of algorithms for dose optimization, and establishment of procedures for dose monitoring.37 Some of the commonly adopted techniques for radiation dose reduction include38:
Prospectively gated imaging (estimated dose reduction up to 83%) as a result of image acquisition during a short period in diastole.
ECG-gated (estimated dose reduction 30–50%) image acquisition is coupled with the cardiac cycle with high tube current applied only during diastole.
High-pitch scanning mode (estimated dose reduction up to 80%) requires dual-source scanners in order to obtain the projection data by the second detector to avoid gaps due to rapid table movement.
Low tube voltage (kVp) (estimated dose reduction up to 30%) results in decreased photon emission. Image quality is maintained due to differences in contrast level. Structures with high atomic number maintain contrast difference over those with low atomic number.
Anatomy-based tube-current modulation (estimated dose reduction 20–60%) involves adjustment of the tube current for each projection angle according to patients’ anatomic structures.
Iterative reconstruction algorithms (estimated dose reduction up to 40%) employ computational techniques that enable improved image quality at lower-radiation doses while reducing image noise.
The use of contrast media in CCTA aims for maximal opacification of the coronary arteries and the left ventricle. The determination of the volume of contrast to be administered is an important aspect of the examination. Initially, a test bolus of contrast is followed by an intravenous saline injection at a prespecified rate. Sequential axial scans at the proximal aorta are obtained to determine timing of peak opacification. The total contrast volume during actual image acquisition is calculated by adding the time required for peak aortic opacification to the time required to cover the scan length, multiplied by the infusion rate. As a result, the need for contrast media use for coronary artery opacification limits the usage of CCTA in patients with advanced renal impairment.
Since the inception of 64-detector row MDCT scanners, debate has centered on CCTA’s ability to determine coronary stenosis severity as compared to an invasive angiographic “gold standard.” Three prospective multicenter studies have addressed this question (Table 28-1). In the first published ACCURACY study, 230 individuals with suspected but without known CAD underwent both CCTA and invasive angiography. Per-patient sensitivity and specificity to detect and exclude obstructive CAD were 95% and 83%.39 This compared similarly to the study by Meijboom et al.40 who evaluated 360 symptomatic patients with both acute and stable chest pain who underwent CCTA and invasive angiography. With more than two-thirds of patients exhibiting a coronary stenosis ≥50%, the sensitivity and specificity of CCTA versus invasive angiography was 99% and 64% respectively. In contrast, Miller et al.41 evaluated a mixed population of patients with and without known CAD and observed a lower sensitivity of 85% with a higher specificity of 90%.
Trial | Year | Sample Size | Patient Population | Variable Evaluated | Accuracy |
---|---|---|---|---|---|
Leschka et al.42 Eur Heart J | 2005 | 67 | Patients with suspected coronary artery disease or prior to coronary artery bypass | ≥50% stenosis | Per Segment Analysis Se 94%, Sp 97%, PPV 87%, NPV 99% |
Raff et al.43 J Am Coll Cardiol | 2005 | 70 | Patients scheduled to undergo coronary angiography | ≥50% stenosis | Per Patient Analysis Se 95%, Sp 90%, PPV 93%, NPV 93% Per Vessel Analysis Se 91%, Sp 92%, PPV 80%, NPV 97% |
Ehara et al.44 Circ J | 2006 | 69 | Patients with suspected or proven coronary artery disease, or prior stenting | ≥50% stenosis | Per Patient Analysis Se 98%, Sp 86%, PPV 98%, NPV 86% Per Vessel Analysis Se 90%, Sp 94%, PPV 89%, NPV 95% |
Schujif et al.45 Am J Cardiol | 2006 | 60 | Patients scheduled to undergo invasive angiography | ≥50% stenosis | Per Patient Analysis Se 94%, Sp 97%, PPV 97%, NPV 93% Per Vessel Analysis Se 87%, Sp 96%, PPV 87%, NPV 96% |
ACCURACY trial39 J Am Coll Cardiol | 2008 | 230 | No history of coronary artery disease | ≥50% or ≥70% stenosis | ≥50% Stenosis Se 95%, Sp 83%, PPV 64%, NPV 99% ≥70% Stenosis Se 94%, Sp 83%, PPV 48%, NPV 99% |
Meijboom et al.40 J Am Coll Cardiol | 2008 | 360 | Stable or unstable anginal syndrome | ≥50% stenosis | Per Patient Analysis Se 99%, Sp 64%, PPV 86%, NPV 97% Per Vessel Analysis Se 95%, Sp 77%, PPV 59%, NPV 98% |
Miller et al.41 N Engl J Med | 2008 | 291 | Suspected symptomatic coronary artery disease | ≥50% stenosis | Per Patient Analysis Se 85%, Sp 90%, PPV 91%, NPV 83% Per Vessel Analysis Se 75%, Sp 93%, PPV 82%, NPV 89% |
Brodoefel et al.46 Eur J Radiol | 2008 | 102 | Known or suspected coronary artery disease | ≥50% stenosis | Per Segment Analysis Se 91%, Sp 99%, PPV 95%, NPV 98% |
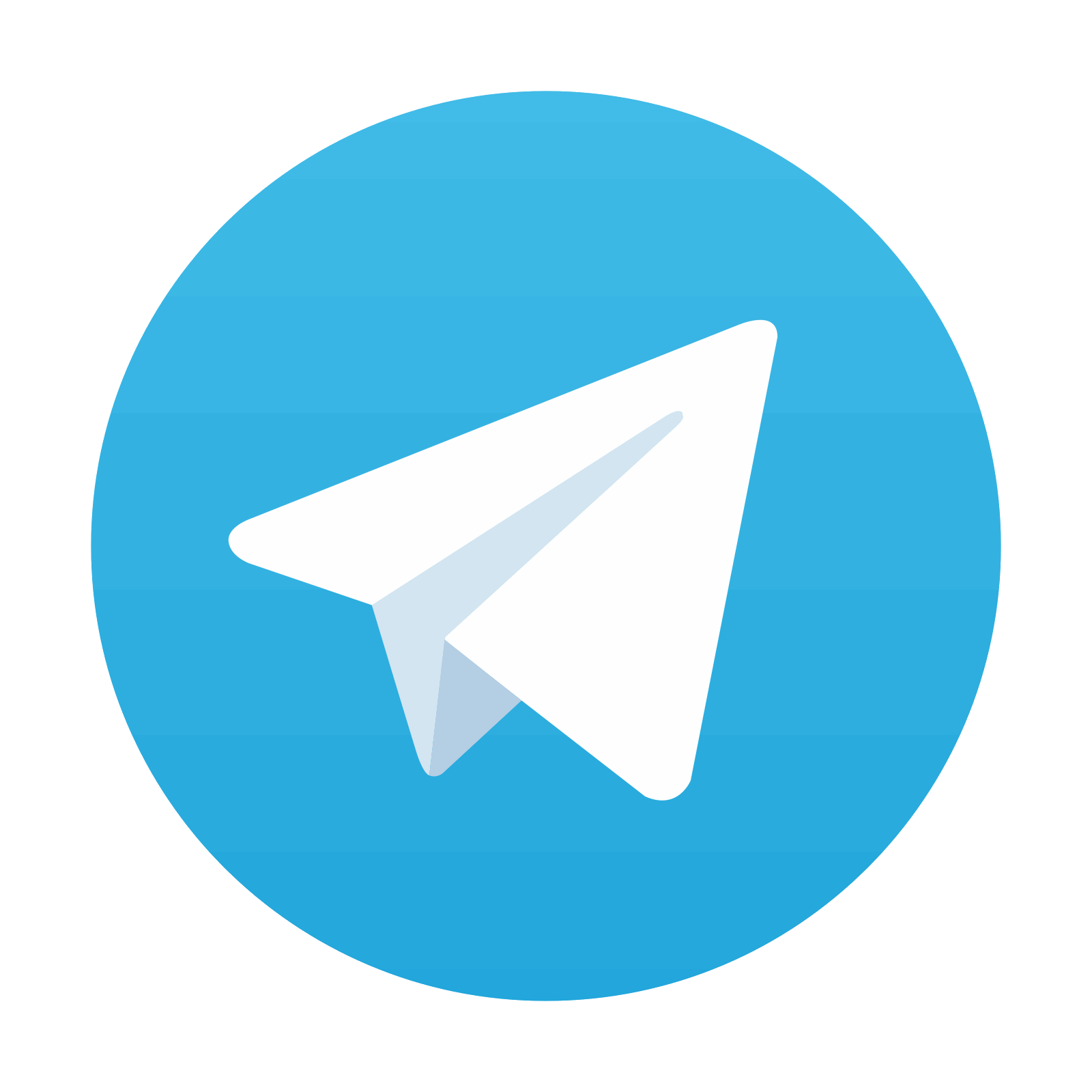
Stay updated, free articles. Join our Telegram channel

Full access? Get Clinical Tree
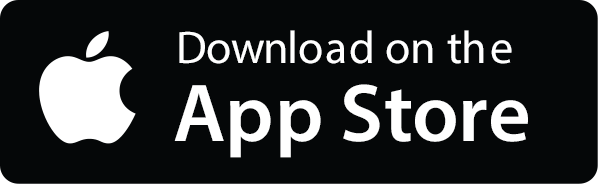
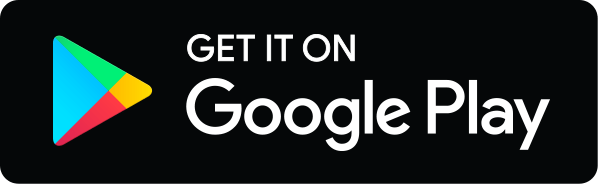
