Background
It has been suggested that the assessment of left ventricular contractile reserve during dobutamine infusion can improve prognostic evaluation for patients with idiopathic dilated cardiomyopathy (DCM). However, the assessment of contractile reserve is often subjective, while three-dimensional (3D) speckle-tracking can quantify three different components of strain simultaneously from all 16 left ventricular segments. The purpose of this study was to investigate the capability of multidirectional deformational indices assessed by 3D speckle-tracking strain to predict cardiac events in patients with DCM. Whether such assessment under dobutamine infusion can enhance this method’s predictive capability was also evaluated by comparison with two-dimensional (2D) speckle-tracking.
Methods
Sixty-five patients with DCM with a mean ejection fraction of 34 ± 8% (all <45%) were prospectively recruited, and both 3D and 2D speckle-tracking echocardiography was used to measure global circumferential strain (GCS), radial strain, and longitudinal strain at baseline and during dobutamine infusion (20 μg/kg/min). Event-free survival was then tracked over 12 months.
Results
Among all indices of contractile reserve, increase in 3D GCS under dobutamine infusion ≤ 2.71% best predicted cardiovascular events, with 83% sensitivity, 80% specificity, and an area under the curve of 0.874 ( P < .001). Furthermore, the area under the curve for increase in 3D GCS was significantly larger than that for both resting 3D GCS and increase in 2D GCS ( P < .05 for both). On multivariate Cox analysis, increase in 3D GCS was the only independent predictor of cardiovascular events (hazard ratio, 0.635; P < .001).
Conclusions
Assessment of contractile reserve using 3D GCS has the potential to predict cardiovascular events in patients with DCM and may thus have clinical implications for the management of such patients.
Idiopathic dilated cardiomyopathy (DCM) is characterized by progressive left ventricular (LV) dilation and depressed ventricular function. Despite remarkable progress in pharmacologic therapy, the overall prognosis of patients with DCM is still not satisfactory. It is thus important to identify patients with DCM with poor prognoses who are likely to experience clinical deterioration, because they may require more aggressive treatments and close monitoring as early as possible. Dobutamine echocardiography is widely used to assess myocardial viability and prognosis, and its safety and usefulness have been well established, while the direct assessment of contractile reserve may improve the prognostic evaluation of patients with heart failure (HF) with DCM. However, analysis of wall motion, such as the wall motion score index (WMSI) and ejection fraction (EF) during dobutamine infusion, is subjective as well as highly load dependent, and its accuracy depends largely on the experience of the operators.
Recently, global circumferential strain (GCS) by means of two-dimensional (2D) speckle-tracking has been introduced as a powerful predictor of cardiac events and is reported to be a better parameter than EF in patients with HF. However, this method is limited to 2D tomographic imaging planes, which may oversimplify the complexities of LV function in individual patients. A newly developed three-dimensional (3D) speckle-tracking system, on the other hand, can quantify LV myocardial function from complete 3D data sets and can be used for quantitative assessment of LV performance. Moreover, this novel 3D speckle-tracking system can evaluate three different components of strain simultaneously. Accordingly, our objective was to investigate the capability to assess these deformational indices using 3D speckle-tracking strain to predict cardiovascular events in patients with HF with DCM and whether an assessment of these parameters combined with dobutamine echocardiography can further enhance the predictive capability of this method. Furthermore, we evaluated the prognostic utility of 3D speckle-tracking strain analysis by comparison with 2D strain measurements.
Methods
Study Population
Seventy-two consecutive patients with DCM were prospectively recruited for this study. Four of these patients (6%) were excluded from all subsequent analyses because of suboptimal quality of images, defined as more than three nonassessable segments, and we also excluded three patients (4%) with atrial fibrillation. The final study group thus consisted of 65 patients. The diagnosis of DCM was established with the following criteria: (1) presence of LV dilation (LV end-diastolic diameter ≥ 55 mm); (2) reduced LV EF (all <45%); (3) coronary angiographic evidence of absence of coronary artery disease, defined as >50% stenosis of a major epicardial vessel or a history of myocardial infarction; (4) absence of primary valvular heart disease; and (5) absence of cardiac muscle disease secondary to any known systemic diseases. At the time of enrollment, all patients were in clinically stable condition and on optimal and maximally tolerated pharmacologic therapy. This study was approved by the local ethics committee of our institution, and written informed consent was obtained from all patients.
Two-Dimensional Echocardiographic Examination
Two-dimensional echocardiography was performed using a commercially available ultrasound system (Aplio Artida; Toshiba Medical Systems, Tochigi, Japan). LV stroke volume was obtained by means of the velocity-time integral, assessed by pulsed Doppler positioned at the LV outflow tract. Cardiac output was obtained by multiplying stroke volume by heart rate and cardiac index by dividing cardiac output by body surface area. The pulsed-wave Doppler–derived transmitral velocity and digital color tissue Doppler–derived mitral annular velocity were obtained from apical four-chamber views for assessment of diastolic function. The early diastolic (E) and atrial (A) wave velocities as well as the E-wave deceleration time were measured using pulsed-wave Doppler recording. Tissue Doppler–derived early diastolic velocity (E′) was obtained from the septal mitral annulus, and the E/E′ ratio was calculated to estimate LV filling pressure. The WMSI was also assessed using the recommendations of the American Society of Echocardiography. Left atrial (LA) borders were traced using planimetry, and the biplane method of disks was used to calculate LA volume. The LA volume index was then calculated by dividing LA volume by body surface area. All these measurements were averaged from three cardiac cycles.
Two-Dimensional Speckle-Tracking Strain Analysis
Two-dimensional speckle-tracking strain was assessed for each patient with the aid of dedicated software (Ultra Extend; Toshiba Medical Systems), as described previously in detail. Briefly, radial and circumferential strains were assessed from the mid-LV short-axis view. LV myocardium was automatically divided into six standard segments, and time-strain curves were generated from each segment. The average values of strain at each time point was calculated as global strain, and peak global strain values for radial and circumferential components were defined as peak global radial strain (GRS) and GCS, respectively. Each apical image was divided into six LV segments, and corresponding time-strain curves were generated. The average values of strain at each time point was calculated as global strain from each apical view. Then, averaged values from three apical views were calculated as global longitudinal strain.
Three-Dimensional Speckle-Tracking Analysis
All 3D echocardiographic studies were performed using a 2.5-MHz 3D matrix-array transducer (Toshiba Medical Systems). Digital 3D volume data were obtained from the apical view by means of six-beat electrocardiographically gated acquisition at baseline and during peak dobutamine infusion. All 3D images were obtained during a breath hold to avoid stitching artifacts. Special care was taken to include the entire LV cavity within the pyramidal volume using wide-angled acquisition with 90° to 110° × 90° to 110°. The mean volume rate was 21 ± 5 volumes/sec for grayscale imaging used for 3D speckle-tracking analysis. Digital data were transferred to dedicated software (Ultra Extend) for all subsequent analyses. Three-dimensional speckle-tracking analysis was performed as previously detailed. Briefly, the 3D data sets were displayed in five different cross-sections, including three standard short-axis views and apical four-chamber and two-chamber views that could be modified interactively ( Figure 1 ). Regions of interest were placed on the endocardium and epicardium, and the software automatically tracked the endocardial and epicardial contours in the subsequent volumes. On the basis of these contours, LV end-diastolic volume and end-systolic volume could then be obtained as the respective maximum and minimum values, and EF was also obtained automatically. The software automatically divided the left ventricle into 16 standard segments and generated corresponding time-strain curves from each segment ( Figure 1 ). Three-dimensional circumferential, radial, and longitudinal myocardial functions were quantified as global peak strains (3D GCS, 3D GRS, and 3D GLS, respectively) using 3D speckle-tracking strain results from all 16 LV segments ( Figure 2 ).


Dobutamine Infusion Protocol
All patients underwent dobutamine stress echocardiography in incremental stages lasting 5 min each, with an initial dose of 5 μg/kg/min, which was increased to 10 μg/kg/min, and finally to the maximal dose of 20 μg/kg/min for the assessment of LV contractile reserve. Electrocardiography, blood pressure, and heart rate were recorded during the entire procedure. The infusion of dobutamine was discontinued before the maximal dose was reached if symptomatic nonsustained or sustained ventricular tachycardia was observed. LV WMSI, LV EF, and three components of both 2D and 3D global LV strain were evaluated as indices of LV contractility. Contractile reserve was defined as the difference between the baseline values and their values obtained at peak dobutamine dose during the test (Δ).
Definitions of Long-Term Outcome Analysis
Long-term unfavorable outcome events were prespecified as primary end points of death from or hospitalization for deteriorating HF and sudden cardiac death. Long-term follow-up was performed for 12.0 ± 8.1 months after enrollment.
Analysis of Reproducibility
The interobserver and intraobserver variability of contractile reserve indices was expressed as the absolute difference between the measurements divided by their mean value from 10 randomly selected patients. The intraclass correlation coefficient was also used to determine interobserver and intraobserver reproducibility from 10 randomly selected subjects. For the assessment of intraobserver reproducibility, measurements of these patients were analyzed using the same data set on two consecutive days, by an observer blinded to the results of the previous measurements. For the assessment of interobserver reproducibility, measurements for selected patients were analyzed by the second observer, using the same data set used for the analysis of intraobserver reproducibility, blinded to the values obtained by the first observer. Moreover, for the assessment of repeatability, measurements of these patients were reanalyzed using the different data sets ≥2 weeks apart, by a first observer blinded to the results of the previous measurements.
Statistical Analysis
Continuous variables are expressed as mean ± SD or as percentages, while categorical data are summarized as frequencies and percentages. We divided the patients into two subgroups on the basis of whether they did or did not develop adverse cardiovascular events during the follow-up period. The parameters of the two subgroups were compared using the unpaired t test, and the paired t test was used for comparison of continuous variables before and during peak dobutamine infusion. Proportional differences were evaluated by using Fisher’s exact test or the χ 2 test as appropriate. Receiver operating characteristic (ROC) curves were computed to determine optimal cutoff points for dobutamine-induced changes in the examined LV contractility indices as well as to calculate the area under the curve (AUC) for each of the indices to determine their prognostic significance. After performing the initial univariate Cox proportional-hazards regression analysis to identify univariate predictors of cardiovascular events, we proceeded with a multivariate Cox model on the basis of stepwise selection, with the P levels for entry into and removal from the model set at .05 and .10, respectively. For all steps, P values < .05 were considered statistically significant. SPSS version 13.0 (SPSS, Inc., Chicago, IL) was used for all calculations.
Results
Baseline Characteristics
Of the 65 patients who met all inclusion and exclusion criteria, none were lost to follow-up. During the follow-up period of 12.0 ± 8.1 months, predefined cardiovascular events occurred in 12 patients (18.5%). Of these patients, one patient died of HF, two patients experienced sudden cardiac death, and the remaining nine patients were admitted for worsening HF. The baseline clinical, hemodynamic, and echocardiographic characteristics of these patients are presented in Table 1 . Compared with patients without cardiovascular events, patients with DCM with cardiovascular events were older and were more often prescribed loop diuretics, reflecting more severe HF at baseline. Although no significant differences were noted in hemodynamic parameters between the two groups, patients with cardiovascular events were more likely to have higher transmitral E/A ratios and larger LA volumes, suggesting a greater elevation of LA pressure. Interestingly, of the 2D and 3D speckle-tracking strain parameters, only 3D GCS was found to be smaller in patients with idiopathic DCM with cardiovascular events ( Table 1 ). The results of reproducibility analysis are shown in Table 2 .
Variable | All patients ( n = 65) | Cardiovascular events | P | |
---|---|---|---|---|
Patients without cardiovascular events ( n = 53) | Patients with cardiovascular events ( n = 12) | |||
Age (y) | 58 ± 17 | 55 ± 18 | 68 ± 10 | <.05 |
Men/women | 40/25 | 30/23 | 10/2 | NS |
BSA (m 2 ) | 1.61 ± 0.20 | 1.61 ± 0.20 | 1.61 ± 0.20 | NS |
Hemodynamics | ||||
Systolic BP (mm Hg) | 102 ± 18 | 103 ± 18 | 100 ± 18 | NS |
Diastolic BP (mm Hg) | 57 ± 14 | 58 ± 14 | 53 ± 13 | NS |
Heart rate (beats/min) | 66 ± 12.4 | 65 ± 12 | 71 ± 12 | NS |
Stroke volume (mL) | 65 ± 19 | 67 ± 19 | 58 ± 18 | NS |
Cardiac index (L/min/m 2 ) | 2.63 ± 0.73 | 2.64 ± 0.71 | 2.57 ± 0.85 | NS |
QRS duration (msec) | 110.0 ± 24.0 | 109.4 ± 23.7 | 112.3 ± 26.5 | NS |
NYHA functional class | ||||
II | 55 (85%) | 47 (89%) | 8 (67%) | NS |
III | 10 (15%) | 6 (11%) | 4 (33%) | NS |
Medication | ||||
Loop diuretics | 39 (60%) | 28 (53%) | 11 (92%) | <.05 |
β-blockers | 64 (98%) | 52 (98%) | 12 (100%) | NS |
Spironolactone | 34 (52%) | 26 (49%) | 8 (67%) | NS |
ACE inhibitors/ARBs | 54 (100%) | 45 (100%) | 10 (100%) | NS |
LV volume index (mL/m 2 ) | ||||
End-diastole | 101.6 ± 32.7 | 100.0 ± 32.4 | 108.5 ± 34.6 | NS |
End-systole | 67.9 ± 28.3 | 65.2 ± 26.6 | 79.1 ± 33.2 | NS |
LV EF (%) | 33.8 ± 8.4 | 34.9 ± 8.0 | 29.0 ± 8.8 | <.05 |
LA volume index (mL/m 2 ) | 54.7 ± 19.4 | 51.2 ± 16.3 | 69.4 ± 25.1 | <.01 |
Transmitral flow | ||||
E/A ratio | 1.29 ± 0.96 | 1.15 ± 0.63 | 1.89 ± 1.70 | <.05 |
Deceleration time (msec) | 219.5 ± 80.0 | 222.2 ± 72.2 | 207.8 ± 110.7 | NS |
Tissue Doppler | ||||
E′ (cm/sec) | 6.0 ± 2.1 | 6.1 ± 2.1 | 5.9 ± 2.0 | NS |
E/E′ ratio | 11.7 ± 5.9 | 11.5 ± 5.7 | 12.7 ± 6.8 | NS |
2D global strain (%) | ||||
GRS | 15.2 ± 8.3 | 15.6 ± 8.3 | 13.6 ± 8.1 | NS |
GCS | −7.2 ± 3.0 | −7.6 ± 3.1 | −5.8 ± 1.9 | NS |
GLS | −7.6 ± 2.5 | −7.8 ± 2.6 | −6.8 ± 2.2 | NS |
3D global strain (%) | ||||
GRS | 14.2 ± 5.9 | 14.8 ± 6.0 | 11.4 ± 4.7 | NS |
GCS | −13.6 ± 4.6 | −14.2 ± 4.6 | −11.1 ± 3.9 | <.05 |
GLS | −9.4 ± 2.6 | −9.5 ± 2.4 | −8.7 ± 3.2 | NS |
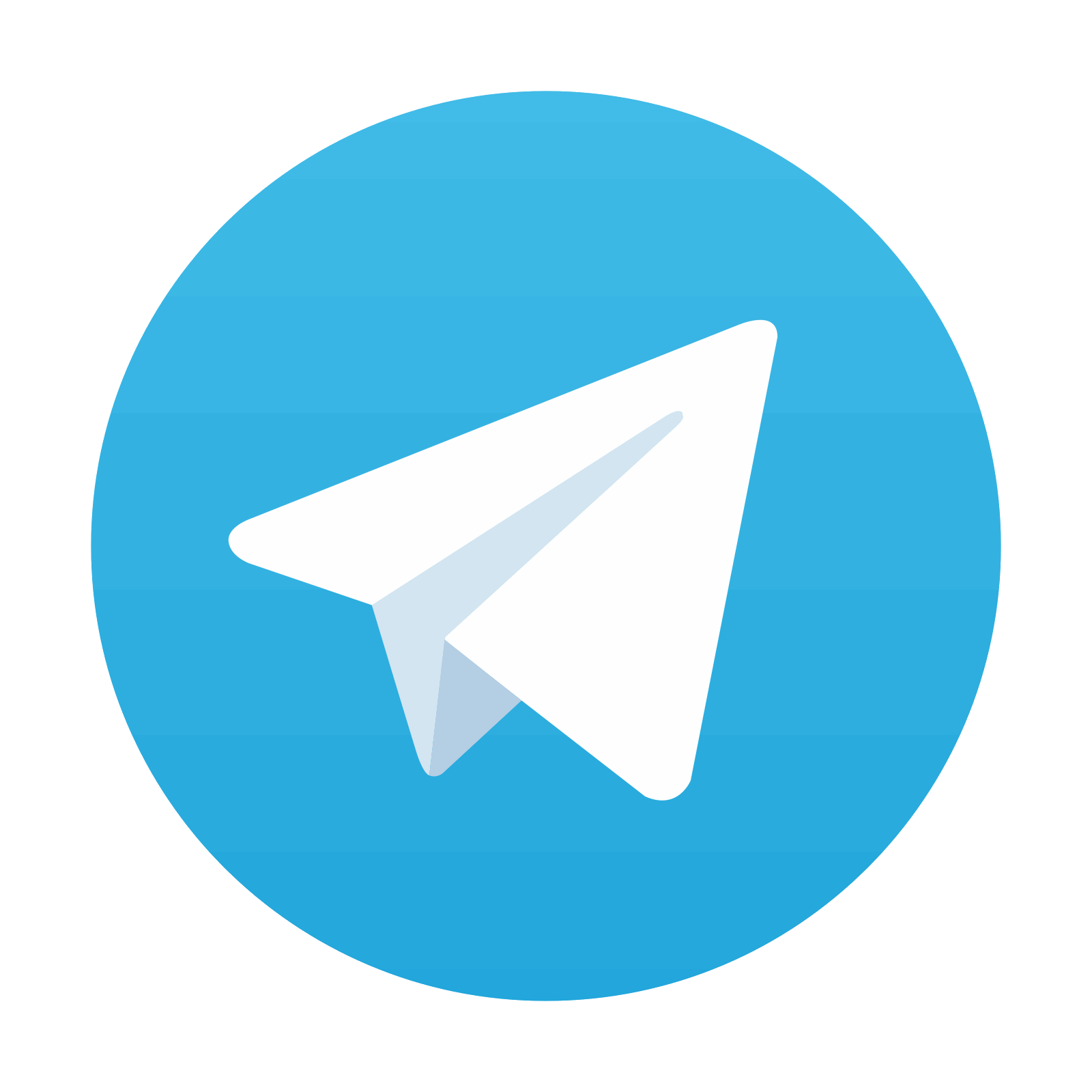
Stay updated, free articles. Join our Telegram channel

Full access? Get Clinical Tree
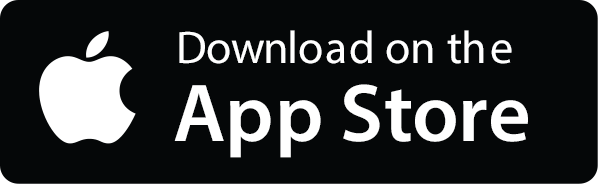
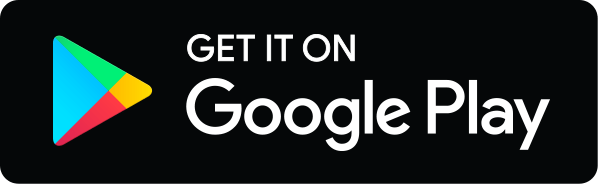
