Generic name
Gadopentetate dimeglumine
Gadoteridol
Gadodiamide
Gadoversetamide
Gadobenate dimeglumine
Gadoxetate
Gadobutrol
Gadoterate
Gadofosveset trisodium
Trade name
Magnevist
ProHance
Omniscan
OptiMARK
MultiHance
Eovist
Gadavist
Dotarem
Ablavar
Manufacturer
Bayer healthcare
Bracco diagnostics
GE healthcare
Mallinckrodt
Bracco diagnostics
Bayer healthcare
Bayer heatlhcare
Guerbet
Lantheus medical imaging
Label dose
0.1 mmol/Kg (0.2 mL/Kg)
0.1 mmol/Kg (0.2 mL/Kg)
0.1 mmol/Kg (0.2 mL/Kg)
0.1 mmol/Kg (0.2 mL/Kg)
0.1 mmol/Kg (0.2 mL/Kg)
0.025 mmol/Kg (0.1 mL/Kg)
0.1 mmol/Kg (0.1 mL/Kg)
0.1 mmol/Kg (0.2 mL/Kg)
0.03 mmol/Kg (0.12 mL/Kg)
Approval year
1988
1992
1993
1999
2004
2008
2011
2013
2008
Approved indication
Central nervous system
Extracranial/extraspinal Tissues
Body (excluding the heart)
Central nervous system
Extracranial/extraspinal tissues
Central nervous system
Body (excluding the heart)
Central nervous system
Liver
Central nervous system
Hepatobiliary disease
Disrupted blood brain barrier
Central nervous system
Breast
Central nervous system
Aortoiliac occlusive disease
Approved age
≥2 years
2–18 years and adults
2–16 years and adults
18–76 years
≥2 years
Adults
≥2 years
≥2 years
Adults
Molecular structure
Linear, ionic
Linear, ionic
Cyclic, nonionic
Linear, nonionic
Linear, nonionic
Linear, ionic
Macrocyclic, nonionic
Macrocyclic, ionic
Linear, ionic
Chelating agent
DTPA
HP-DO3A
DTPA-BMA
DTPA-BMEA
BOPTA
EOB-DTPA
DO3A-butrol
DOTA
Fosveset
Elimination pathway
Renal
Renal
Renal
Renal
Renal (97 %) and biliary (3 %)
Renal (50 %) and biliary (50 %)
Renal
Renal
Renal (91 %) and biliary (9 %)
Serum elimination half-life
96 ± 7.8 min
94 ± 4.8 min
77.8 ± 16 min
104 ± 19.5 min
70–121 ± 15.6–36 min
55–57 min
1.33–2.13 h
1.6 ± 0.2 h
16.3 ± 2.6 h
In addition to its need for safety purposes, the chelate has multiple effects on gadolinium. When gadolinium is bound to a chelate with a large molecular structure, its rate of tumbling slows resulting in an increase in relaxivity [6]. Conversely, chelates decrease the T1 shortening effect of gadolinium by reducing the interaction between Gd3+ ions and 1H protons [7]. Additionally, the renal excretion of chelated gadolinium increases approximately 500-fold when compared to that of free Gd3+ ions [8].
The coordinated water molecule also plays a critical role in contrast enhancement. Its proximity to the gadolinium ion results in efficient relaxation. It undergoes rapid chemical exchange with the solvating water molecules, resulting in a catalytic effect whereby the gadolinium complex shortens the relaxation time of the bulk solution [2]. GBCAs transiently bind to water molecules which results in a shortening of the T1 relaxation time and the production of a brighter signal. This can be simply quantified by the following equation: [2]
Where T1 is the observed relaxation time with contrast agent, T1 0 is the relaxation time before addition of the contrast agent, [CA] is the concentration of the contrast agent, and R1 is the relaxivity of the GBCA. As demonstrated by this equation, the contrast agent usually increases the relaxation rate (1/T1) proportionally to the amount of contrast agent [2]. Furthermore, the performance of the contrast agent is highly dependent on its relaxivity (R1). Of note, although the linear relationship of the equation is maintained at the concentrations used in routine clinical imaging, it is not preserved at the extremes of the concentration spectrum [9]. For example, very high concentrations actually reduce the signal intensity as the T2 shortening effect of the contrast agent then becomes dominant [9].
![$$ 1/{\mathrm{T}}_1=1/{{\mathrm{T}}_1}_0+{\mathrm{R}}_1\times \left[\mathrm{C}\mathrm{A}\right] $$](/wp-content/uploads/2016/07/A315651_1_En_19_Chapter_Equa.gif)
Classification of GBCAs
There are several methods with which to classify MRI contrast agents, none of which are all encompassing. The most commonly used contrast agents for cardiovascular imaging are gadolinium based. There are currently nine available GBCAs approved for use by the FDA in the United States. Table 19.1 summarizes the properties of these FDA approved GBCAs.
GBCAs can be further classified into categories based on their biodistribution in tissues, which is determined by the chemical structure of the chelator. The two most clinically applicable categories are the extracellular and blood pool/intravascular contrast agents.
Extracellular Contrast Agents
The first approved GBCAs were extracellular contrast agents (ECCAs). As demonstrated in Table 19.1, each agent has a generic name and a trade name. Despite the complicated nomenclature, these agents share three similar properties in chemical structure. They are gadolinium-based, contain a chelate bound to the gadolinium, and have a single water molecule coordination site to the gadolinium [2].
After intravenous injection, ECCAs distribute rapidly into both the intravascular and extracellular spaces, a process which can be divided into three phases: arterial, venous, and equilibrium phases. Initially, the water-soluble contrast agent distributes into the intravascular space. The arterial phase is the time between contrast arrival in the region of interest and subsequent venous filling [9]. This phase is typically less than 20 s in duration; therefore, the timing of arrival of the contrast bolus with imaging is critical. The arterial phase is followed by venous filling (venous phase). Approximately 10 min later, the extracellular contrast agent redistributes into interstitial spaces, known as the equilibrium phase.
Elimination of contrast agents occurs rapidly and without biotransformation through glomerular filtration in the kidney [1]. The plasma elimination half-life is typically 70–90 min in the setting of normal kidney function with approximately 98 % of the dose being cleared within 24 h [7]. In patients with renal impairment, the plasma half-life is lengthened. In one study, the elimination half-life of Gadodiamide (Omniscan) increased to 34.2 h in a patient with severe renal insufficiency (GFR 2–10 mL/min) [10]. Hepatic excretion is negligible, even in those with severely reduced renal function except for Gadoxetate (Eovist) which has 50 % biliary excretion making it useful for hepatobiliary disease imaging. Other available ECCAs include Gadopentetate dimeglumine (Magnevist), Gadoteridol (ProHance), Gadodiamide (Omniscan), Gadoversetamide (OptiMARK), Gadobenate dimeglumine (MultiHance), Gadobutrol (Gadavist) and Gadoterate (Dotarem).
Intravascular/Blood Pool Contrast Agents
Extracellular contrast agents are frequently used off-label for MR angiography (MRA) with imaging targeted to their arterial or venous phase as required. However, because of their pharmacokinetic profile, they are not the ideal agents for this use given their short period of peak vascular enhancement before rapid extravasation into the interstitial space. Intravascular or blood pool contrast agents were specifically designed to overcome this limitation through deliberate confinement to the intravascular space, resulting in a longer plasma half-life with prolonged intravascular enhancement [11]. These agents allow for the imaging of multiple vascular beds with a higher intravascular signal than is achievable with the use of ECCAs. Furthermore, the need for bolus timing as with ECCA is eliminated, as the optimal imaging window is significantly longer on the order of tens of minutes as compared to seconds with the use of ECCAs.
The distribution and elimination of a contrast agent can be altered by modifying the size, charge and molecular shape of the ligand relative to the permeability of the capillary bed. The higher the molecular weight of the compound, the slower its elimination half-life and clearance are [1]. Early blood pool agents consisted of gadolinium covalently linked to very large molecules such as polylysine, dextran, and modified bovine serum albumin. These large compounds had restricted diffusion out of the vascular space with good imaging results. However, this resulted in very slow elimination creating concerns about a potential immunologic response in preclinical studies [12]. This led to the revised approach of synthesizing a compound large enough to be partially or completely confined to the vascular compartment in some tissues, but small enough to be eliminated by glomerular filtration. As a point of reference, the capillary beds in myocardial tissue are lined with an uninterrupted layer of endothelial tissue, allowing for diffusion of only small molecules (such as Gd-DTPA, weighted 590 Da), whereas the fenestrations in the glomerular capillaries are 60–70 nm in diameter and allow for passage of any molecule weighing ≤20,000 Da [1].
In December 2008, the FDA approved the use of Gadofosveset (Vasovist or Ablavar, previously known as MS-325), making it the first commercial agent to be approved in this class [13]. Gadofosveset is a unique gadolinium-based contrast agent in that it binds reversibly to serum albumin. Reversible albumin binding serves various purposes: (1) the albumin binding slows extravasation of the contrast agent, thus allowing the contrast agent to remain in the intravascular space for a much longer period of time (plasma half-life approximately 15.5 h), (2) the reversibility still allows a path for excretion, and (3) the relaxivity of the contrast agent is increased four to tenfold by its binding to albumin allowing for the administration of a much smaller dose. In the United States, gadofosveset is FDA approved only for aortoiliac disease in adults with other uses being off-label at present. Compared to traditional ECCAs, blood pool agents allow for both arterial and venous phase imaging, as well as delayed venous imaging and cover a larger anatomic area, e.g. head to foot without re-injection.
There are several other intravascular agents in clinical development, including Gadocoletic acid (also known as B-22956 and B22956/1) which is a Gd-DTPA derivative and binds to albumin. Polymeric gadolinium chelates are also blood pool contrast agents that are in development and exhibit higher relaxivity and more pronounced contrast enhancement than traditional ECCAs [14]. Sometimes, the extracellular contrast agent Gadobenate dimeglumine, Gd-BOPTA (MultiHance) is erroneously categorized as a blood pool agent due to its affinity for albumin, which results in a modest increase in relaxivity relative to the ECF agents [2, 5]. However, as it only binds weakly and transiently to albumin, it should not be classified as such.
Novel/Experimental Contrast Agents
Ferumoxytol
Ferumoxytol (Feraheme, AMAG Pharmaceuticals, Cambridge, MA) is a novel ultrasmall paramagnetic iron oxide (USPIO) nanoparticle, which is FDA approved as an intravenous iron replacement therapy for the treatment of iron deficiency anemia in adult patients with chronic kidney disease. Ferumoxytol has recently been investigated extensively as an intravenous contrast agent in MRI [15]. Ferumoxytol is given as a rapid intravenous injection, has a long half-life on the order of 14–15 h and distributes only in the intravascular compartment behaving as a blood pool agent. Ferumoxytol is made of iron oxide particles surrounded by a carbohydrate coat and as an iron replacement agent it carries no risk of nephrogenic systemic fibrosis (NSF). It has no renal excretion and is cleared by macrophages over several days. It shortens T1 leading to strong enhancement on T1-weighted imaging but also can cause strong susceptibility artifacts due to shortening of T2*. Dilution of the ferumoxytol minimizes its marked T2* shortening effects, which can lead to signal loss [16]. Ferumoxytol has been used off-label as a MRA blood pool agent, in the imaging of the carotid arteries, thoracic and abdominal aorta, renal arteries and peripheral arteries and veins. The main advantage is likely to be in patients where GBCAs use is contraindicated, e.g. in patients with end-stage renal disease with or without dialysis where it has been studied extensively as an iron replacement therapy.
Manganese Based Contrast Agents
Manganese (Mn) is a non-lanthanide metal which has been used as a paramagnetic contrast agent in MRI. Manganese ion (Mn2+) works primarily as GBCAs by shortening the T1 thus leading to increase in signal intensity on T1-weighted imaging. Similar to Gd, Mn in its free form is toxic and needs to be chelated before administration. At the cellular level, Mn is transported into the cells through calcium channels and accumulates in the mitochondria [17]. Because of its involvement in mitochondrial function, Mn uptake is based on mitochondrial density in the cell which makes it an attractive contrast agent for liver, pancreas, kidney, heart and other mitochondrial rich organs. Manganese based contrast agents have been studied in Manganese-enhanced MRI (MEMRI) for the assessment of tissue viability. In a study of ten patients with recent myocardial infarction, use of manganese dipyridoxyl diphosphate (MnDPDP) showed increased uptake by normal myocardium compared to infarcted myocardium [18]. In a mouse model of acute and chronic myocardial infarction, MEMRI using MnCl2 was compared with late gadolinium enhancement (LGE) using Gadodiamide [19]. In the acute stage, LGE overestimated infarct size compared to MEMRI and histology (+12.0 % ± 3.6 %, P = 0.001) while no difference in infarct size was found in chronic stage. A major drawback of using Mn in free ionic form (MnCl2) is its cellular toxicity (cardiotoxicity, neurotoxicity), however, unlike GBCAs there is no risk of NSF with Mn use. Some toxicity has been observed in animal experiments even with low-affinity chelates. Two Mn based contrast agents, intravenous Mn-DPDP (Teslascan, GE Healthcare) for liver imaging and oral manganese chloride tetrahydrate for gastrointestinal imaging (LumenHance, Bracco Diagnostics) are available for human use.
Molecular MRI Contrast Agents
Molecular imaging is the visualization of biological processes at the cellular or subcellular level [20]. Imaging of the molecular pathways have the potential to transform the current treatment paradigms by providing accurate early diagnosis and treatment follow up which may form the basis of personalized medicine in cardiovascular, cancer and neurological diseases. MRI has the advantage of providing a comprehensive evaluation of cardiac morphology and function. New technical advances in high-resolution imaging can potentially make MRI an ideal modality for molecular imaging. Studies of carotid plaque imaging by MRI have documented the feasibility to delineate carotid plaque composition by characterizing lipid core, calcification and fibrous cap [21]. Most of carotid plaque characterization studies were conducted by using high-resolution imaging techniques with or without GBCAs, however, in vivo imaging of finer molecular processes require the use of molecular probes in the form of novel contrast agents. New contrast agents are being developed for molecular MRI that may aid in the detection of microthrombi or arterial plaques.
Lanza et al. developed a novel fibrin-specific MR contrast agent, which is a ligand-directed, lipid-encapsulated liquid perfluorocarbon nanoparticle (250 nm nominal diameter) that can detect and quantify occult microthrombi within the intimal surface of atherosclerotic vessels with high sensitivity [22]. In subsequent in vitro and in vivo (canine model of jugular vein thrombus) experiments using this nanoparticle formulated with Gd-DTPA-BOA, they found presence of nanoparticles on the surface of thrombi that produced a high signal (contrast enhancement) on T1-weighted imaging [23]. Their results suggested that molecular imaging of fibrin-targeted paramagnetic nanoparticles could provide sensitive detection and localization of fibrin with potential for early detection of vulnerable plaques.
Magnetic iron oxide nanoparticles constitute another class of molecular MRI agents also called novel ultrasmall paramagnetic iron oxide (USPIO) nanoparticles. They have a central core of iron oxide, which measures 3–5 nm in diameter surrounded by a dextran, starch, or polymer coat. Ferumoxytol is an example of USPIO used as blood pool agent (see above). Two USPIO have been approved for liver imaging, Feumoxides (Feridex) and Ferrixan (Resovist). There are other USPIO that are in various phases of development or clinical testing. Cross linked iron oxide (CLIO) nanoparticles have been specifically developed for molecular imaging and studied as molecular probes for imaging apoptosis and vascular cell adhesion molecule 1 (VCAM-1) [20]. Their small size and long circulating half-life allows them to penetrate atherosclerotic plaques or myocardial interstitial space.
Applications and Methods for Contrast Administration
As elaborated in other chapters, the use of GBCAs has become an integral component of cardiovascular MRI. Their use is particularly crucial in the diagnosis of cardiomyopathies, the assessment of myocardial viability, the evaluation of myocardial perfusion, the evaluation of cardiac masses and thrombi, the assessment of congenital heart disease, and the diagnosis of vascular abnormalities. Novel uses under development include the use of contrast agents in coronary artery imaging, T1 mapping for diffuse myocardial fibrosis, and MRI-guided cardiac interventions.
Dosing of GBCAs in the literature is variable and depends on the indication for its use. Standardized protocols have been published by the Society for Cardiovascular Magnetic Resonance Imaging and were recently updated in 2013 [24]. Contrast volume and injection rates depend upon the scan duration, type of contrast agents and specific imaging protocol (Tables 19.1 and 19.2).
Table 19.2
Contrast and chasing bolus doses and injection rates
Indication | Contrast dose (mmol/Kg body weight) | Injection rate | Saline chasing bolus (mL) | Injection rate (mL/s) |
---|---|---|---|---|
Perfusion | 0.05–0.1 | 3–7 mL/s | 30 | 3–7 |
Late gadolinium enhancement | 0.1–0.2 | 20 | ||
Angiography (carotids, renals, aorta) | 0.1–0.2 | 2–3 mL/s | 20 | 2–3 |
Time-resolved angiography | 0.005 | 3–5 mL/s | 30 | 3–5 |
Peripheral angiography | 0.2 | First 10 mL @ 1.5 mL/s, rest @ 0.4–0.8 mL/s | 20 | 0.4–0.8 |
Adverse Effects
GBCAs are generally considered safe with an exceptionally low incidence of adverse reactions. The frequency of all acute adverse events after an injection of a 0.1 or 0.2 mmol/kg dose ranges from 0.07 to 2.4 % [25]. The vast majority of reactions are considered mild as defined by the American College of Radiology (ACR), which includes coldness, warmth or pain at the injection site, nausea, vomiting, headache, flushing, dizziness and mild pruritus (Table 19.3) [26]. In a recent international multicenter registry study that assessed the safety of GBCAs in over 17,000 patients, only 30 acute adverse reactions occurred with an incidence of 0.17 %, all of which were mild [26]. The most frequent adverse reaction was rash/hives (0.05 %), followed by nausea (0.04 %), and anxiety (0.03 %). Reactions resembling an “allergic” response including urticaria and less frequently bronchospasm are very rare and occur with a frequency of 0.004–0.7 % [25]. Although there are reports of severe adverse reactions including life-threatening anaphylactoid reactions and death, they are exceptionally rare [27, 28]. The largest assessment of GBCA safety came in 2013 on the use of Gd-DTPA (Magnevist) in 120 million administrations which reported an incidence of severe reactions of only 0.003 % [28].
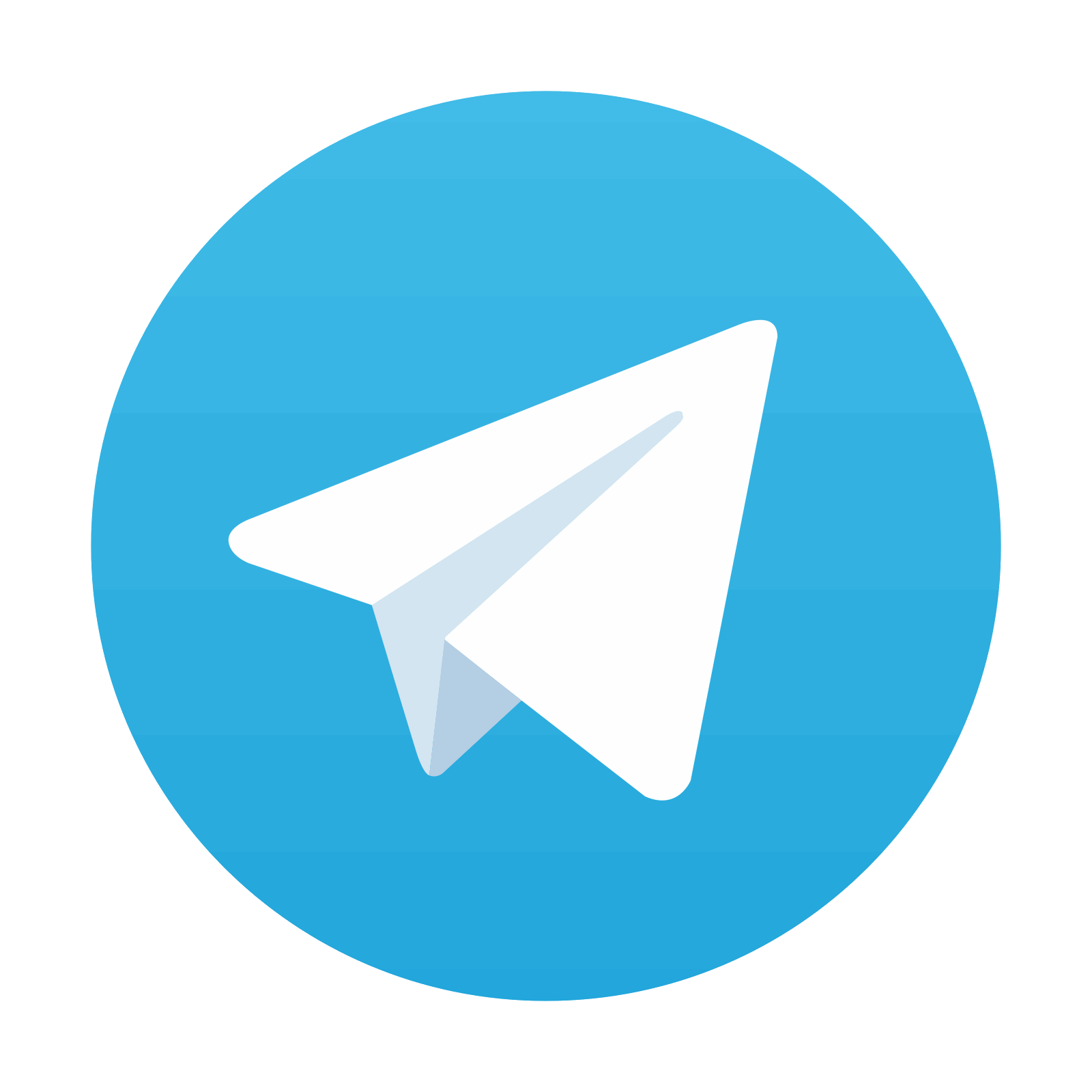
Table 19.3
Classification of severity and manifestation of adverse reactions to contrast media
< div class='tao-gold-member'>
Only gold members can continue reading. Log In or Register a > to continue
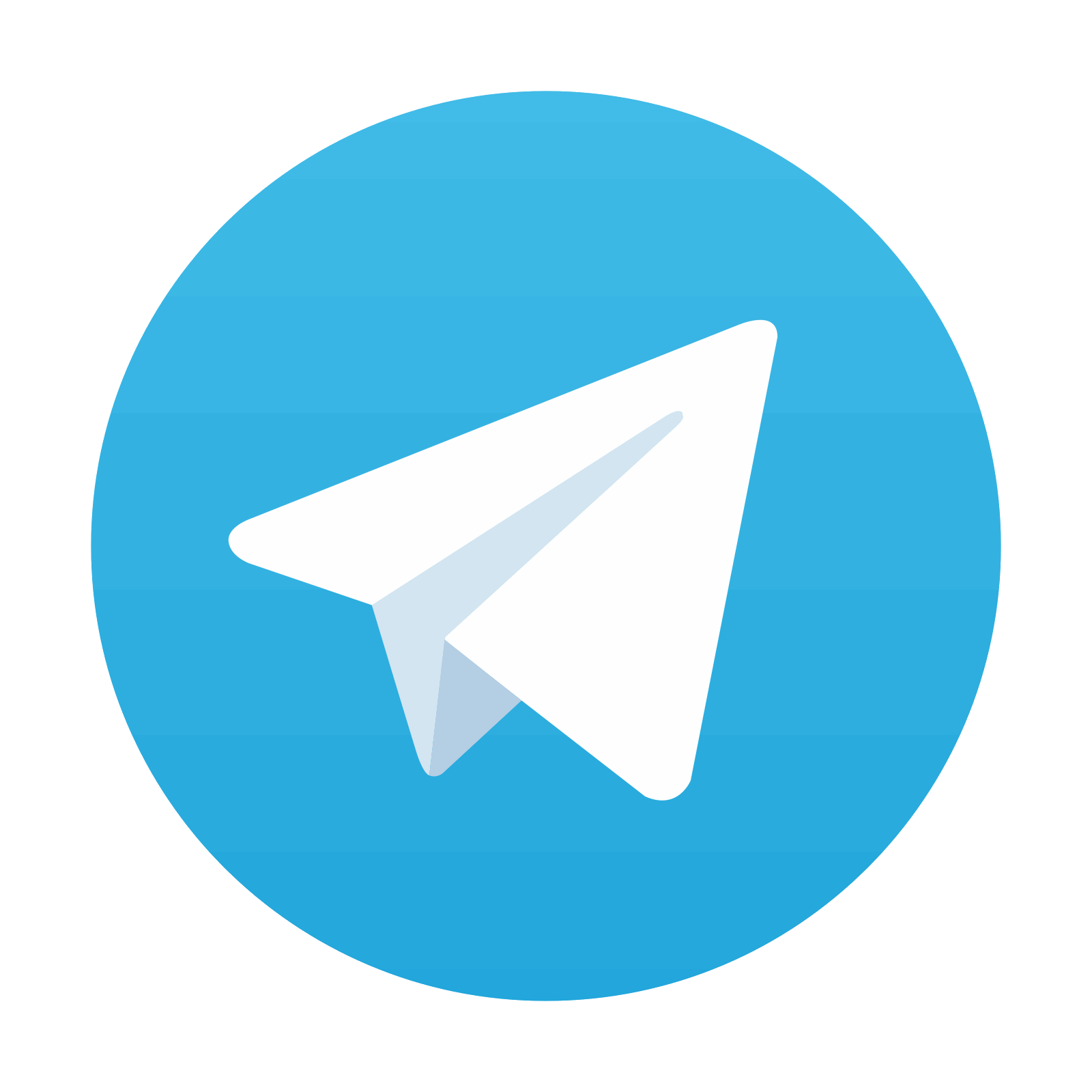
Stay updated, free articles. Join our Telegram channel

Full access? Get Clinical Tree
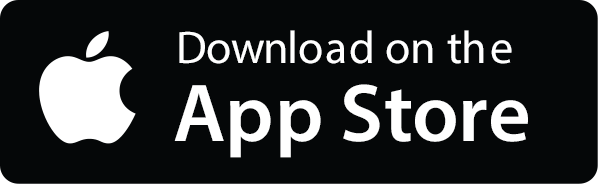
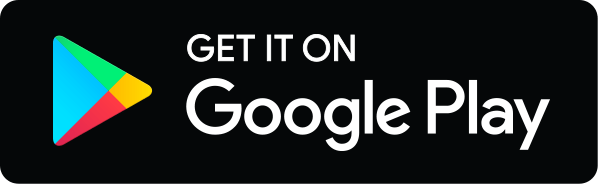
