INTRODUCTION
While the importance of hypertension in the pathogenesis of diastolic dysfunction and diastolic heart failure is well known, relatively less attention has been paid to the interaction between coronary artery disease (CAD) and diastolic dysfunction. Accordingly, this chapter will be devoted to reviewing aspects of diastolic dysfunction in patients with coronary disease, with an emphasis on the role of Doppler echocardiography in elucidating aspects of the pathogenesis of heart failure.
Diastolic heart failure is a condition characterized by signs and symptoms of heart failure, where “the dominant abnormality resides in diastole.” Cross-sectional and population-based studies indicate that at least one-third of all patients with congestive heart failure (CHF) have a normal or near-normal ejection fraction (EF) and that most have diastolic heart failure. The prevalence is highest in patients over age 75. Historically it has been assumed that the mortality rate of diastolic heart failure is less than that of systolic heart failure, ranging from 5% to 8% annually, compared with 10% to 15% in patients with systolic heart failure. However, recent data have challenged this notion. As is the case with systolic heart failure, the mortality rate of diastolic heart failure is directly related to age and the presence of coronary disease. The reported prevalence of diastolic heart failure depends not only on age, but on the diagnostic criteria used to define diastolic dysfunction, the etiology of the disease, and the methods and design of the particular study in question.
The prevalence of coronary disease in patients who develop diastolic heart failure is high. This conclusion is based on data compiled from several types of studies: cross-sectional studies; randomized, placebo-controlled, clinical trials; and community-based studies. Gottdiener et al. studied over 5000 community-based individuals, age 65 or greater, enrolled in the multicenter Cardiovascular Health Study (CHS). These investigators tabulated incidence rates of heart failure over a 5-year period. Prevalent CAD was associated with more than a threefold higher rate of incident CHF (15.3% vs. 41%). Other predictors of CHF include other indicators of prevalent atherosclerotic disease, such as reduced ankle-brachial index, history of stroke, and increased levels of C-reactive protein. The population-attributable risk of prevalent CAD in this study was roughly equivalent to that of arterial hypertension, which itself is epidemiologically important. In a subsequent analysis of CHF by EF in the CHS database, prevalent coronary heart disease was present in 58% of patients with CHF and normal EF and in 78% of patients with CHF and reduced EF.
Framingham Study investigators confirmed the importance of CAD in patients with diastolic heart failure, using the concept of population-attributable risk. When adjusting for age and heart failure risk factors in proportional hazards regression models, hypertension accounted for 39% of cases in men and 59% of cases in women. Among hypertensive subjects, CAD (in this instance, a history of myocardial infarction) was associated with increased risk for CHF in both sexes.
Similarly high rates of CAD have been reported in a survey of clinical characteristics of patients hospitalized in New York City with diastolic heart failure reported by Klapholz et al., as well as those enrolled in the “Candesartan in Heart Failure: Assessment of Reduction in Mortality and Morbidity” (CHARM)–Preserved study, the only published randomized clinical trial to date of treatment for diastolic heart failure. In the study by Klapholz et al., roughly one half of patients hospitalized in New York City for CHF who had a normal EF had a history of CAD. In the CHARM study, roughly one fifth had a prior coronary artery bypass graft; one half, myocardial infarction; and one quarter, angina. These data demonstrate the importance of coronary heart disease in the pathogenesis of diastolic heart failure.
PATHOPHYSIOLOGY
As is reviewed in Chapter 2 of this volume, diastolic function is determined by the passive elastic properties of the left ventricle and the process of active relaxation. Active relaxation is an energy-dependent process that is affected by ischemia. By contrast, abnormal passive elastic properties are generally caused by a combination of increased myocardial mass and alterations in the extramyocardial collagen network. The effects of impaired active myocardial relaxation, caused by acute ischemia, can lead to further stiffness. The interplay between this impaired relaxation and passive stiffness determines left ventricular (LV) filling pressure.
Acute Ischemia
It has been demonstrated that acute myocardial ischemia effects changes in both global and regional LV diastolic properties. The results of these alterations include a slower LV pressure decay with a consequent slowing of diastolic filling. Acute changes in the passive properties of the left ventricle result in an upward shift in the diastolic pressure-volume relationship ( Fig. 22-1 ). As a result, the LV diastolic pressure-volume relationship changes: Chamber compliance is reduced, the time course is altered, and diastolic pressure is elevated. Under these circumstances, a relatively small increase in central blood volume or an increase in venous tone or arterial stiffness can produce a substantial increase in filling pressures and may result in acute pulmonary edema.

The slowed relaxation following acute ischemia or infarction may persist for days. However, a slower rate of LV pressure fall is not the only result of direct effects of ischemia on myocardial relaxation. Ischemia may also affect contractility, loading conditions, and may worsen nonuniformity, which may also act to slow the rate of LV pressure decline. This subject is reviewed in detail elsewhere.
Postinfarction Left Ventricular Remodeling
The subject of postinfarction LV remodeling is complicated, and excellent reviews of this topic have been published. To summarize briefly, myocardial infarction produces global and regional changes in ventricular function in both infarcted and noninfarcted regions, resulting in alterations in LV shape and geometry. The immediate changes following infarction involve mainly the infarcted region. Subsequently, over weeks to months, further changes occur, and these involve the noninfarcted region. This constellation of changes is known as ventricular remodeling. In the consensus paper by Cohn et al., LV remodeling is defined as “changes in size, shape, and function of the heart after cardiac injury. [The process] … is influenced by hemodynamic load, neurohumoral activation, and other processes.”
A review of the processes involved in LV remodeling make it clear that what may begin as an adaptive process can have malefic consequences for LV diastolic function. During acute ischemia, injury, and the attendant reduction in systolic function, the region undergoing infarction may develop systolic expansion and perform a reduced amount of effective work. Following a large myocardial infarction, LV end diastolic pressures remain elevated, and the volume of viable myocardium increases approximately 30% because of increases in both myocyte cell length and diameter. In some instances, the ventricle may undergo progressive infarct expansion, usually within the first 24–72 hours. In part, this expansion and volume increase help to compensate for the reduction in effective work via the Frank-Starling mechanism. Even in the absence of acute LV expansion, LV dilation may develop, usually over several months after myocardial infarction involving both the infarcted and the noninfarcted regions.
Given the curvilinear diastolic pressure-volume relationship, lengthening/dilation of noninfarcted regions is associated with an increase in LV end diastolic pressure. High wall stresses and ventricular remodeling may provide the stimuli for deleterious changes and further remodeling. The signal for progressive remodeling in the postinfarction ventricle may be diastolic wall stress, with parallels between this process and what is observed in chronic volume overload due to valvular regurgitation. Myocyte hypertrophy and changes in the extracellular matrix also are part of the remodeling process.
CLINICAL RELEVANCE
Diagnosis by Doppler Echocardiography
Doppler echocardiography plays a critical diagnostic role in all patients with heart failure for a variety of reasons. First, diagnostically, it should be emphasized that the physical examination, electrocardiogram, and chest roentgenogram do not reliably distinguish diastolic from systolic heart failure. Second, an accurate EF determination is essential for the diagnosis of diastolic heart failure. Echocardiography can also rapidly exclude diagnoses such as acute mitral or aortic regurgitation or constrictive pericarditis, conditions also associated with signs and symptoms of heart failure and a normal EF. Finally, Doppler echocardiography can be used in many instances to reliably estimate filling pressures.
General Principles
As is reviewed in Chapter 5 of this volume, Doppler echocardiography measures the velocity of intracardiac blood flow. Doppler transmitral flow analysis has become the mainstay of the clinical assessment of diastolic function. In normal sinus rhythm, diastolic flow from the left atrium to the left ventricle across the mitral valve occurs in two phases, denoted by two distinct waves: the E wave, which reflects early diastolic filling, and the A wave, in late diastole, which reflects atrial contraction. Because the velocity of blood flow across the mitral valve depends on the transmitral pressure gradient, the E-wave velocity is influenced by both the rate of early diastolic relaxation and left atrial (LA) pressure. Alterations in the pattern of these velocities give insight into LV diastolic function and prognosis. As has been pointed out previously (see Chapter 10 ), standard mitral inflow patterns are extremely sensitive to loading conditions, particularly LA pressure.
Stated another way, early diastolic filling rates might be increased by elevated LA pressure. Therefore, just as a “normal” (E > A) filling pattern does not necessarily indicate normal diastolic function, a reduced E-wave velocity and E/A ratio might be found in the presence of elevated filling pressures. As we have come to realize over the past decade, other data are necessary to give a complete picture of diastolic function. Such other data are generally provided by pulmonary vein velocities and tissue Doppler analysis. Tissue Doppler techniques appear to be somewhat less sensitive to loading conditions than standard Doppler velocities ( Fig. 22-2 ). It is important to bear in mind, in this connection, that the height of the transmitral E wave merely reflects the early diastolic LA-LV gradient. As is shown in Figure 22-3 , it is possible that the E-wave height and E/A ratio may be low, not necessarily because of low LA pressure, but because of a protracted decline in LV diastolic pressure. As we will see, such a protracted decline in diastolic pressure may be caused by acute ischemia.


Acute Effects of Ischemia on Doppler Inflow Patterns
There have been a number of studies of mitral inflow patterns in patients with acute myocardial ischemia ( Table 22-1 ). Labovitz et al. studied functional changes by Doppler echocardiography in 32 patients during transient myocardial ischemia induced by percutaneous transluminal coronary angioplasty (PTCA). Ventricular filling during coronary occlusion showed significant change, evident within 15 seconds of balloon occlusion; there was an acute decrease in peak E velocity as well as E/A ratio ( Fig. 22-4A and B), with a return to baseline values within 15 seconds following balloon deflation. The diastolic changes occurred prior to systolic and other clinical changes.
AUTHOR | NUMBER OF PATIENTS (n) | PATIENT POPULATION | E | A | E/A | AFF | DT | IVRT |
---|---|---|---|---|---|---|---|---|
Labovitz et al. | 32 | Patients undergoing PTCA | NA | NA | ↓ | NA | NA | NA |
Snow et al. | 22 | Patients in the unstable angina group who underwent elective PTCA * | NA | NA | ↔ | ↔ | ↓ | ↓ |
Iliceto et al. | 9 | Patients with significant CAD who developed ischemic ECG changes with transesophageal atrial pacing | ↓ | ↑ | ↓ | ↑ | NA | NA |
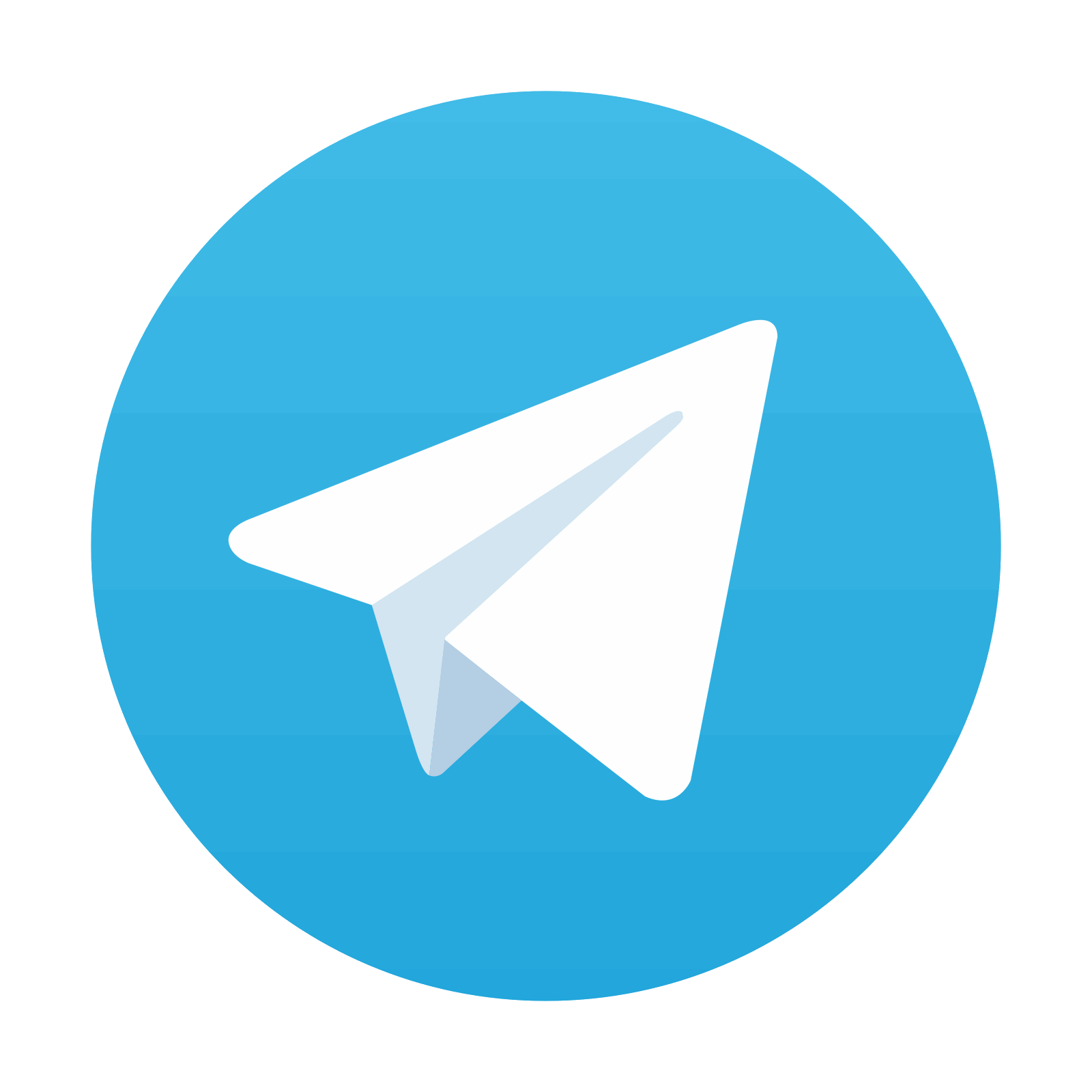
Stay updated, free articles. Join our Telegram channel

Full access? Get Clinical Tree
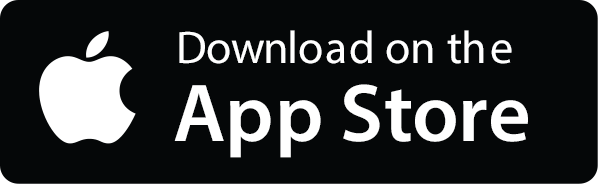
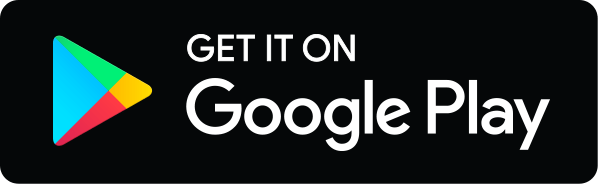
