In the stent era, coronary artery perforation (CAP) is the most serious complication and a leading cause of death from percutaneous coronary intervention (PCI). Although preventable to a great extent, perforations are inevitable in any high-volume center. Prompt recognition and treatment can make the difference between benign and fatal outcomes. The incidence of CAP varies with the complexity of disease under treatment and with the aggressiveness of individual operators. An operator who never experiences a perforation is probably underdilating lesions and underdeploying stents. The reported incidence varies from 0.2% to 0.6%.1-5 It is much higher when atheroablative devices are used.5 In a meta-analysis of nearly 200,000 PCIs, the pooled incidence was 0.43%.4 Although there is no good series reporting the effect of stenting on the incidence of perforation, routine stenting most likely increases the perforation rate.
The classification of Ellis and colleagues1 is generally accepted. In this classification, a grade I perforation is an extraluminal crater without extravasation (this might also be termed a pseudoaneurysm); a type II perforation is a pericardial or myocardial blush without contrast jet extravasation; and a type III perforation is an extravasation through a 1-mm or larger perforation. This classification correlates with prognosis. A fourth category is designated cavity spilling, in which the perforation empties into an anatomic cavity: right ventricle, left ventricle, coronary sinus, and so on (Fig. 61-1).
FIGURE 61-1
Grade III perforation from the left anterior descending (LAD) coronary artery to the right ventricle (RV) treated with reversal of anticoagulation. (A) Grade III perforation from mid-LAD to RV. Contrast in RV chamber. (B) Contrast seen in RV outflow track. (C) Twenty minutes after discontinuing bivalirudin anticoagulation and following 20-minute balloon occlusion, the perforation is almost sealed, but a small leak continues. (D) Twenty-five minutes after, only a tiny leak remains. (E) Thirty minute later, the perforation is completely sealed, but the LAD is occluded.

Small perforations can usually be solved by prolonged balloon inflations combined with reversal of anticoagulation. Large perforations require more sophisticated treatment. Perforations in vein grafts frequently resolve with simple measures. This is because scarring around vein grafts may be enough to limit extravasation.
Perforations in proximal arteries require treatments that will both resolve the perforation and preserve antegrade flow. Blocking antegrade flow can sometimes solve more distal perforations. The most practical way to treat perforation in previously occluded arteries is to reocclude the artery. Perforations involving bifurcations are much more difficult to solve, because it may be necessary to stop bleeding in 2 branches while preserving flow to both branches. Occluding flow is the best way to solve perforations of small branches.
Perforations caused by balloons are relatively easy to solve, whereas those caused by stents are generally more severe and more difficult to treat. Devices are 2-edged swords in that debulking prior to stenting may prevent perforations, but the devices themselves can cause very severe and sometimes untreatable perforations.5
Guide wire perforations are more frequent, especially when hydrophilic wires are used in complex cases. In the usual scenario, the guide wire migrates distally in a tiny branch and eventually perforates the distal tip of the branch. This complication may not be recognized until after the guide wire has been withdrawn. Although the leaks are generally small, they can lead to cardiac tamponade. In one reported series, 20% of perforations causing cardiac tamponade were the result of guide wire perforations.6 This is especially a problem in the presence of glycoprotein IIb/IIIa inhibition. Because of relatively small and sometimes unrecognized leaks, guide wire perforation can lead to late cardiac tamponade.
Perforations are a significant problem in patients with chronic total occlusions. Guide wire perforations in this setting are seldom a serious problem, unless the perforation is unrecognized and balloon inflation is made over the perforating guide wire. In cases of chronic total occlusion, a guide wire may enter a tiny branch running parallel to the main vessel. If a balloon is inadvertently inflated within this vessel, serious perforation can ensue. Either of the aforementioned circumstances may result in cardiac tamponade and death. This is particularly distressing when the intervention is entirely elective.
Certain anatomic and morphologic conditions predispose to perforation. Recognition of these circumstances and appropriate adjustments in treatment strategies can prevent problems that would otherwise occur.
Certain locations in the native coronary arteries represent a higher than standard risk for perforation.
In general, lesions on bends present high risks for dissection with balloons and extreme risks for perforation with atherectomy devices. The curving nature of the right and left circumflex arteries may explain the higher incidence of perforation in these vessels.4,5 Excimer laser has the highest perforation risk when used on sharp bends. Excimer laser ablates only in a forward direction. Similar to the headlights on an automobile, laser light points straight when the road turns. Thus, excimer laser invariably causes perforation when used on a sharp bend. Rotablator (Boston Scientific, Marlborough, MA) has some ability to follow bends, but wire bias tends to drive the Rotablator burr toward the outer curvature, thus predisposing to perforation. When using Rotablator on sharp curves, the rule is to undersize the burr by at least 1 and preferably 2 sizes. Directional atherectomy and cutting balloon are less likely to perforate lesions on bends, but the undersize rule is still operative when these devices are used in the curved portions of arteries.
Bifurcations are troublesome to treat no matter what the strategy. Because plaque in this location frequently involves both the main artery and the ostium of the branch vessel, debulking strategies are often employed. The problem is that debulking devices may perforate the carina of the bifurcation, thus producing a very large hole that requires emergent treatment. Treatment of this problem with a covered stent invariably sacrifices the side branch and may not solve the problem. The perforation may involve both branches; therefore, collateral filling of the side branch can lead to continuing leak. Worse, once the stent graft covers the side branch ostium, access to the side branch is no longer possible.
The best way to deal with this problem is to avoid it. Theoretically, the bifurcation could be reconstructed with 2 “kissing” covered stents, but this is technically difficult because of the bulky nature of the stent grafts. In the face of a large perforation at the left anterior descending (LAD)/diagonal bifurcation, it may be best to occlude both branches with kissing balloons and send the patient to surgery.
Because of their tapering nature, branches and distal vessels may be prone to rupture during stenting. Stents of appropriate size for proximal branches may be too large distally. This is particularly a problem in the distal LAD, where diffuse negative remodeling may be present. Figure 61-1 is an example of a long, drug-eluting stent in the LAD with a perforation occurring at the distal one-third of the stent. With the advent of drug-eluting stents, it is more common to stent more distally and to use longer stents. The best way to approach these tapering vessels is to carefully interrogate them with intravascular ultrasound (IVUS) before and after stenting. Stents should be sized appropriately for the distal vessel, and then postdilated proximally with short balloons of larger sizes.
Lesions of the right coronary artery (RCA) at the acute margin represent a significant risk for perforation, especially with devices. The problem with this area is that the artery bends more acutely than is generally appreciated in the left anterior oblique projection. Compounding the problem is the bifurcation with the anterior ventricular branch and the propensity for severe stenoses to involve this bifurcation. When devices are used to treat this area, there is a tendency for the device to migrate in the direction of the anterior ventricular branch and thus perforate the artery at the inferior aspect of the bifurcation. This results in extremely large perforations that can become catastrophic if not treated within seconds.
Calcified arteries are noncompliant. When stretched to extreme degrees, they tend to tear, especially at junctures where heavily calcified areas meet relatively normal ones.7 This is a particular problem when stents are placed in these vessels without proper preparation with Rotablator or cutting balloon. When the stents are deployed, they cannot be expanded fully. High-pressure balloons are then used, and the resulting uneven expansion of noncalcified areas leads to perforation or rupture. The mechanisms are similar to those described under vein grafts and eccentric lesions (vida infra).
Vein graft perforations generally occur when a resistant lesion suddenly responds to high-pressure balloon inflation. Pathologically, these are tears or ruptures as opposed to discrete holes. These resistant lesions may be the result of old fibrosis, sometimes outside the wall of the vein graft. The vein graft itself is relatively thin walled. Once resistance of the fibrous tissue is overwhelmed, the graft itself ruptures. Clinically, this is seen as an imprint that persists on an inflated balloon despite high pressure. If the imprint suddenly resolves as, for example, after a single bar increase in pressure, the operator should be concerned that rupture has occurred. In this instance, it is prudent to inject around the deflated balloon before removing it. If perforation has occurred, the balloon can be reinflated at low pressure while anticoagulation is reversed and preparations are made for stent grafting.
Because vein grafts are normally embedded in scar tissue and there is no pericardium, vein graft perforations usually act differently from those in native vessels. Following perforation of a vein graft, extravasation is generally limited by the dense surrounding cicatrix. This is especially true of vein grafts to the lateral left ventricle and posterior surfaces of the heart. These perforations can usually be treated with reversal of anticoagulation and observation. There are exceptions to this rule, especially in the proximal and mid-portions of grafts to the LAD and RCA. Vein graft perforations tend to be large. When they do bleed freely, blood loss is extreme, and early and effective treatment is mandatory. The first treatment must always be to cover the perforation with an inflated balloon.
Plaque eccentricity is the single most important morphologic feature that portends a propensity to perforation.7 Thick atheroma on one side and thin, frequently normal, arterial walls on the other characterize eccentric plaques. Balloon inflation and stenting tend to preferentially stretch the thin normal wall while barely affecting the noncompliant plaque. This is especially true when the plaque is diffusely fibrotic or heavily calcified. This is one condition in which preparation of the vessel with cutting balloon or a debulking device prior to stenting may actually reduce the risk of perforation. Routine use of IVUS prior to stenting will identify extreme eccentricity that may not be evident on the angiogram. Sumitsuji and colleagues in the Japanese Intervention and Ultrasound Study group have identified 3 ultrasound patterns that may predict perforation: (1) severe eccentric calcified plaque with weak segment on the opposite side; (2) severe eccentric fibrous plaque with weak segment on the opposite side; and (3) severe superficial calcium with weak segment in the opposite side.7
Guide wire perforations are a product of technologic advance (Fig. 61-2). They occur most frequently with hydrophilic wires. The extreme lubricity of these wires allows them to migrate without being noticed distally into and out of the tips of small branches. During difficult cases, involving multiple balloon exchanges, it is not unusual to find the tip of a hydrophilic wire several centimeters into the pericardial space. Usually, this does not result in a clinical problem. Occasionally, it can result in a minor or major leak into the pericardium that is difficult or impossible to resolve.
FIGURE 61-2
Guide wire perforation treated with thrombin injected through a microcatheter. (A) Guide wire in distal portion of small branch. (B) Faint contrast stain during first post–percutaneous coronary intervention injection. (C) Subsequent injection shows grade II perforation. (D) Balloon inflated at low pressure temporarily stops the leak. (E) After balloon inflation, the perforation is not fully sealed. (F) Grade II leak resumes within a few minutes after balloon inflation. (G) After thrombin administration, the perforation is sealed without interrupting flow to other branches.


The best way to prevent guide wire perforations is to avoid hydrophilic wires whenever possible, but guide wire perforations can also occur with conventional wires, especially ones with stiffer tips. The guide wire perforation in Figure 61-2 was caused by a conventional wire with intermediate tip stiffness. Unfortunately, hydrophilic wires are most useful in the most difficult cases. In such cases, it is good practice to exchange the hydrophilic wire for a conventional wire at the earliest opportunity. When using hydrophilic wires, it is important to be constantly aware of distal tip position and to guard against distal migration. Problems with guide wire perforation are accentuated in the presence of glycoprotein IIb/IIIa inhibitors. Most guide wire perforations can be easily solved with reversal of anticoagulation. When IIb/IIIa inhibitors are used, anticoagulation reversal is prolonged.2
In a single case, I had the experience of a pinhole leak in the aortic root resulting from stenting of the RCA ostium. This occurred in an elderly patient with a calcified aorta. The aortic location of the leak was immediately above the RCA ostium and could not be distinguished angiographically from a proximal RCA perforation. This problem was only identified at surgery after stent grafting of the proximal RCA and its ostium failed to correct the problem. It was possible to suture the small aortic hole without cardiopulmonary bypass and without grafting the coronary artery.
Effective management of perforations is greatly dependent on the ability to reverse anticoagulation. Different anticoagulants have different levels of reversibility and differing times required to reverse their effects. All anticoagulants can be reversed with large amounts of fresh frozen plasma and platelets. Unfortunately, the time required to obtain these agents from the blood bank and subsequently administer them is at best 1 hour and usually much longer. Although it has been my general practice to fully reverse anticoagulation in the presence of any perforation ≥ grade 2, there is evidence that partial reversal of anticoagulation is safe.2
Heparin is the anticoagulant most commonly used for PCI. In the case of perforation, it is the anticoagulant that can be most quickly and completely reversed (using protamine). Although protamine reversal should be instantaneous, in reality, it takes almost 30 minutes to achieve a normal activated clotting time (ACT) in the setting of perforation. Because protamine titrations are not generally available in the catheterization laboratory, it is necessary to perform a de facto titration by guessing at the initial protamine dose and giving additional protamine after serial ACT measurements. There is a minimum delay of 10 to 15 minutes between doses. If too much protamine is given initially, the protamine itself acts as an anticoagulant, and the operator does not know what to do in the face of a persistently elevated ACT. There is also the problem of reabsorption of heparin from adipose tissue and release of protein-bound heparin. Thus, the theoretically instantaneous reversal of heparin does not take place under real-world conditions. The heparin problem is further complicated by the concomitant use of glycoprotein IIb/IIIa inhibitors. It is extremely difficult to reverse anticoagulation in the presence of these agents.2,8
Direct thrombin inhibition with bivalirudin in lieu of heparin has become increasingly popular. The advantages of bivalirudin are predictable pharmacokinetics and the ability to avoid concomitant administration of a glycoprotein IIb/IIIa inhibitor. The half-life of bivalirudin is 25 minutes. After 2 half-lives (50 minutes), anticoagulation is essentially finished. Although there is no readily available antagonist to bivalirudin, its effect is dissipated in less time than it takes to obtain and infuse fresh frozen plasma. Because bivalirudin blocks thrombin-induced platelet aggregation, it is unnecessary to give concomitant glycoprotein IIb/IIIa inhibitors, even in the presence of unstable angina.7 This is a major advantage of bivalirudin, if perforation occurs.
Theoretically, the presence of thienopyridines should make it difficult to stop bleeding after coronary perforation. There is no specific information on this subject either in the literature or in my personal experience. My colleagues and I give thienopyridines during or immediately after stent procedures; pretreatment is the exception. However, an increasing number of patients undergoing PCI are receiving chronic thienopyridine therapy. In these patients, platelet transfusion would be the only way to completely reverse anticoagulation. Newer antiplatelet agents, specifically prasugrel and ticagrelor, have a more rapid onset of action compared to clopidogrel. Theoretically, this could be problematic in the presence of CAP, but there are no reports of problems with these agents in the presence of CAP.
Glycoprotein IIb/IIIa inhibitors present the greatest problem when attempting to reverse anticoagulation after coronary perforation. Despite relatively short half-lives and low blood levels, the effects of these agents generally persist.2,8 Although there is no series that documents a poorer prognosis in patients with perforation who have been treated with IIb/IIIa inhibitors, it is generally accepted that that these agents complicate the treatment of perforation. There are only 3 possible solutions: (1) avoid use of IIb/IIIa inhibitors whenever possible; (2) avoid coronary perforation when IIb/IIIa inhibitors must be used; or (3) transfuse platelets when perforation occurs in the presence of IIb/IIIa inhibition. In my opinion, glycoprotein IIb/IIIa inhibition is only indicated in the presence of thrombus (ie, acute coronary syndromes). High-risk situations for perforation occur mostly in chronic settings in which IIb/IIIa inhibition has little rationale. When an operator approaches situations that are high risk for perforation, this risk must be carefully evaluated before administration of these agents.
Although devices make it possible to successfully treat otherwise untreatable lesions, the perforation rate with devices is more than double the rate with balloons alone.2,9 Devices have been discussed elsewhere in this chapter, especially in relation to lesions on bends, eccentric lesions, and bifurcation lesions. Here, each of the devices is discussed on an individual basis. In general, when used incorrectly, devices can be a source of massive perforation. When used correctly, they can actually prevent perforation by changing the compliance of diseased arteries and allowing ballooning and stenting at lower pressures.
Balloons and stents are responsible for more perforations than all other devices combined. The major causes of perforations with balloons and stents are oversizing and stenting of highly eccentric lesions. In the series by Sumitsuji,7 the stent-artery ratio was 1.25 or more in 67% of perforations, and 72% of perforations occurred when eccentric lesions were treated. Guidance using IVUS or optical coherence tomography (OCT) is invaluable in accurately sizing vessels and assessing eccentricity.
Although Rotablator use has decreased over the past 15 years, this device is invaluable in the presence of heavy calcification. Perforation secondary to Rotablator occurs mostly with larger burr sizes and when treating lesions on sharp bends. In recent years, I have avoided large burr sizes, because complete debulking is no longer my primary goal. Rather, I am trying to merely change lesion and vessel compliance to permit full stent deployment. This goal can usually be accomplished using burr sizes of 1.5 mm or less—never more than 1.75 mm. With lesions on sharp bends, I invariably start with a 1.25 burr, and check compliance with a balloon before going to larger burr sizes. Compliance is evaluated by the ability to fully inflate a 2.5-mm or 3.0-mm balloon. When a larger stent is contemplated, compliance is verified with a larger balloon. If full balloon inflation is possible, then it should also be possible to fully deploy a stent. If an imprint remains on the balloon, even at high pressure, then further rotational ablation is necessary. I never exceed a burr size of 1.5 mm for lesions on sharp bends.
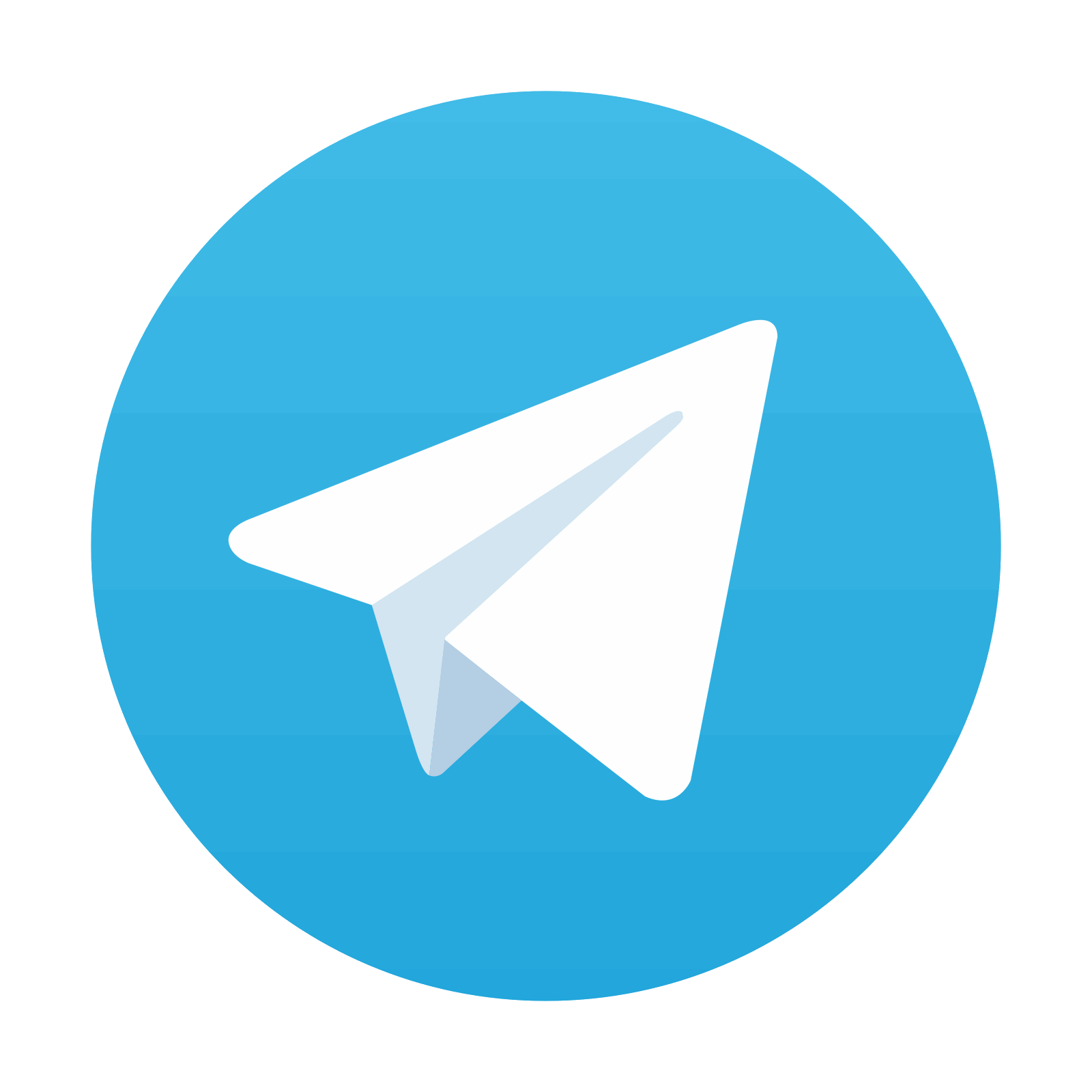
Stay updated, free articles. Join our Telegram channel

Full access? Get Clinical Tree
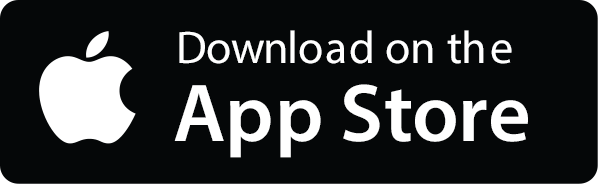
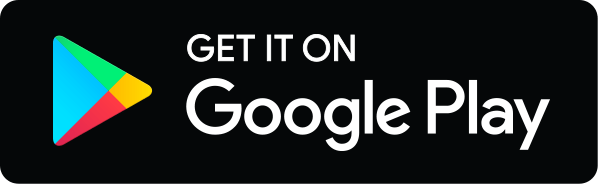