Coronary atherectomy continues to be an important tool for percutaneous coronary intervention (PCI), particularly as the population ages and more patients present with complex calcified coronary artery disease. Early studies using rotational and laser atherectomy failed to demonstrate a clear advantage over angioplasty alone, and as such, atherectomy of straightforward atherosclerotic disease is not commonly used.1 With the very low restenosis rates of second-generation drug-eluting stents (DESs) in many lesion types, contemporary practice is to use atherectomy primarily as adjunctive therapy for plaque modification of calcific lesions that cannot be crossed with devices or dilated with balloons to enable DES delivery.
Utilization of atherectomy composes approximately 3% of PCIs in the United States1 and between 0.8% and 3.1% worldwide (Table 34-1).2 Yet, as the population ages, more PCIs are performed in higher risk patients with more complex coronary artery disease,3 and in a recent evaluation of patients undergoing PCI, 33% were found to have moderate to severe coronary calcification (Fig. 34-1).4 Furthermore, the extent of vessel calcification is often underappreciated fluoroscopically. In one study, significant calcification seen by intravascular ultrasound (IVUS) was seen only 50% of the time fluoroscopically,5 which is often the cause for “rota-regret” when a stent cannot be delivered or dilated.
FIGURE 34-1
Prevalence of moderate to severe calcification in 1800 patients undergoing percutaneous coronary intervention (COMPARE study).4 ACC, American College of Cardiology; AHA, American Heart Association; CTO, chronic total occlusion.

Vessel calcification remains a major independent predictor of PCI failure6 and adverse outcomes (Fig. 34-2),7,8 and atherectomy is a critical component to improve PCI success in these situations. Some studies are already starting to show an increasing trend in the number of operators and centers performing atherectomy.9
FIGURE 34-2
Increasing risk associated with degree of lesion calcification among patients being treated with percutaneous coronary intervention (HORIZONS-AMI and ACUITY studies).7 P < .05 for all comparisons. MACE, major adverse cardiac events; MI, myocardial infarction; ST, stent thrombosis; TLR, target lesion revascularization.

Rotational atherectomy is the most widely used form of atherectomy, although utilization of laser and orbital atherectomy has been increasing. The majority of clinical data regarding coronary atherectomy is based on evaluation of the Rotablator device (Boston Scientific, Marlborough, MA). As such, this chapter will focus primarily on rotational atherectomy, with more brief discussion of the potential utility of other atherectomy devices.
The early experience with coronary atherectomy focused on plaque reduction and lesion debulking, given the residual stenosis and need for repeat revascularization in an era in which PCI was limited to balloon angioplasty.
Several devices were developed and approved for use, but clinical trials found minimal benefit and potentially harm compared to angioplasty. Directional coronary atherectomy and laser atherectomy have fallen out of favor given their association with coronary perforations and similar rates of restenosis and adverse events compared with patients undergoing angioplasty alone.10
In the coronary stenting era, in which restenosis rates with DES are less than 3% annually,11 atherectomy is used primarily for plaque modification and lesion preparation rather than debulking. Rotational atherectomy has remained a useful tool for its ability to preferentially ablate calcific and fibrotic disease to help facilitate stent delivery,12 avoiding the barotrauma caused by repeated high-pressure balloon inflations that can lead to vessel dissection or perforation.13,14
Furthermore, forceful advancement of DES through narrow, calcified lesions may cause stent polymer damage, which could be avoided with proper lesion preparation using atherectomy.15 In the ROTAXUS (Rotational Atherectomy Prior to Taxus Stent Treatment for Complex Native Coronary Artery Disease) study, among patients who underwent an angioplasty-stenting strategy, stent loss was observed in 2.5% of patients, and 12.5% of these patients ultimately crossed over to atherectomy, whereas no stent loss was observed among patients randomized to the atherectomy strategy.12
The ROTATE Multicenter Registry demonstrated that patients undergoing planned rotational atherectomy had fewer balloon inflations, less radiation exposure, lower contrast volumes, and shorter procedure times than those undergoing provisional atherectomy for device delivery failure or balloon expansion failure (Fig. 34-3).16
FIGURE 34-3
Differences in procedural outcomes between planned and provisional use of rotational atherectomy (ROTATE Multicenter Registry).16 P < .05 for all comparisons. RA, rotational atherectomy.

In addition to this, atherectomy would potentially modify a calcified lesion to allow optimal stent expansion and improve lumen gain.12,17 This may have particular benefits for PCI with bioresorbable scaffolds that may have lower radial strength and greater recoil than metal stents that demand more complete vessel preparation to allow stent delivery and more complete apposition.18,19 Although the use of atherectomy for debulking atherosclerotic tissue has fallen out of favor in the era of DES, plaque reduction remains useful in specific situations such as bifurcations or ostial disease, reducing disease at the carina to avoid geographic miss, and plaque shift.20-22 The list of potential benefits of atherectomy to optimize PCI are listed in Table 34-2.
Potential Benefits of Atherectomy to Optimize PCI |
|
The Rotablator rotational atherectomy system was developed by David C. Auth, PhD, PE, at the University of Washington over a period of 13 years. After years of experimentation and animal studies, on January 6, 1988, Fourrier et al23 performed the first procedure in a human coronary artery. At the same time, Zacca and colleagues performed the procedure in the peripheral vasculature in humans. Since then, multiple clinical trials and registries have demonstrated the efficacy of rotational atherectomy, which has become the most commonly used coronary atherectomy device worldwide.
The components of the Rotablator rotational atherectomy system include the burr, drive shaft, turbine, and console (Fig. 34-4).
The Rotablator burr is a nickel-plated brass ellipse coated with diamond crystals between 20 and 30 μm in size on the leading edge, on average extruding only 5 μm from the surface, forming an abrasive sanding surface. There are no diamond chips on the trailing edge, so atherectomy is only achieved with forward motion of the burr. For the coronary arteries, burrs are available in sizes of 1.25, 1.5, 1.75, 2.0, 2.15, 2.25, 2.38, and 2.5 mm. The inner diameter of the guide catheter must be at least 0.01 mm (0.004 in) greater than the burr size to provide adequate clearance for device advancement and withdrawal. The later section on guide catheters describes this in greater detail.
The burr is bonded to a drive shaft housed in a 4.3-Fr, 135-cm-length Teflon sheath and connected to a turbine within the advancer. Potential injury to the arterial wall (by the spinning drive shaft) is prevented by the Teflon sheath, which also acts as a conduit, delivering flush at 7 to 13 mL/min when activated.
Compressed nitrogen powers a turbine within the advancer that rotates the shaft and burr at speeds of 150,000 to 200,000 rpm. The turbine is controlled by the console and activated by a foot pedal. The advancer has a knob that controls the movement of the burr during atherectomy. The knob can be locked down to avoid movement of the burr during advancement through the coronary guide. The back end of the advancer has a brake that locks the advancer onto the wire and prevents the coaxial guide wire from spinning along with the burr. The break is released by pressing the black button on the end of the advancer, which needs to be released when withdrawing the device.
The console regulates the flow of compressed air, which controls the turbine speed. A fiberoptic light probe monitors the rotational speed and displays it on the console tachometer. Depressing the foot pedal initiates rotation of the burr and activates the breaking system. The button on the right of the foot pedal toggles between the rotation used for atherectomy and DynaGlide, which rotates the burr at a constant 30,000 rpm to prevent stalls. DynaGlide is used for withdrawing the system through the guide and helping to disentangle an entrapped burr.
The high-speed rotations of the Rotablator are effective in increasing lumen diameter through plaque ablation but can also have deleterious effects that may weaken the vessel adventitia, leading to perforations and vessel aneurysms, or cause microcavitations, hematologic effects, and embolization of microparticulate debris that can lead to no reflow.
The Rotablator system is analogous to a low-powered rotary sander. High-speed rotational atherectomy effectively ablates calcified, inelastic atherosclerotic tissue while sparing healthy, elastic tissue, leading to lumen enlargement and reduction of cross-sectional atherosclerotic plaque area. There is also minimal trauma to normal “healthy” tissue. Data from human and animal studies confirm these findings.23-25
The 2 principles that govern the operation and effectiveness of the Rotablator system are differential cutting and orthogonal displacement of friction.
Differential cutting is the ability to selectively ablate one material while preserving the integrity of the other, based on differences in the composition and texture of the substrate. The elasticity of the normal tissue deflects the ablative surface, whereas the inelastic properties of diseased tissue engage the cutting surface. A common analogy to differential cutting is shaving. Whiskers are relatively inelastic compared to skin, and a razor will preferentially cut these while sparing the skin. The atherectomy burr tends to spare healthy, elastic tissue, while calcium, fibrous tissue, fatty deposits, and intimal hyperplasia (restenotic tissue) increase the inelastic properties of vascular tissue, making it susceptible to ablation by the cutting edges of the atherectomy burr.
Orthogonal displacement of friction is essential in allowing easy passage of the burr through diseased and tortuous vascular segments. The sliding motion and high rotational speeds virtually eliminate the longitudinal friction vector, allowing for unhindered advancement and withdrawal of the burr. This principle is similar to a corkscrew, in which the twisting motion reduces the friction on the surfaces and facilitates movement of the corkscrew. The faster it is turned, the easier it is to remove it.
These 2 basic principles allow for the burr to effectively ablate diseased and atherosclerotic tissue, with lower risk of harming normal tissue. This was confirmed with early animal studies using cholesterol-fed New Zealand white rabbits. After treatment, the minimum lumen diameter (MLD) was reduced from 81% ± 9% to 38% ± 22% (P < .001, paired t test).26 Histologic specimens showed effective intimal plaque removal, with minimal medial injury and no medial or intimal dissections. Similar results were shown by Ahn et al27 in cadaveric human coronary arteries.
Quantitative coronary angiography has been used to evaluate the efficiency of rotational atherectomy in debulking lesions. Early studies using quantitative coronary angiography in 109 patients showed that a predictable MLD can be achieved across the range of burr sizes. Expressing MLD as a burr ratio (MLD/burr size), the Rotablator achieved immediate results of 72% ± 19% of the burr selected (ie, a 2.0-mm burr predicted an MLD of approximately 1.4 mm). Mintz et al25 confirmed this using IVUS. There was minimal injury to the media and adventitia of adjacent tissue. Measured 24 hours later, the burr ratio increased to 84% ± 15%.28
Guide wire bias, however, preferentially orients the burr toward either the inner or outer curvature of an angulated lesion and causes unequal force to be exerted on one region of the vessel wall, resulting in a much larger, eccentric lumen. The Rotablator burr creates a force vector that not only ablates the vessel media and creates an elliptical lumen,29 but can also form deep vessel dissections or “rota-gutters,”30 aneurysms,31 and perforations32 (Fig. 34-5).
FIGURE 34-5
Formation of a deep vascular dissection by rotational atherectomy. A. Angiogram showing severe calcification along an angulated lesion (white solid arrow). There is a calcified plaque before atherectomy with the wire in contact with the vessel wall (I), and plaque modification after the debulking intervention with a channel formation (II). The diameter of the channel (solid white double arrow) is exactly the same diameter of the 1.25-mm burr that was used. After stent placement (III), some segments still showed the presence of the channel (asterisk) behind the struts (white dashed arrow). (Used with permission from Attizzani GF1, Patrício L, Bezerra HG. Optical coherence tomography assessment of calcified plaque modification after rotational atherectomy. Catheter Cardiovasc Interv. 2013;81(3):558-561.)

In some studies, sequential IVUS imaging26,28 and electron microscopy33 have shown not only plaque reduction, but also ablation of media volume and damage to the smooth muscle layer, albeit without change in the external elastic membrane diameter. Nevertheless, unequal ablation of tissue, atherectomy of calcium, and disruption of the media layer change vessel compliance in such a way that allows for device delivery and full expansion, even with minimal initial lumen gain. In more contemporary studies of atherectomy adjunctive to stenting, significant increases in lumen areas were documented despite use of primarily smaller 1.5-mm burrs.12,16,34
For these reasons, the maximal burr size should not exceed 70% of the vessel diameter, and floppy wires or guide and wire positioning techniques can be used to reduce eccentric cutting of the vessel media layer. Atherectomy of extremely angulated lesions may need to be avoided.
Thermal injury can lead to smooth muscle proliferation, increasing restenosis rates, as well as cause red blood cell aggregation and platelet activation.35-37 Increased temperatures are generated with longer, more continuous engagement of coronary lesions with the spinning burr and aggressive ablation with excessive decelerations (14,000-18,000 rpm), whereas an intermittent or oscillating “pecking” technique can limit decelerations and reduce the temperature changes.38 Minimizing thermal injury using this technique may prevent vessel trauma and smooth muscle proliferation that may increase restenosis.
The vast majority of microparticulate debris produced by rotational atherectomy is generally 2 to 10 μm and smaller, compared to red blood cells and capillaries, which are about 6 to 10 μm26,27; however, about 2% to 10% of particles are 10 to 20 μm and larger when using the larger burr sizes.27,39 A recent study of the orbital atherectomy device reported an average particulate size of 2 μm, with approximately 1% of particles being larger than 9.5 μm. Most of this debris passes through the capillaries and is cleared by the reticuloendothelial system.27 Transient perfusion defects can be observed on single-photon emission computed tomography imaging, but these appear to resolve after 48 hours and are not necessarily clinically significant.40 Other studies have shown similar defects with PCI that may not differ significantly from rotational atherectomy.41
The rapidly spinning Rotablator burr is also known to form relatively large gas bubbles dissolved in blood called microcavitations, which appear to collapse quickly. During activation of the burr, transient enhancement of echocardiographic contrast is seen in the area of the myocardium subtended by the artery, which disappears immediately after the burr rotation is stopped. Microcavitations may transiently reduce myocardial blood flow.42
Nevertheless, more significant no reflow or vessel closure during the procedure, associated with hypotension, is clinically relevant, and steps should be taken to minimize such events. It is recommended that each atherectomy run last no longer than 30 seconds to restore normal myocardial blood flow and allow time for microparticles to clear from the distal vasculature. Administration of prophylactic vasodilators or mixing verapamil and nitroglycerine with the RotaGlide lubricant may help reduce no reflow.43,44
The high rotational speed of the burr establishes a shear field and affects both erythrocytes and platelets. A study using 8 samples of porcine blood exposed to a spinning burr demonstrated evidence of platelet aggregation as measured by optical microscopy.38 The number of aggregates was affected by the speed of the burr. Larger platelet aggregates (>60 μm in diameter) were seen in all 8 samples at 180,000 rpm and in only 1 of 8 samples at 140,000 rpm. These findings support the importance of lowering the burr speed to minimize the hematologic impact of rotational atherectomy. The combination of lower rotablation speeds and the use of glycoprotein IIb/IIIa inhibitors during atherectomy has also been shown to reduce platelet aggregation45 and the incidence of periprocedural myocardial ischemia46 and infarctions in patients.47
These observations establish the basic foundation for understanding the mechanism of rotational atherectomy and provide general principals to mitigate the untoward effects of atherectomy (Table 34-3). Smaller burr/artery ratios, management of guide wire bias, and avoidance of severely angulated lesions will help avoid significant damage to the vessel media layer. Heat generation and thermal injury can be minimized by advancing the device gently and intermittently and avoiding significant decelerations. Use of smaller burrs, limiting atherectomy runs to 30 seconds, and prophylactic administration of vasodilators may reduce the impact of downstream microparticulate embolization. Platelet aggregation and other hematologic affects can be controlled by lowering the rotational speeds and the use of platelet inhibitors.
Complication | Causes | Recommendation |
---|---|---|
Perforations and aneurysms | Burr oversizing, forceful burr advancement, angulated lesions and guide wire bias causing deep fissures, and eccentric cutting of the vessel media layer |
|
Restenosis | Thermal injury predisposing to intimal hyperplasia |
|
Slow flow | Microparticulate debris and platelet aggregation |
|
Burr entrapment | Forceful advancement of the rotablation burr or atherectomy within a dissection |
|
The internal diameter of the guiding catheter should be at least 0.01 mm (0.004 in) larger than the burr. Therefore, a 5-Fr guide with an inner diameter of 1.47 mm (0.058 in) can only allow delivery of the 1.25-mm burr. A 6-Fr guide with an inner diameter of 1.80 mm (0.071 in) can accommodate the 1.25-, 1.5-, and 1.75-mm burrs. A 7-Fr guide with an inner diameter of 2.05 mm (0.081 in) can accommodate up to a 2.0-mm burr; however, larger guides provide greater clearance to facilitate advancement of the burr through the guide, so an 8-Fr guide may be preferable for the 1.75-mm burr. An 8-Fr guide with an inner diameter of 2.28 mm (0.090 in) can accommodate up to a 2.15-mm burr. A 9-Fr guide would be needed for the 2.25- and 2.38-mm burrs, and a 10-Fr guide would be needed for the 2.5-mm burr. Since 9-Fr and 10-Fr guides are rarely used in the coronaries in the contemporary era, the larger burr sizes are primarily used in peripheral interventions. Operators must be attentive to the specifications of guide catheters from different manufacturers as inner diameters may vary.
Guide extension catheters may facilitate burr delivery to distal, tortuous lesions, but the inner diameter of these catheters limits what sizes can be used. Although the inner diameter of the 6-Fr Guideliner (Vascular Solutions, Minneapolis, MN) is 1.42 mm (0.056 in) and that of the 6-Fr Guidezilla (Boston Scientific) is 1.45 mm (0.057 in), there is minimal clearance for the 4.3-Fr Rotablator drive shaft, and device advancement is not easy. The 8-Fr Guideliner inner diameter is 1.80 mm (0.071 in), which could allow for the 1.25- and 1.5-mm burrs to be advanced.
Coaxial guide positioning is important to ensure easy of passage of the Rotablator burr and avoid trauma to the coronary ostia. The guide can also be used to modify the trajectory of the guide wire to change the direction of the ablation plane, which is important in atherectomy of proximal lesions that are highly angulated or are affected by guide wire bias.
The Rotablator spins over a 0.009-in stainless steel wire with a radiopaque platinum 0.014-in spring coiled tip. The larger tip diameter prevents the burr from spinning beyond the tip of the wire, so the radiopaque dip must be positioned distal to the lesion, because the burr cannot track over this larger segment. Every attempt should be made to keep the guide wire from prolapsing or coiling, because any rotation during burr activation can result in fracture or trapping of the tip in the vessel wall.
Two types of guide wires are currently available. The Floppy RotaWire (Boston Scientific) has a long, tapered shaft that maximizes flexibility to reduce vessel straightening and help relieve unfavorable wire bias in tortuous vessels. On the other hand, in highly calcified vessels, the floppy wire may bias the Rotablator vector preferentially toward normal tissue, leading to eccentric atherectomy or potential dissections. The RotaWire Extra Support wire (Boston Scientific) is a stiffer-bodied wire that increases vessel straightening to help steer the burr through heavily calcified and ostial lesions, but it may create pseudolesions and lead to ablation of normal tissue.
It is possible to angle the guide catheter and manipulate the guide wire to orient the burr closer to the lesion, particularly when treating proximal eccentric and angulated lesions. Pulling or pushing on the wire can direct the burr force vector to the inner or outer curve of the lesion—a concept that has been referred to as directional rotational atherectomy.
Early plaque debulking strategies incorporated a 2-burr approach, with the burr-to-artery ratio of the first burr being approximately 0.5 to 0.6, and that of the second approximately 0.75 to 0.8. In the stenting era, in which the goal is plaque modification, a single burr with a burr-to-artery ratio of at most 0.6 to 0.7 is sufficient, in conjunction with angioplasty and stenting. A burr-to-artery ratio of less than 0.5 is helpful for total occlusions, sharply angulated lesions, or long lesions. Less aggressive atherectomy has been associated with lower rates of restenosis and helps reduce periprocedural complications.48,49
Once the burr is advanced into the coronary artery, it should be placed just proximal to the lesion—the so-called platform segment. The burr is activated in this position, where it can rotate unimpeded, because if the burr is activated while in contact with the vessel wall, the risk of injury is significantly increased.
After positioning the burr in the proximal position, it is important to relieve the tension on the system by pulling back on the advancer knob to prevent the burr from jumping forward into the lesion when the device is activated, increasing the risk of dissection. Once the operator is certain that the tension on the burr has been released, the burr is activated in the platform segment and then advanced to the lesion. Rotation speeds of 140,000 to 160,000 rpm are ideal to optimize atherectomy and reduce complications.
Ablation of the lesion should be performed using a “pecking” technique to reduce thermal injury and embolization. Burr advancement should be gentle. Monitoring the decelerations and avoiding excessive decelerations of >5000 rpm helps to avoid aggressive atherectomy. Contrast injections can be performed to evaluate the progress of the burr and identify any problems with burr orientation or burr-to-artery relationship. The duration of each run should last 15 to 30 seconds, with an interval of 30 seconds and longer between each run to allow for myocardial perfusion and clearance of microparticles. Longer rest periods may be needed to allow for electrocardiographic changes, hypotension, chest pain, or bradycardia to resolve. Once there is no tactile resistance as the burr crosses the treated segment or when no decrease in revolutions per minute are noted during burr advancement, the burr should be removed.
Bradycardia and heart block are often in seen in patients undergoing atherectomy of right or left dominant circumflex coronary arteries, most likely due to microparticulate emboli leading to a parasympathetic effect on the heart. Atherectomy using larger burrs, longer runs, or more revolutions per minute and treating lesions affecting a greater myocardial territory are more likely to induce heart block.
Routine temporary pacemakers are not required when using smaller burrs at lower revolutions per minute, but operators should stop atherectomy runs when transient atrioventricular nodal block occurs and be prepared to place a temporary wire or address this with pharmacologic (eg, atropine) or mechanical maneuvers (eg, coughing) if it does not resolve quickly.
The major clinical complication rates for rotational atherectomy are similar to those reported for balloon angioplasty and include death in 0.9% of patients, Q-wave myocardial infarction (MI) in 1.3%, and emergency bypass surgery in 1.9%, even in the context of higher risk lesions. There is, however, a trend toward a higher rate of non–Q-wave MI (6%), defined as a creatine kinase–myocardial band (CK-MB) level elevated to more than twice the normal value.30 Most of these complications can be avoided by using proper procedural technique and pharmacotherapy.
However, lesions with thrombus, with extensive dissection, and in saphenous vein grafts should be avoided. The choice of guide catheter (eg, based on vessel takeoff, need for support), guide wire, and burr size should be determined at the start of the procedure.
It is worthwhile to note that use of glycoprotein IIb/IIIa inhibitors has been shown to reduce postprocedural elevation of cardiac enzymes by almost 50%, which may translate to a reduction in long-term adverse events.32,33 Glycoprotein IIb/IIIa use has also been shown to attenuate the transient wall motion abnormality sometimes associated with rotational atherectomy,34 and in vitro studies have shown that abciximab can reduce platelet activation caused by a high-speed burr.35 Although there is significant benefit in using these agents, care is needed when lesions are at high risk for perforation, because the level of anticoagulation could have catastrophic effects.
Vasospasm can sometimes complicate rotational atherectomy; therefore, it is important to have vasodilating agents such as nitroglycerine (100-200 μg) available during the procedure. Some operators prefer to use a cocktail of nitroglycerine, verapamil, and heparin in a flush solution for continuous infusion, and some data support this approach.36 Intracoronary adenosine administration has also been shown to reduce the rates of no reflow.37 RotaGlide lubricant is also usually added to the flush solution to reduce friction between the drive coil and guide wire. Vasopressors should also be readily available; dopamine, epinephrine, and phenylephrine are the usual agents.
The Rotablator burr can be advanced forward too quickly, insufficiently atherectomizing the lesion, and then pushed beyond the lesion. Since the atherectomy surface on the Rotablator is only on the proximal surface, it is possible that the burr cannot be withdrawn back through the vessel in this situation. It is also possible that the burr becomes embedded in a fibrotic and calcified lesion and the device stalls and will not rotate. Extremely calcified lesions and tortuous vessels increase the risk for entrapment.50 A 1.25-mm burr is the most likely to inadvertently be forced through a lesion given its smaller size and lower profile shape compared to the larger burrs. Purposeful, but careful, burr advancement during atherectomy helps to avoid this complication, but it cannot always be avoided.
Gently withdrawing the burr using DynaGlide, with constant low revolutions per minute, typically will disentangle a stalled device; however, this is occasionally insufficient to remove the device.
Expanding the lesion with an angioplasty balloon may reduce the resistance of the lesion and allow the burr to be withdrawn. If an 8-Fr guide is used, a second wire and 1.5-mm balloon can be passed alongside the rotablation catheter, and an attempt can be made to pass a wire alongside the entrapped rotablation burr and angioplasty the resistant lesion. A second guide can be used from a second access site, or a smaller guide can be upsized by cutting the distal hub off of the rotablation burr, removing the smaller guide and advancing a larger guide over the rotablation shaft. Alternatively, once the distal hub of the rotablation burr is cut, the outer plastic sheath covering the burr can be removed.51 This will allow for a second wire and balloon to be passed through a 6- or 7-Fr guide.52
A telescoping 5-Fr guide to provide countertraction53 and a gooseneck snare to provide negative traction54 have been described to successfully remove entrapped rotablation burrs. The hub of the rotablation catheter and the outer plastic sheath need to be removed so a telescoping “mother-in-child” guide can be advanced over the rotablation shaft assembly or the snare, and the snare microcatheter will fit alongside the Rotablator within the guide. Additionally, the distal end of the rotablation wire is 0.014 in and can be withdrawn and used to pull back on the rotablation burr, which has a 0.009-in lumen.
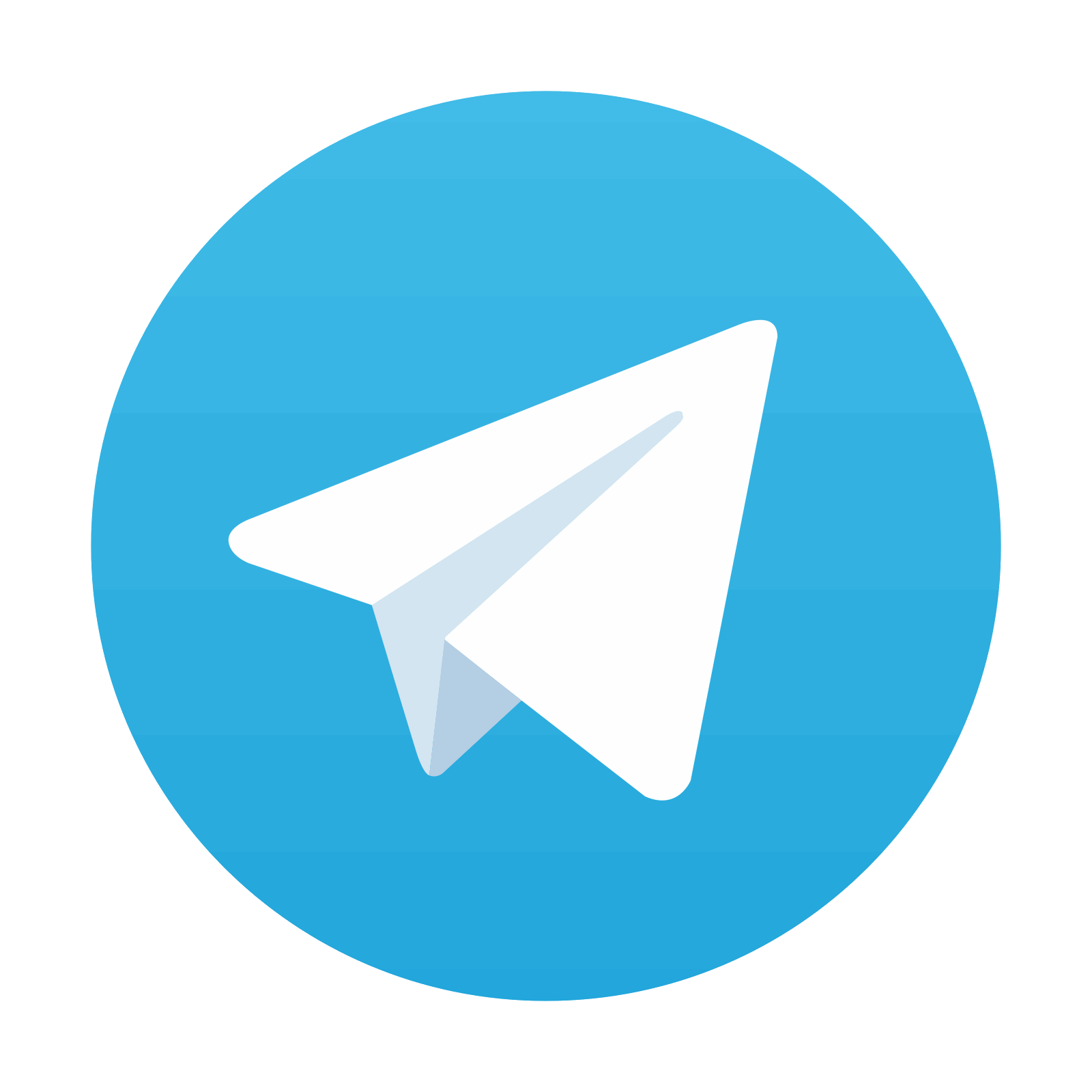
Stay updated, free articles. Join our Telegram channel

Full access? Get Clinical Tree
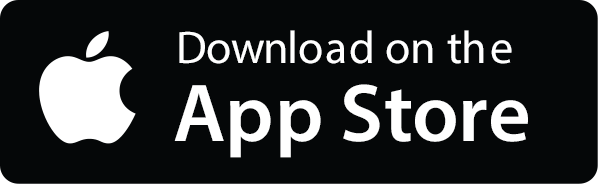
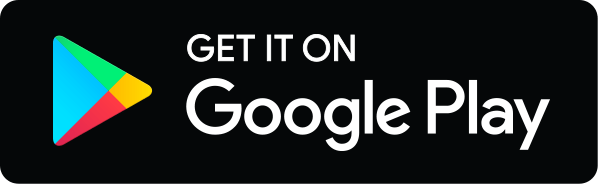