Fig. 1
Timeline for development of hypertension after solid organ transplant. The etiology of hypertension can differ depending on its timing post-transplantation. Recognizing the potential causes for hypertension in the post-transplant period is important for optimal blood pressure management
Bladder distension is a less commonly recognized source of hypertension in a post-surgical patient. In a physiologic study evaluating the response to bladder distension in sixteen healthy volunteers, it was noted that an increase in the measured sympathetic outflow was noted when the urge to urinate was most pronounced. With this, there was also a concomitant increase in blood pressure from 125 ± 2/74 ± 2 mmHg to 140 ± 4/84 ± 3 mmHg (Fagius and Karhuvaara 1989). This effect of elevated blood pressure with bladder distension is especially a consideration in non-kidney transplant recipients because they are less likely to have their bladder routinely drained immediately post-surgery. Even if voiding was not a problem pre-transplant, this should be a consideration because several medications, particularly opioids, impair bladder emptying.
Liver transplant recipients have distinctive reasons to develop hypertension post-transplant. Prior to transplant, these patients are vasodilated and have decreased systemic vascular resistance and increased cardiac output. After liver transplantation, this pathophysiology reverses leading to increased systemic vascular resistance and later reduced cardiac output. This process is augmented by CNI mediated vasoconstriction, resulting in increasing blood pressure within weeks to months following liver transplantation (Hryniewiecka and Żegarska 2011). This phenomenon can be striking. Mean blood pressure can rise by up to 40–50 mmHg following liver transplantation (Textor 1993).
2.2 Chronic Hypertension Post-transplant
2.2.1 Contributing Factors Across All Solid Organ Transplant Recipients
Chronic hypertension is common in general population so it is not surprising that hypertension is widespread among transplant recipients who often have multiple medical comorbidities and are on medications known to aggravate hypertension (i.e. CNIs and steroids). Nevertheless, recognizing potentially modifiable factors that could contribute to hypertension is essential for optimal patient management and to reduce medication burden and side effects (Fig. 2). Obesity, non-steroidal anti-inflammatory drug (NSAID) use, alcohol consumption, excess sodium, sleep apnea, renal artery stenosis (especially a consideration for kidney transplant recipients), primary hyperaldosteronism, pheochromocytoma, and hypothyroidism are some of the most common modifiable factors that can lead to hypertension (Dalby et al. 2001). These potentially modifiable factors can easily be overlooked in transplant recipients who have complicated medical histories.


Fig. 2
Causative Factors Leading to Hypertension in Kidney Transplant Recipients
2.2.2 The Effect of Calcineurin Inhibitors (CNIs) on Blood Pressure
It is difficult to quantify the true effect of CNIs on the prevalence of hypertension, since the definition of high blood pressure has changed over time and the use of CNI-based maintenance immunosuppressive regimens is ubiquitous. What is striking, however, is the high prevalence of hypertension, across different organ transplants in immunosuppression regimens that primarily include CNIs. In one study, kidney transplant who were on belatacept based (non-CNI) immunosuppression needed fewer antihypertensive medications (median 2 drugs) compared with those on CNI based regimens (median 4 drugs) 10 years after follow up (Grannas et al. 2014). In liver transplant patients who typically are not hypertensive pre-transplant, the prevalence of hypertension has been noted to rise to as high as 65–85 % of recipients post-transplant. Similar increases have been quoted for cardiac transplant patients (increase from 10 % pre-transplant to 71–100 % post-transplant), kidney transplant recipients (45–55 % pre-transplant, increasing to 67–86 post-transplant), and even bone marrow transplant recipients (5–10 % pre-transplant, increasing to 33–60 % post-transplant) (Textor et al. 1994). CNIs may have several physiologic effects on blood pressure. They can impair vascular tone by causing vasoconstriction and/or impairing vasodilatation. Cyclosporine (CSA) in particular is known to cause acute vasoconstriction of the renal vessels, mediated by increased levels of endothelin in the pre-glomerular vessels (Meyer-Lehnert et al. 1997; Haug et al. 1995). Thromboxane has also been implicated in promoting vasoconstriction, though experimental data has shown mixed results (Nasser et al. 2014). Other studies suggest that CNIs are associated with impaired vasodilation, which occurs because CNIs can activate protein kinase C leading to reduced nitric oxide production (Vaziri et al. 1998) by the vascular endothelium (Oriji and Keiser 1999). Calcium channel blockers may have a role to play in ameliorating some of these deleterious effects (Sanchez-Lozada et al. 2000; Chander and Chopra 2005).
Some data suggests that CNIs alter the renin/angiotensin system, but this is controversial. Some studies suggest that CNIs suppress renin secretion (Bantle et al. 1987), while others suggest that CNIs directly increases renin secretion (Kurtz et al. 1988; Saraswat et al. 2014). Similar heterogeneity is noted with regards to angiotensin. In one study, CNIs are associated with the up-regulation of angiotensin II receptors and calcium responses in human vascular smooth muscle cells (Avdonin et al. 1999) whereas other studies have noted a downregulation of angiotensin receptors in the kidney (Nishiyama et al. 2003). In addition to the aforementioned mechanisms, CNIs have been shown to promote a sodium-avid state by increasing sodium reabsorption in the proximal tubule (Epting et al. 2006) and the loop of Henle (Ciresi et al. 1992; Esteva-Font et al. 2007).
Chronic administration of CNIs has been associated with obliterative arteriolopathy, ischemic glomeruli, and striped fibrosis of the renal interstitium (Naesens et al. 2009). Given the kidneys central role in blood pressure control, it is logical that renal dysfunction resulting from CNIs could also aggravate hypertension.
In systemic vasculature, CNI use is associated with increased arterial stiffness (Martínez-Castelao et al. 2005), which would not only lead to hypertension, but cardiovascular risk in general. Sirolimus (Joannidès et al. 2011) and belatacept (Melilli et al. 2015) are both associated with reduced vascular stiffness and hypertension as compared to CNIs. The similar changes in blood pressure have been noted with other CNIs, including tacrolimus, although the hypertensive effect of cyclosporine is higher than that seen with tacrolimus (Radermacher et al. 1998).
2.2.3 The Effect of Corticosteroids on Blood Pressure in Transplant Recipients
Corticosteroids may mediate hypertension by stimulating mineralocorticoid receptors and promoting sodium reabsorption. The impact of corticosteroids on hypertension is most pronounced in the early post-transplant period when solid organ transplant recipients often receive high doses of corticosteroids as part of their induction therapy. However, kidney transplant recipients maintained on corticosteroids long term tend have higher blood pressure than those in steroid avoidance or withdrawal programs (Knight and Morris 2010). In a meta-analysis of 34 studies including 5637 kidney transplant recipients, there was a reduced incidence of hypertension in the steroid avoidance or withdrawal group, compared with those on steroids (RR 0.90, CI 0.85–0.94, P < 0.0001) (Knight and Morris 2010).
2.2.4 Unique Causes of Hypertension in Kidney Transplant Recipients
Because the kidney has a role in blood pressure control, any factor (donor or recipient related) that is associated with a lower GFR, is indirectly associated with hypertension post-transplant. Examples of donor related factors associated with hypertension post-transplant include: increased donor age (Ducloux et al. 2002), and presence of donor hypertension (Guidi et al. 1996).
Studies have shown that recipients of kidneys from hypertensive donors are prescribed more anti-hypertensive medications than recipients of kidneys from normotensive donors (Guidi et al. 1996). In the study by Guidi et al., this effect was mainly restricted to recipients without a family history of hypertension (Guidi et al. 1996). Recipients of kidneys from expanded criteria donors [defined as older kidney donors (≥60 years) or donors who are aged 50–59 years and have two of the following three features: hypertension, terminal serum creatinine >1.5 mg/dl, or death from cerebrovascular accident] also have higher rates of hypertension at 3 years and 5 years post transplantation than recipients of kidneys from standard criteria donors (Blanca et al. 2012; Wlodarczyk et al. 2003). Recipients of kidneys from older donors also have lower graft function after transplantation (Noppakun et al. 2011) and therefore the donor related factors associated with hypertension may simply be related to reduced renal allograft function, but this remains unclear.
Certain donor genetic variants such as single-nucleotide polymorphisms within the genes that encode for ABCC2, ABCB1 and CYP3A5, and APOL-1 have been indirectly associated with higher rates of post-transplant hypertension likely resulting from their association with reduced allograft function. Specifically, polymorphisms in ABCC2 are associated with delayed graft function and certain CYP3A5 mutations (Joy et al. 2007), and ABCB1 polymorphisms (Hauser et al. 2005) are associated with CNI toxicity. The APOL-1 gene variant which has been associated with kidney disease in African Americans is also associated with early graft failure in recipients of kidneys from donors with this gene variant (Thomas et al. 2013; Weir et al. 2015; Reeves-Daniel et al. 2011).
Just as renal artery stenosis in a non-transplant patient is associated with hypertension; renal artery stenosis of the transplant renal artery (TRAS) is associated with hypertension. Although the prevalence of TRAS is 1–23 % of renal transplant recipients, it is thought to contribute to hypertension in between 1 and 5 % of kidney transplant recipients (Fervenza et al. 1998; Bruno et al. 2004). It may become apparent at any time post transplantation, though presentation between 3 months and 2 years is typical. The stenosis most often occurs near the anastomosis and is a result of the technical aspects of the transplant surgery itself (Willicombe et al. 2014) such as vessel torsion, use of vascular clamps, perfusion pump cannulation injury, disproportionate vessel size, or a reaction to suture material. Less commonly the stenosis is diffuse along the transplant renal artery, in which case immune based mechanisms have been proposed as a potential etiology (Wong et al. 1996). In fact, the presence of de novo class II donor-specific antibodies has been associated with development of post-anastomosis TRAS (Willicombe et al. 2014).
The typical presentation of transplant renal artery stenosis is worsening or refractory hypertension and/or graft function, with notable edema from a sodium-avid state. An audible bruit in the correct clinical context may be useful in the diagnosis, though it is neither sensitive nor specific. Doppler ultrasound often demonstrates an increase in the peak systolic velocity >2.5 m/s, and a “tardus parvus” waveform abnormality in the intra-renal vessels. The gold standard for diagnosis, however, remains renal angiography (and if possible, carbon dioxide angiography should be utilized, in order to avoid iodinated contrast). Treatment options include conservative therapy, angioplasty with or without stent placement, versus surgical correction. Treatment decisions are determined on a case by case basis are are reviewed elsewhere (Bruno et al. 2004; Buturović-Ponikvar 2003; Chen et al. 2015). The effects of treatment on blood pressure have been mixed (Touma et al. 2014; Ali et al. 2015).
Other potential kidney transplant specific factors that could lead to hypertension include increased cold ischemia time and/or delayed graft function and other causes of impairment in allograft function such as acute and chronic rejection, medication induced nephrotoxicity, BK nephropathy, recurrent disease in the allograft, and ureteral obstruction (Weir et al. 2015; Mangray and Vella 2011; Cosio et al. 1999). Whenever a kidney transplant recipient has an abrupt change in blood pressure, these conditions should be a consideration. Not uncommonly, a change in blood pressure is the first signal of impending allograft dysfunction from rejection or recurrent disease.
2.2.5 Unique Contributors to Hypertension in Cardiac Transplant Recipients
Cardiac transplant patients experience inadequate adaptation of the renin-angiotensin system in the setting of fluid retention, which could contribute to the incidence and severity of hypertension in this population (Braith et al. 1996). Pharmacologic suppression of the RAS system eliminated avid salt and fluid retention in heart transplant recipients, improving blood pressure and overall fluid homeostasis (Braith et al. 2003).
3 Hypertension Following Living Kidney Donation
A paucity of data exists about the effect that living kidney donation has on blood pressure. Many live donors have not been followed prospectively, and it is hard to distinguish the effect of donation on blood pressure because hypertension is prevalent in the general population. In addition, the lack of appropriate control groups makes it challenging to make inferences about the effect of living kidney donation on blood pressure. A meta-analysis that included 5145 kidney donors revealed that factors associated with higher blood pressure post-kidney donation included older age at the time of donation, age, male sex, increased BMI, and a lower pre-donation glomerular filtration rate (Boudville et al. 2006). Recently, a prospective controlled trial evaluating live kidney donor outcomes 3 years post-donation revealed no difference in blood pressure as measured by ambulatory blood pressure monitoring at 36 months (Kasiske et al. 2015). A retrospective, matched-cohort study with a median follow up of 10.9 years demonstrated a higher incidence (11 %) of gestational hypertension or preeclampsia in living kidney donors than in matched non-donors (5 %), but maternal and fetal outcomes did not differ significantly between the two groups (Garg et al. 2015).
4 Hypertension Management for the Transplant Patient
4.1 Blood Pressure Measurement and Diagnosis
As in the general population, before acting on a blood pressure measurement; the provider must consider the circumstances and make sure that the measurement is reliable. Appropriate blood pressure measurement techniques are imperative to avoid falsely low or high readings as over or under-treatment of hypertension can lead to adverse outcomes. Furthermore, white coat hypertension and masked hypertension needs to be identified to avoid the unique risks in these situations (Griffin and Schinstock 2015).
Home and ambulatory blood pressure monitoring are useful adjuncts to in-office blood pressure readings. Several studies have suggested that office blood pressure readings are unreliable in both the general and the renal transplant population (Kooman et al. 2001; Stenehjem et al. 2006). In fact, home blood pressure readings correlate better with ambulatory blood pressure readings than office readings (Agena et al. 2011; Sberro-Soussan et al. 2012). When office blood pressures are compared with ambulatory blood pressure readings one can further identify patients with “white coat hypertension”, in whom office blood pressures are typically higher than the 24 h readings, and patients with “masked hypertension”, in which the opposite occurs: blood pressures in the office appear normal, whereas those at home are high. Additionally, ambulatory blood pressure readings are able to identify the nocturnal blood pressure patterns, stratifying patients into those with normal nocturnal dipping, versus those with a “non-dipping” pattern or even “reverse dipping”. We recommend monitoring ambulatory blood pressures at the 1 year annual visit post transplantation, whenever feasible. In a study evaluating 24 h ambulatory blood pressures at 1 year post kidney transplantation, it was noted that the lack of nocturnal fall in SBP is related to poor allograft function, high chronic vascular score on biopsy, and high resistive index irrespective of allograft fibrosis (Wadei et al. 2007).
4.2 Goals of Therapy
Currently, there are no specific high quality evidence based recommendations for blood pressure targets in transplant recipients. As in the general population, there remains some debate regarding optimal blood pressure targets. According to the Eighth Report of the Joint National Committee on the Evidence-Based Guideline for Management of High Blood Pressure in Adults (James et al. 2014), blood pressure of greater than 140/90 is still felt to be a reasonable definition of high blood pressure, although the recently published SPRINT trial (SPRINT Research Group et al. 2015) suggests that certain patients (including some high risk patients with CKD) may do better with even lower blood pressures. In kidney transplant recipients, the Kidney Disease Outcomes Quality Initiative (KDOQI) commentary on the 2012 KDIGO guidelines stipulate that a target blood pressure of <130/80 in kidney transplant recipients is not guided by evidence, and in the absence of data to support the benefits of a BP goal <140/90 mmHg in transplant recipients, it is better to individualize BP goal decisions, taking into account risk and benefit profiles (Taler et al. 2013).
Excluding hypertensive emergencies when treatment is indicated for acute management of hypertension, the main goal of treatment is to reduce target organ damage and cardiovascular risk over the long term. Compared with End Stage Renal Disease (ESRD), kidney transplant recipients have an improved cardiovascular risk profile (Wolfe et al. 1999). However, the transplant in and of itself does not eliminate this risk (Ojo 2006), and death from cardiovascular disease remains the most common reason for decreased patient survival (Ojo et al. 2000). In addition to this, it is important to take into consideration that reduced allograft function in and of itself may confer increased cardiovascular risk. In a large retrospective analysis of 58,900 adult renal transplant recipients registered in the United States Renal Data System, it was noted that serum creatinine values at 1 year after transplantation were strongly associated with the risk for cardiovascular death (Meier-Kriesche et al. 2003). While the following text describes pharmacologic options in the management of hypertension after transplantation, it should be noted that other aspects of cardiovascular risk such as glycemic control and lipid status should also be addressed (Cosio et al. 2005) in tandem with lifestyle modification.
4.3 Pharmacological Therapy
An optimal antihypertensive treatment regimen for a transplant patient does not exist and initial anti-hypertensive therapy should be tailored based on the patient’s underlying comorbidities and preferences. We recommend using medications the patient used prior to transplantation unless there are particular drug-drug interactions or adverse effects. Special considerations for transplant patients are described below.
4.3.1 Calcium Channel Blockers
Calcium channel blockers are classified into non-dihydropyridine calcium channel blockers (examples include verapamil and diltiazem) and dihydropyridine calcium channel blockers (examples include amlodipine, nifedipine, and nicardipine). The dihydropyridine calcium channel blockers have a higher selectivity for vascular smooth muscle compared with myocardial tissue. In the transplant population on CNIs, non-dihydropyridine calcium channel blockers need to be used with caution because they can lead to increased CNI levels due to the inhibition of CYP3A4 activity (Hooper et al. 2012; Hooper et al. 2011). Dihydropyridine calcium channel blockers such as nifedipine have been shown to have an antagonistic effect to CSA mediated vasoconstriction at the level of the endothelium, favoring their use in this population (Sanchez-Lozada et al. 2000; Chander and Chopra 2005; Ruggenenti et al. 1993; Cross et al. 2009; Mehrens et al. 2000; Kuypers et al. 2004).
In the general population, there is some concern for increased risk of myocardial infarction with high doses of short acting dihydropyridine calcium channel blockers such as nifedipine (Psaty et al. 1995), and longer acting agents such as amlodipine appear to be safer. In the renal transplant population, one study has shown that dihydropyridine calcium channel antagonists were associated with an increased risk for ischemic heart disease in this population (relative risk, 2.26; 95 % confidence interval, 1.24–4.12; P = 0.008), and this association was independent of other antihypertensive agents and risk factors (Kasiske et al. 2000). On the other hand, a prospective randomized (but non-blinded or placebo controlled) study in the cardiac transplant population suggested that diltiazem (selected in the study protocol due to its antihypertensive effects) may attenuate the usual reduction in the coronary artery diameter during the first year after cardiac transplantation and may inhibit the development of visually evident coronary artery disease (Schroeder et al. 1993).
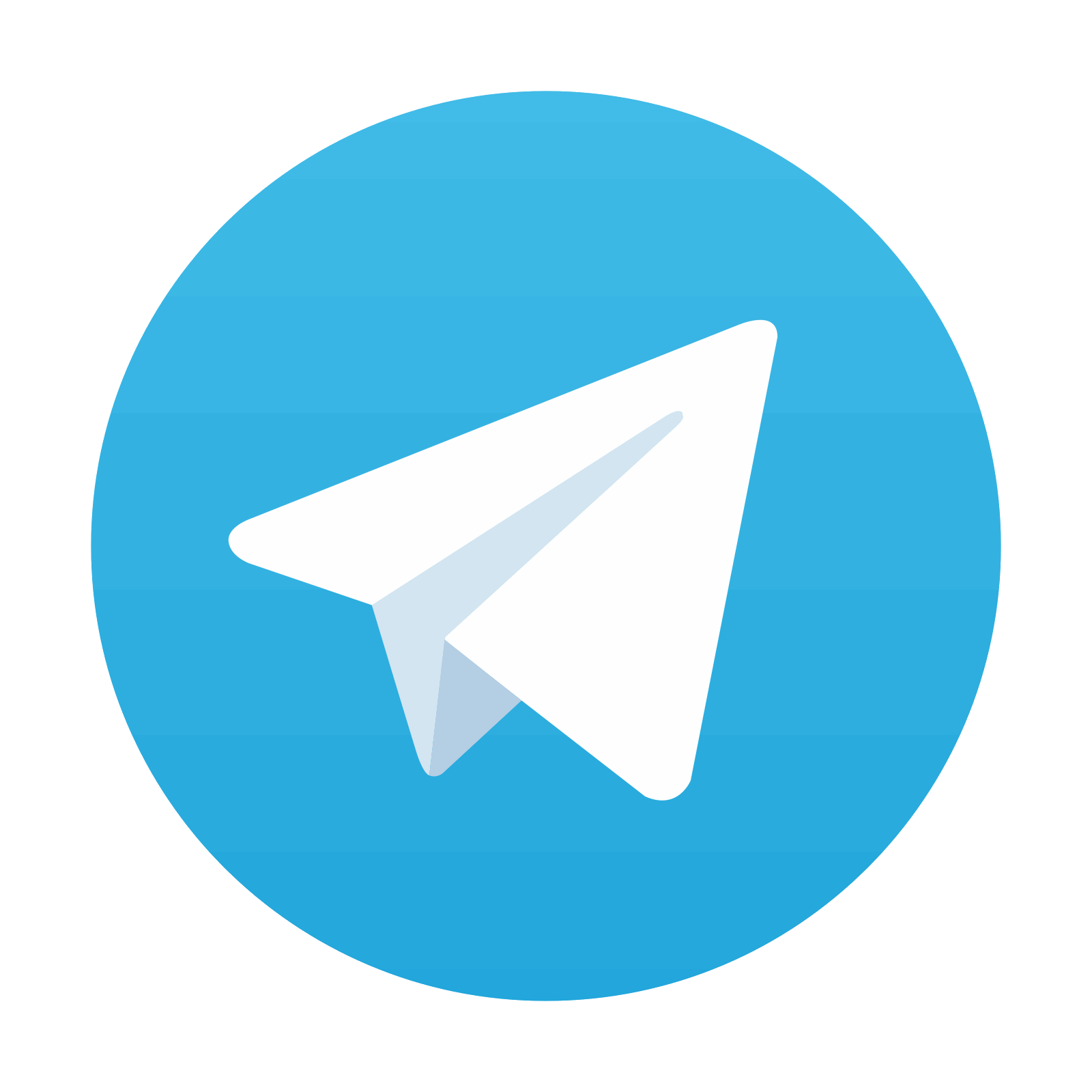
Stay updated, free articles. Join our Telegram channel

Full access? Get Clinical Tree
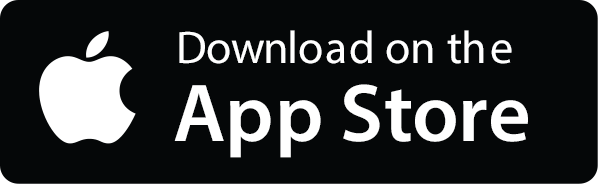
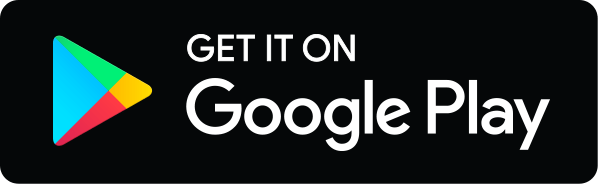