Fig. 16.1
Pulmonary artery smooth muscle cell therapeutic targets and corresponding licensed (green boxes) and investigational (red boxes) treatment approaches for pulmonary arterial hypertension. Arrows represent receptor stimulation, whereas terminated lines show receptor blockade. Abbreviations: AC adenylate cyclase, cAMP cyclic AMP, cGMP cyclic GMP, ECE–1 endothelin converting enzyme 1, eNOS endothelial nitric oxide synthase, ETA endothelin receptor type A, ETB endothelin receptor type B, ERA endothelin receptor antagonists, IP prostaglandin I2, PDE–5 phosphodiesterase type 5, PDGF platelet derived growth factor, PDGF–R TKI PDGF receptor tyrosine kinase inhibitors, PGIS prostaglandin I synthase, sGC soluble guanylate cyclase, VPAC vasointestinal peptide receptor (This figure is reproduced with permission from O’Callaghan et al. [115])
Although the exact mechanisms that leads to the development and progression of idiopathic PAH remain largely elusive, there are histologic features of the disease that are common in most cases of chronic PAH [2]. Environmental factors acting through genetic mediators likely precipitate varying degrees of vasoconstriction, vascular and cellular proliferation, thrombosis, and chronic inflammation which indicate pathologic remodeling [3, 4]. The pulmonary arterial vascular system is normally a high-output circulation with low pressure and low resistance. The high vascular compliance of the pulmonary vasculature leads to a small increase in pulmonary artery pressure with increases in cardiac output that occur with exercise. Regulation of vascular tone is mediated by intracellular calcium which is influenced by adrenergic factors, calcium-dependent potassium channels, and local environmental factors (i.e. hypoxia) [5]. While fewer than 15 % of PAH patients are acutely responsive to vasodilator therapy, inappropriate pulmonary vasoconstriction likely contributes to PAH pathophysiology to some extent [6].
The remodeling process in PAH is marked by dysregulation of cellular proliferation, apoptosis, and angiogenesis, with some similarities to the pathologic findings in certain malignancies [7, 8]. A series of growth factors and cytokines trigger intracellular signaling pathways that alter nuclear protein expression and ultimately lead to a constitutive growth process [9–11]. PAH is a vasculitic disorder that is also notable for intraluminal thrombosis of the medium to small pulmonary arteries as noted on histologic examination [12]. Thrombin, in the setting of stressors and ongoing inflammation, appears to be a central mediator in the propagation of thrombosis [13]. Thrombin activation also leads to mitogenic and angiogenic effects in addition to direct platelet activation, potentiating the coagulation cascade [12–14].
Endothelial cells act as a central mediator in the regulation of pulmonary vascular tone through vasoactive substances, which ultimately alter pulmonary smooth muscle cells (PSMCs) [15]. In the setting of continuous or excessive activation, PSMCs demonstrate pathologic and eventually irreversible growth, proliferation, and contraction [3, 16, 17]. Prostacyclin (prostaglandin I2, PGI2), through the activation of cyclic guanosine monophosphate (cGMP) pathways, promotes vasodilation while inhibiting PSMC proliferation and platelet aggregation [15, 18]. This endogenous, potent vasodilator is decreased in idiopathic PAH patients, a finding supported by decreased expression of prostacyclin synthase and stable metabolites of PGI2 noted in the endothelial cells [19, 20]. Nitric oxide (NO) is also an important mediator of vasodilation by raising cGMP intracellularly [21]. NO release occurs predominantly via the endothelial cells acting in a paracrine manner on the adjacent PSMCs [21, 22]. However, the extent of NO availability is complex with shear stress, thrombin formation, vascular injury, and toxins all mediating NO release and metabolism [23–25]. Endothelin-1 (ET-1), secreted by vascular endothelial cells, acts on endothelin receptor A (ETA) and B (ETB) in PSMCs to further trigger vasoconstriction and cellular proliferation [18, 26]. Additionally, the regulation of intracellular calcium through a variety of triggers and mechanisms involving local kinases and phosphatases regulates vascular tone and remodeling potential [3, 27].
Pulmonary vascular inflammation may occur in response to physical, immune, toxic, or infectious injury that initiates the recruitment of inflammatory cells. These inflammatory cells act as central propagators through immune-mediated mechanisms in an effort to resolve the perceived injury [28, 29]. Chronic inflammation appears central to the maladaptive remodeling in PAH, which is, in part, directed by the dysregulation of the immune response [30]. As mentioned above, a host of inflammatory cytokines/chemokines and growth factors can induce the proliferation and migration of endothelial and PSMCs [31, 32]. Thus, it is not entirely surprising that higher levels of inflammatory mediators have been associated with worse outcomes in PAH patients [33–37].
Central to the pathobiologic changes in PAH is a genetic predisposition to vascular and cellular injury leading to the cascade of events discussed above. Over the past two decades, the development of next-generation sequencing techniques, genome-wide association studies, carefully designed familial studies, and longitudinal data from registries have enhanced the understanding of the hereditary components of PAH [38]. As genomic research continues in the field of PAH, there will be a continued effort to define the genomics, metabolomics, and proteomics for a more robust genetic description of the disease process and predisposition to pathologic changes [39, 40]. For instance, genetic linkage analyses of patients with a known family history of PAH have elucidated the importance of the transforming growth factor beta (TGF-β) receptor and downstream signaling pathways in the pathobiologic processes [41]. Overall, the continued investigation of possible infectious, inflammatory, hypoxic, and toxic environmental triggers on a background of various genetic and genomic underpinnings will further our understanding of the complexities of PAH pathobiology and lead to additional, novel therapeutic targets.
Therapeutic Targets
Prostacyclins (Table 16.1)
Table 16.1
Pharmacologic and clinical points for prostacyclins in the treatment of PAH
Prostacyclin agent | Mechanism of action | Administration route(s) | Suggested dosing | Relative & absolute contraindications | Monitoring & side effects |
---|---|---|---|---|---|
Epoprostenol (Flolan™) | Prostaglandin I2 (PGI2), Prostacyclin analogue Pulmonary and systemic vasodilation Inhibition of platelet aggregation Anti-proliferative properties | Intravenous via continuous pump delivery | Initiation: 1–2 ng/kg/min Titration: 1–2 ng/kg/min daily while in the hospital for 3–5 days; weekly as outpatient Chronic: Average dose 30–50 ng/kg/min at 1 year | Avoid abrupt discontinuation which may lead to acute rebound pulmonary hypertension and cardiogenic shock Inability to reliably manage an indwelling catheter and pump delivery system | Catheter–related: Sepsis, thrombosis Drug–related: Hypotension, nausea, vomiting, jaw pain, headache, flushing |
Treprostinil (Remodulin™) (Tyvaso™) (Orenitram™) | Tricyclic benzidine analog of epoprostenol | Intravenous, Subcutaneous, Inhaled, Oral | SQ: Initiation: 2 ng/kg/min Titration: 2 ng/kg/min 1–2 per week as outpatient Chronic: Average dose 2–3× epoprostenol (i.e. 60–80 ng/kg/min) Inhaled: 3 breaths (18 mcg) 4 times per day; goal 9 breaths (54 mcg) per session (4 times daily); increase by 3 breaths per session every 1–2 weeks Oral: 0.25 mg BID; increase by 0.25 mg per dose every 3–4 days; average dose 4.2 mg BID at 1 year | IV treprostnil: See epoprostenol Oral treprostinil contraindicated in severe hepatic impairment (Child-Pugh Class C or greater) | See epoprostenol Intravenous treprostinil associated with increased risk of gram-negative bacteremia Severe site pain associated with SC Cough and throat irritation with inhaled PO administration less risk of systemic effects but frequent GI upset including nausea and diarrhea |
Iloprost (Ventavis™) | Stable prostacyclin analog | Inhaled, Intravenous | Inhaled: 2.5 mg per inhalation 6–9 times per day; titrate to goal 5 mg per dose at least 6 times per day; do not exceed 70 mg daily | See epoprostenol Monitor closely with advanced hepatic or renal disease | See epoprostenol Additional risk of cough and throat irritation with inhalation |
Among the pathways disrupted in the pathobiology of PAH is the prostacyclin pathway leading to a relative deficiency of endogenous prostacyclin synthesis. Prostacyclins and prostacyclin analogues (prostanoids) primarily target the prostaglandin I receptor activating adenylate cyclase activity, which results in potent pulmonary (but also systemic) vasodilation, inhibition of platelet aggregation, and a blunting of certain inflammatory responses that would otherwise trigger endothelial and smooth muscle cell proliferation [15, 42, 43]. Over the past two decades, prostacyclin, the first approved therapy for PAH, has maintained its role as a cornerstone of therapy in patients with progressive PAH. The evolution of prostacyclin therapies since the original approval of intravenous epoprostenol includes the development and approval of alternative intravenous, subcutaneous, inhaled, and oral formulations. We will review the various prostacyclin therapies with a focus on landmark clinical trials, important pharmaceutical properties, and an approach to dosing while monitoring for adverse effects and toxicity.
Parenteral Therapies: Intravenous and Subcutaneous
Intravenous Epoprostenol (Flolan™)
Epoprostenol is the intravenous formulation of prostacyclin I2 (PGI2), a synthetic, stabilized compound initially approved by the FDA in 1995 for idiopathic PAH. As such, the most robust longitudinal and mortality data are available for this compound. Following a number of encouraging, smaller clinical trials of prostacyclin therapy in PAH [44–47], a pivotal, 12-week, prospective, placebo-controlled randomized trial evaluating hemodynamics, functional capacity, quality of life, and survival in 81 patients with New York Heart Association functional class (FC) III or IV idiopathic PAH (IPAH) (previously known as primary pulmonary hypertension) was performed. Patients receiving epoprostenol demonstrated significant improvements in pulmonary vascular resistance, pulmonary artery pressure, and cardiac index with marginal effects on mean systemic arterial pressure. Additionally, 6-min walk distance (6MWD) and quality of life scores improved in the epoprostenol-treated participants compared to the placebo group, with the placebo patients experiencing a worsening of quality of life and 6MWD. There were no deaths in the epoprostenol group compared to eight deaths in the placebo arm [47]. Subsequent to this trial in IPAH, similar hemodynamic and functional improvements were observed in scleroderma-related PAH treated with IV epoprostenol over a 12-week period but with similar survival rates in the IV epoprostenol group compared to the conventional therapy group [48].
Since the completion of the key epoprostenol PAH clinical trials, three additional, observational studies have compared survival in those treated with epoprostenol to historical controls from the original US National Institutes of Health registry of PAH [49, 50]. In the first study, Shapiro and colleagues prospectively followed 69 patients with FC III and IV idiopathic PAH at a single center and observed an improved survival at 1, 2, and 3 years respectively as compared to historical controls [51]. Those subjects followed for more than 1 year had additional improvements in non-invasively assessed pulmonary vascular resistance (PVR) indices, suggesting a long-term hemodynamic benefit of the therapy [51]. Similarly, a survival benefit from epoprostenol was observed by McLaughlin and colleagues in 162 patients PAH with functional class III or IV treated at a single center. With a median follow up of 31 months, a substantial survival benefit was observed in those on epoprostenol therapy at 1 year, 2 years, 3 years, and 5 years, respectively. Long-term improvements in symptoms and invasively measured hemodynamic parameters were demonstrated as well [52]. Around the same time, Sitbon and colleagues reported similar findings of improved symptoms, hemodynamics and survival at 1, 2, 3, and 5 years, respectively, in a French PAH cohort [53].
Pharmacologic Features and Clinical Considerations
The chemical and pharmacokinetic properties of IV epoprostenol necessitate the intricate delivery system for the drug. Epoprostenol is prepared in sterile, freeze-dried powder which requires cool storage recommended at 15 to 20 °C. The half-life of the drug is 6 min as the drug is rapidly degraded in the blood and ultimately renally excreted. Therefore, the drug must be delivered via continuous infusion with a central catheter via a positive pressure pump (Fig. 16.2); abrupt discontinuation of therapy may lead to cardiovascular collapse and death [54]. In the hospital setting in the treatment naïve patient, epoprostenol is typically initiated via a peripherally inserted central catheter or central line. As the transition to outpatient care occurs, the placement a durable, tunneled central venous catheter is strongly advised. Additionally, the intravenous pump is converted to a battery operated portable pump (Fig. 16.2). Veletri™, a generic formulation of epoprostenol (Flolan™), is stable at room temperature and gained FDA approval in 2012. This l-arginine formulation purportedly provides a more thermally stable preparation with an administration time of up to 7 days after mixing the drug without a compromise in potency [55, 56].
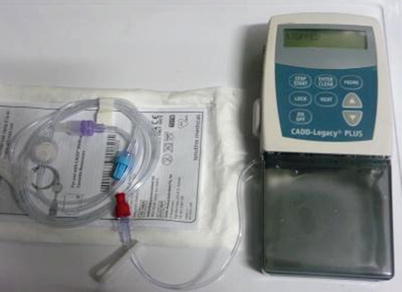
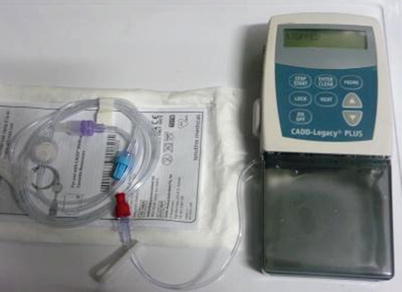
Fig. 16.2
Pictured is an actual portable, battery-powered infusion pump into which a drug cassette of epoprostenol is inserted. This set-up allows for the self-administration of a continuous infusion of prostacyclin therapy via a single lumen central venous catheter (often a Hickman catheter) (This figure is reproduced with permission from Cheryl Switzer @ PHCentral.org http://www.phcentral.org/medical/flolan-resource-page (Accessed January 2015))
The continuous nature of the drug infusion via central venous access, the associated equipment, and the required meticulous care can be a substantial barrier for some patients. Extensive education is required with documented competency in pump management (including alarms), sterile technique, medication preparation and storage, and awareness of pump or catheter related complications (including sepsis and thrombosis) [57]. As such, the administration and longitudinal follow up care for patients on parenteral therapy including epoprostenol should be coordinated by an experienced integrated health center expert in the management of PAH.
Dosing Recommendations and Adverse Events
Given the variability and relatively short follow-up duration in clinical trials, dosing recommendations for the long-term use of epoprostenol therapy comes from empiric clinical experience. Traditionally, epoprostenol has been initiated at a dose of 2 ng/kg/min and titrated daily to a dose that results in the initial optimization of PAH symptoms and hemodynamics while limiting side effects. In the pivotal trial by Barst and colleagues, a mean dose of 9 ng/kg/min was achieved at 8 weeks [47]. In the later studies by McLaughlin et al. and Sitbon et al., mean doses of approximately 22–27 ng/kg/min and 14 ng/kg/min respectively at 12 weeks were achieved [52, 53, 58]. Recently, McLaughlin and Palevsky provided a contemporary dosing recommendation for the initiation and dose escalation for intravenous epoprostenol therapy targeting an eventual average dose of 35–50 ng/kg/min at 1 year [57].
The off-target effect profiles are similar for all forms of prostacyclin therapies with a few exceptions. With the slow initiation of therapy, systemic vasodilation due to epoprostenol may induce a modest decrease in systemic blood pressure in addition to common side effects including flushing, headache, diarrhea, and a specific jaw discomfort that occurs with the initial mastication during a meal [52, 53]. In overdose states, patients may report extreme side effects with a predominance of diarrhea and flushing and invasive hemodynamic monitoring may even demonstrate a high cardiac output state [58]. However, many of these side effects will abate as a steady state dose is achieved and the drug is used chronically. In addition, there are potential adverse events associated with the intravenous delivery system. As with any catheter-based system, the patient and physician must remain vigilant in the monitoring of thrombotic complications and perhaps more commonly, local or systemic infections [57].
Subcutaneous and Intravenous Treprostinil (Remodulin™)
Treprostinil was the second prostacyclin therapy approved by the FDA in 2002, initially via the subcutaneous route. The subcutaneous delivery of treprostinil provided an alternative, parenteral route of prostanoid delivery given some of the limitations related to intravenous therapy. The main clinical data examining the safety and efficacy of subcutaneous treprostinil comes from a single placebo-controlled trial and a subsequent observational study. Simonneau and colleagues conducted a 12 week, multinational, double-blinded trial in four-hundred and seventy patients with PAH. The primary endpoint of 6MWD improved significantly in those treated with SC treprostinil and secondary outcomes of dyspnea scores and hemodynamic parameters also improved [59]. This study was followed by an observational study of eight-hundred sixty patients by Barst and colleagues, providing longer term follow-up data on safety and survival. Participants were followed for up to 4 years with Kaplan-Meier derived survival estimates compared to the NIH predicted mortality and suggested possible improved survival on therapy [60]. Following the approval of SC epoprostenol, the safety and efficacy of IV treprostinil was demonstrated in a prospective, open-label, 12-week trial of 16 patients with mixed etiologies of PAH (8 with idiopathic) in whom improvements in 6MWD, dyspnea scores, and hemodynamics were observed [61].
Pharmacologic Features and Clinical Considerations
The longer half-life, thermal stability at room temperature, and avoidance of a central catheter delivery system were all leveraged when developing the subcutaneous formulation. Treprostinil is a thermally stable tricyclic benzene analog of prostacyclin with peak plasma concentrations of the drug occurring within 2 to 3 h of subcutaneous delivery [62, 63]. Treprostinil also has a longer half-life of approximately 4.5 h as compared to epoprostenol. The longer half-life may mitigate the risk of cardiovascular collapse that may be seen with the acute discontinuation of epoprostenol [64, 65]. Similar to the intravenous formulations of prostacyclin therapies, the subcutaneous delivery is via a positive pressure infusion pump but with a self-inserted catheter. The initiation of subcutaneous treprostinil can be performed in the inpatient or outpatient setting with close monitoring. The initial FDA approved dose is recommended at 1.25 ng/kg/min, however there is clinical data suggesting the safety of a 2.5 ng/kg/min initial dose with rapid escalation upon demonstrating improved clinical and hemodynamic endpoints compared to a slower escalation strategy beginning at a dose of 2 ng/kg/min. When studied, those participants in the rapid titration group had a higher average dose of approximately 20 ng/kg/min at 12 weeks [59]. Particular attention to early pump and site complications should be noted by the patient and alert the care team as soon as possible [54]. Contrary to other subcutaneously-based therapies, a specific infusion pump site is not required for appropriate absorption: the abdomen is the preferred site although the buttock, flank, thigh, and upper arm are potential alternative sites. Although rotation of the administration site is recommended to occur at three day intervals, patients will often administer the drug at the same site for up to 30 days and typically rotate the site every 2–4 weeks without obvious detrimental effects. Additionally, the transition from SC to IV treprostinil (and vice versa) can be generally done safely without deterioration in clinical status as long as it is performed in an appropriate clinical setting and under expert supervision [66, 67].
Dosing Recommendations and Adverse Events
As with IV epoprostenol, IV treprostinil is typically initiated in the hospital setting at an initial dose of 2 ng/kg/min with incremental increases of 2 ng/kg/min titrated to hemodynamic and clinical endpoints and limited by tolerability. The ultimate, average dose of treprostinil achieved will typically be about two to three-times the dose of epoprostenol [61, 63]. The side effects of IV and SC treprostinil are similar to that of epoprostenol including flushing, headache, diarrhea, and jaw discomfort [54, 59, 60]. With SC treprostinil, perhaps the most limiting side effect is severe site pain, which may occur in up to 85 % of patients. Rarely, abscess formation may occur at the infusion site requiring local drainage and systemic antibiotics. With IV treprostinil, patients may be at higher risk of bacteremia, particularly gram negative rod sepsis as compared to IV epoprostenol. The concern of gram-negative systemic infections were noted in an examination of the REVEAL registry (Registry to Evaluate Early and Long Term PAH Disease Management) that demonstrated a rate of 0.36 vs. 0.12 per 1000 treatment days compared to epoprostenol therapy. A subsequent multivariate analysis of the data adjusting for potential confounders reported an approximately 3- and 6-fold increase in the risk of bloodstream infections and gram-negative infections respectively with IV treprostinil when compared to epoprostenol [68]. Interestingly, a subsequent study demonstrated that the increased risk of gram negative bacteremia may be attenuated when epoprostenol diluent is used with IV treprostinil administration, perhaps best explained by differences in the pH between the respective diluents [69].
Inhaled Prostanoids: Iloprost (Ventavis™), Treprostinil (Tyvaso™)
The next iteration of available prostacyclin therapy moved from the pump and catheter based therapies that require continuous administration to an inhaled delivery system with frequent dosing. As with prostanoids in general, the inhaled prostacyclins induce pulmonary vascular dilation and inhibit platelet aggregation and cell proliferation [70, 71]. At their inception, inhaled prostanoids possessed some attractive theoretical characteristics that included the direct inhalation of drug directly into the alveoli and subsequently into the pulmonary capillaries. Additionally, the inhaled route avoids the need for central venous access or painful subcutaneous injections. Unfortunately, there are a number of disadvantages with inhaled prostanoids including being less potent (effective) than parenteral therapy and the requirement for multiple inhalations per day.
Pharmacologic Features and Clinical Considerations
Iloprost (Ventavis™) was the first inhaled prostacyclin to gain approval in 2004 following a 12 week, randomized, double blind, placebo-controlled trial of 203 patients with predominantly PAH but also chronic thromboembolic pulmonary hypertension (CTEPH). Patients were randomized to either 2.5 μg or 5.0 μg of iloprost versus placebo with the combined primary endpoint of improvement in at least one functional class and a 10 % improvement in 6MWD without clinical deterioration or death. By study’s end, those randomized to iloprost achieved a significant improvement in the primary endpoint (17 % with iloprost versus 5 % with placebo). Improvements in secondary endpoints of dyspnea score, quality of life, and hemodynamics also favored iloprost (though it should be noted that hemodynamics were measured shortly after drug administration) [72].
A different inhaled prostanoid, inhaled treprostinil, was evaluated in two small studies of PAH and chronic thromboembolic pulmonary hypertension (CTEPH) and demonstrated encouraging clinical and hemodynamic improvements [73, 74]. These findings led the way for the Treprostinil Sodium Inhalation Used in the Management of Pulmonary Arterial Hypertension 1 (TRIUMPH-1) clinical trial of 235 patients with FC III PAH on background therapy with either bosentan or sildenafil randomized to inhaled treprostinil or placebo over a 12 week period. By study’s end, a significant median improvement in peak 6MWD was seen in the inhaled treprostinil cohort although no significant improvements in secondary endpoints of time to clinical worsening or functional class were observed [75]. In a subsequent observational extension study, 118 patients who remained on inhaled treprostinil demonstrated a persistent improvement in 6MWD at 2 years. Inhaled treprostinil received FDA approval in 2009 [76].
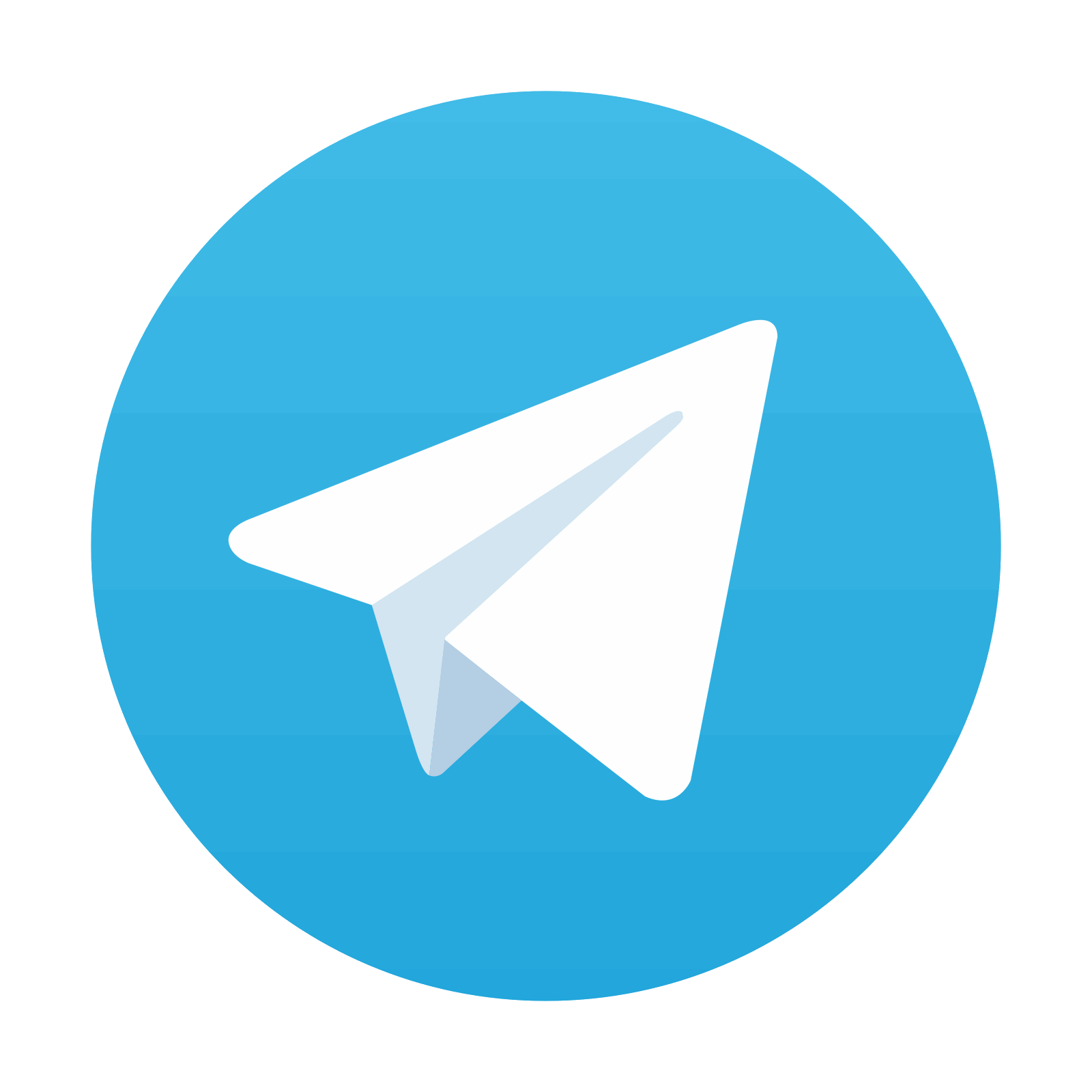
Stay updated, free articles. Join our Telegram channel

Full access? Get Clinical Tree
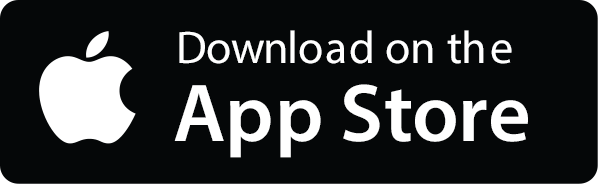
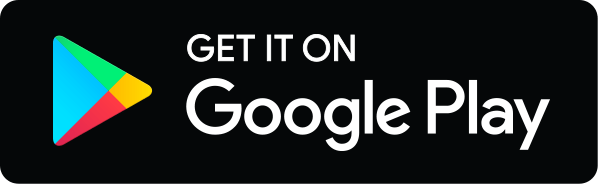
