Cause
The heart failure syndrome may arise from diverse causes. Common causes of CHF are volume or pressure overload (or both) caused by congenital or acquired heart disease and myocardial diseases. Tachyarrhythmias and heart block can also cause heart failure at any age. By far the most common causes of CHF in infancy result from congenital heart defects (CHDs). Beyond infancy, myocardial dysfunctions of various etiologies are important causes of CHF. Among the rare causes of CHF are metabolic and endocrine disorders, anemia, pulmonary diseases, collagen vascular diseases, systemic or pulmonary hypertension, neuromuscular disorders, and drugs such as anthracyclines.
Congenital Heart Disease
Volume overload lesions, such as ventricular septal defect (VSD), patent ductus arteriosus (PDA), and endocardial cushion defect (ECD), are the most common causes of CHF in the first 6 months of life. In infancy, the time of the onset of CHF varies predictably with the type of defect. Table 27-1 lists common CHDs according to the age at which CHF develops. When looking at the table, the following should also be noted.
- 1.
Children with tetralogy of Fallot (TOF) do not develop CHF unless they have received a large systemic-to-pulmonary artery shunt procedure (e.g., too large a Gore-Tex interposition shunt).
- 2.
Atrial septal defect (ASD) rarely causes CHF in the pediatric age group, although it causes CHF in adulthood.
- 3.
Large left-to-right shunt lesions, such as VSD and PDA, do not cause CHF before 6 to 8 weeks of age because the pulmonary vascular resistance does not fall low enough to cause a large left-to-right shunt until this age. The onset of CHF resulting from these left-to-right shunt lesions may be earlier in premature infants (within the first month) because of an earlier fall in the pulmonary vascular resistance in these infants.
Age of Onset | Cause |
---|---|
At birth | HLHS |
Volume overload lesions: | |
Severe tricuspid or pulmonary insufficiency | |
Large systemic arteriovenous fistula | |
First week | TGA |
PDA in small premature infants | |
HLHS (with more favorable anatomy) | |
TAPVR, particularly those with pulmonary venous obstruction | |
Others | |
Systemic arteriovenous fistula | |
Critical AS or PS | |
1–4 wk | COA with associated anomalies |
Critical AS | |
Large left-to-right shunt lesions (VSD, PDA) in premature infants | |
All other lesions previously listed | |
4–6 wk | Some left-to-right shunt lesions such as ECD |
6 wk–4 mo | Large VSD |
Large PDA | |
Others such as anomalous left coronary artery from the PA |
Acquired Heart Disease
Acquired heart disease of various causes can lead to CHF. With acquired heart disease, the age at onset of CHF is not as predictable as with CHD, but the following generalities apply:
- 1.
Dilated cardiomyopathy is probably the most common cause of CHF beyond infancy. It may cause CHF at any age during childhood and adolescence. The cause of the majority of dilated cardiomyopathy is idiopathic, but it may be caused by infectious, endocrine, or metabolic disorders; autoimmune diseases; or after antineoplastic treatment (e.g., anthracycline).
- 2.
Doxorubicin cardiomyopathy may manifest months to years after the completion of chemotherapy for malignancies in children.
- 3.
Cardiomyopathies associated with muscular dystrophy and Friedreich’s ataxia may cause CHF in older children and adolescents.
- 4.
Myocarditis associated with Kawasaki’s disease is seen in children 1 to 4 years of age.
- 5.
Patients who received surgery for some types of CHDs (e.g., Fontan operation, surgery for TOF, transposition of the great arteries and other cyanotic defects) may remain in or develop CHF after varying period of time.
- 6.
Viral myocarditis tends to be more common in small children older than 1 year. It occurs occasionally in the newborn period, with a fulminating clinical course with poor prognosis.
- 7.
Acute rheumatic carditis is an occasional cause of CHF that occurs primarily in school-age children.
- 8.
Rheumatic valvular heart diseases, usually volume overload lesions such as mitral regurgitation (MR) or aortic regurgitation (AR), cause CHF in older children and adults. These diseases are uncommon in industrialized counties.
- 9.
Endocardial fibroelastosis, a rare primary myocardial disease, causes CHF in infancy; 90% of cases occur in the first 8 months of life.
Miscellaneous Causes
Miscellaneous causes of CHF includes the following:
- 1.
Metabolic abnormalities (severe hypoxia and acidosis, as well as hypoglycemia and hypocalcemia) can cause CHF in newborns.
- 2.
Endocrinopathy such as hyperthyroidism.
- 3.
Supraventricular tachycardia (SVT) causes CHF in early infancy.
- 4.
Complete heart block associated with structural heart defects causes CHF in the newborn period or early infancy.
- 5.
Severe anemia may be a cause of CHF at any age. Hydrops fetalis may be a cause of CHF in the newborn period and severe sicklemia at a later age.
- 6.
Bronchopulmonary dysplasia seen in premature infants causes predominantly right-sided heart failure in the first few months of life.
- 7.
Primary carnitine deficiency (plasma membrane carnitine transport defect) causes progressive cardiomyopathy with or without skeletal muscle weakness that begins at 2 to 4 years of age.
- 8.
Acute coronary pulmonale caused by acute airway obstruction (such as seen with large tonsils) can cause CHF at any age but most commonly during early childhood.
- 9.
Acute systemic hypertension, as seen in acute postinfectious glomerulonephritis, causes CHF in school-age children. Fluid retention with poor renal function is important as a cause of hypertension in this condition.
Pathophysiology
Cardiac output is determined by preload, afterload, myocardial contractility, and heart rate. Cardiac output is proportional to filling pressure (preload) and inversely proportional to the resistance against which the heart pumps (afterload).
Preload
According to the Frank-Starling law, as the ventricular end-diastolic volume (or preload) increases, the healthy heart increases cardiac output until a maximum is reached and cardiac output can no longer be augmented (see Figure 27-1 , which appears in the section on Management in this chapter). This is a built-in property of the heart that normally allows it to pump out automatically whatever amount of blood flows into the heart. When the left ventricular (LV) end-diastolic pressure reaches a certain point, however, pulmonary congestion develops with congestive symptoms (tachypnea and dyspnea). Congestive symptoms occur even with a normally functioning myocardium if the end-diastolic pressure is greatly increased, such as seen with infusion of a large amount of fluid or blood. An increase in the stroke volume is also achieved in the failing heart when the preload is increased but the failing heart does not achieve the same level of maximal cardiac output as seen in the normal heart. The increased stroke volume obtained in this manner increases the myocardial oxygen consumption.

Afterload
Afterload is the force that resists myofibril shortening during systole, which contributes to total myocardial wall stress (or tension). A decrease in afterload increases cardiac output, and an acute increase in afterload results in decreases in stroke volume and ejection fraction. Indices of afterload include aortic pressure, total systemic vascular resistance, arterial impedance, and myocardial peak wall stress. Afterload reducing increases cardiac output without increasing oxygen consumption.
Wall Stress
According to the law of Laplace, wall tension is the product of pressure and radium.
Wall stress = Pressure × Radius / 2 × Wall thickness
The Laplace law, although an oversimplification, emphasizes the following two points: (1) the bigger the LV and the greater the radius, the greater the wall stress, and (2) at any given radius (LV size), the greater the pressure developed in the LV, the greater the wall stress. Thus, dilated ventricles require more tension in the wall and thus increased oxygen demand to generate the same pressure. A relationship exists between wall stress and preload as well as afterload. Preload can be defined as the wall stress at the end of diastole. The afterload, being the load on the contracting myocardium, is the wall stress during LV ejection.
The increased wall tension in the dilated ventricle leads to ventricular hypertrophy that tends to keep the wall tension low. Well-trained athletes develop cardiac hypertrophy, which helps reduce wall stress, according to Laplace’s law. A failing heart will also hypertrophy to reduce the increase in wall stress, but the hypertrophy in the failing heart is abnormal because it occurs as part of ventricular remodeling secondary to neurohormonal compensatory mechanisms. (Cardiac remodeling is defined as genomic expression resulting in molecular, cellular, and interstitial changes that are manifested clinically as changes in size, shape, and function of the heart after cardiac injury.) Although hypertrophy may tend to lower wall tension, abnormally hypertrophied ventricles may interfere with synthesis of some of the contractile proteins and leads to collagen damage, including fibrosis. It is also possible that capillary growth does not keep up with the growth of the muscle fibers, causing difficulties in supplying energy.
Compensatory Mechanisms
In the early stages of heart failure, various compensatory mechanisms are evoked to maintain normal metabolic function. Among the compensatory responses are the activation of the sympathetic nervous system and the renin–angiotensin–aldosterone system. Although these responses are an attempt to preserve cardiovascular homeostasis and thus beneficial initially, chronic stimulation of these systems may be deleterious in the natural history of myocardial dysfunction.
- 1.
One major compensatory mechanism for increasing cardiac output is an increase in sympathetic tone secondary to increased adrenal secretion of circulating epinephrine and increased neural release of norepinephrine. The initial beneficial effects of adrenergic stimulation include increased heart rate and myocardial contractility with a resulting increase in cardiac output. However, chronic adrenergic stimulation eventually leads to adverse myocardial effects, including increased afterload, hypermetabolism, arrhythmogenesis, and direct myocardial toxicity.
- a.
Catecholamines are toxic to cardiac muscle, perhaps by producing calcium overload or by inhibiting the synthesis of contractile proteins.
- b.
High catecholamine levels decrease the density of β-adrenergic receptors on the surfaces of the myocardial cell, which may be the major cause of the functional loss of the catecholamine-mediated positive inotropic response.
- a.
In clinical settings, the reduction of adrenergic stimulation by the use of β-adrenergic blockers has resulted in clinical improvement in patients with dilated cardiomyopathy, in whom increased levels of catecholamines have been shown to be present.
- 2.
The reduced blood flow to the kidneys in patients with CHF causes a marked increase in renin output, and this in turn causes the formation of angiotensin II. Angiotensin II leads to a further increase in reabsorption of both water and salt from the renal tubules. Angiotensin II may cause a trophic response in vascular smooth muscle (with vasoconstriction) and myocardial hypertrophy. Angiotensin II also promotes myocardial fibrosis. Thus, although a hypertrophic response is adaptive by attempting to restore wall stress to normal, angiotensin II plays a maladaptive role in CHF by initiating fibrosis and altering ventricular compliance.
Thus, the reasons for using β-adrenergic blockers and angiotensin-converting enzyme (ACE) inhibitors in the treatment of CHF are to block the maladaptive role of adrenergic and renin–angiotensin–aldosterone systems.
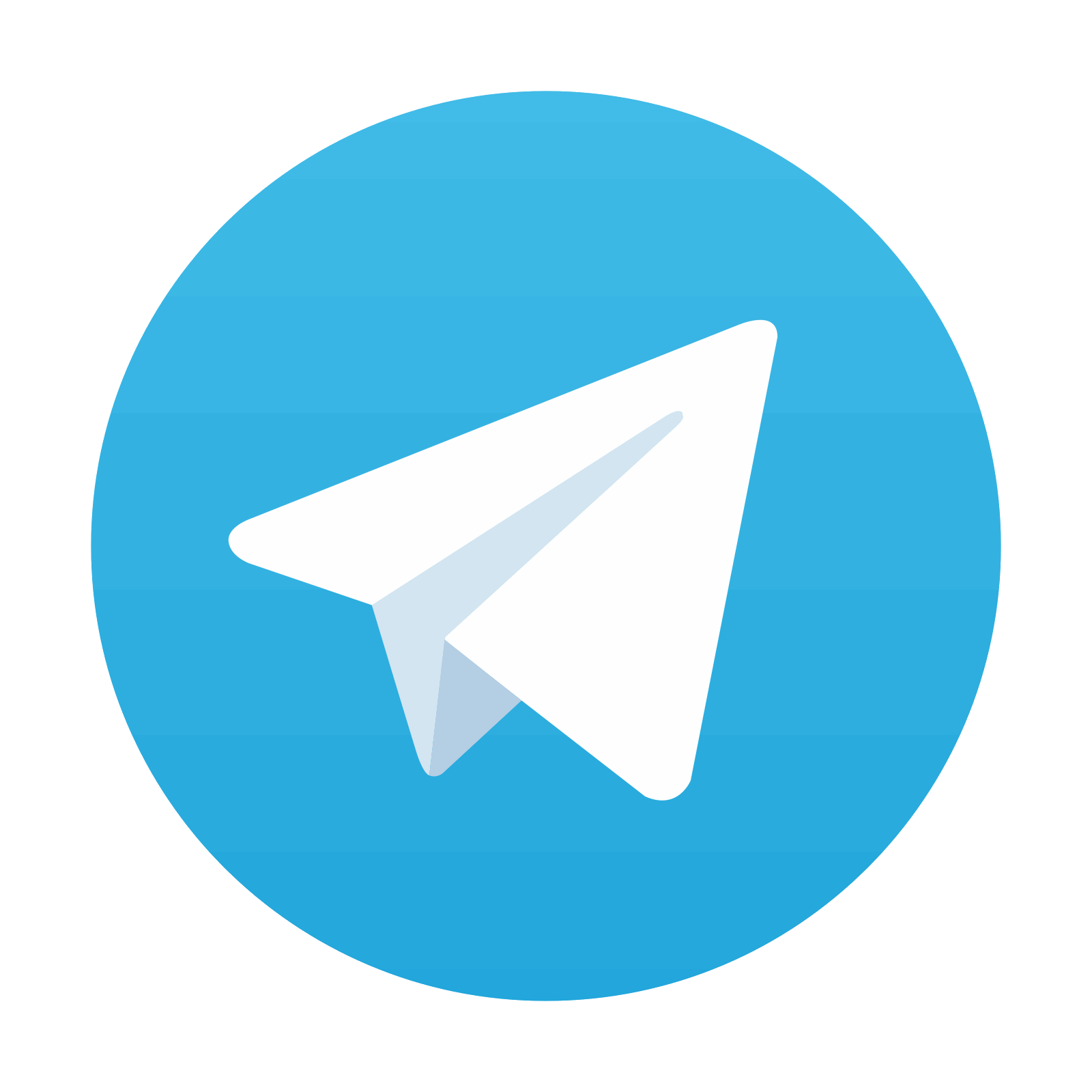
Stay updated, free articles. Join our Telegram channel

Full access? Get Clinical Tree
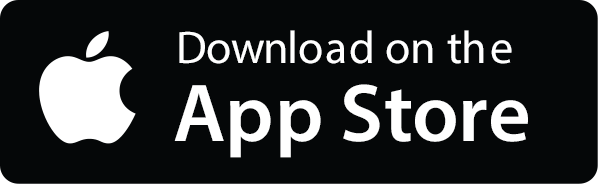
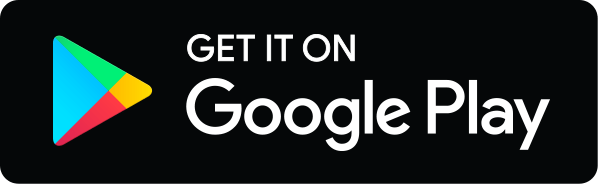