INTRODUCTION
As invasive and noninvasive methods of assessment of ventricular performance evolve, the clinical importance of diastolic function in both children and adults with congenital heart disease has become better appreciated. Alterations in ventricular geometry and loading conditions are the hallmark of congenital heart disease and further complicate the quantitative evaluation of diastolic function. Additional intrinsic and extrinsic factors also may affect diastolic performance, significantly altering this phase of the cardiac cycle. Systolic ventricular function, atrial and ventricular compliance, ventricular filling pressure, pericardial constraint, and ventricular interaction may each have a significant effect on overall diastolic performance. This chapter will discuss current concepts in diastolic function in children and adults with congenital heart disease and detail ongoing clinical efforts regarding the assessment of diastolic performance in this population.
PATHOPHYSIOLOGY OF DIASTOLIC DYSFUNCTION IN CHILDREN
Diastole is a complex process involving both active and passive components. Abnormalities of relaxation and early rapid filling are often manifested by changes in the rate of relaxation and the amount of early rapid filling. Diastolic dysfunction also is manifested by changes in chamber stiffness or compliance. Adverse changes within the myocardial cytoskeleton are common in diastolic disease processes, with increases in collagen content and altered extracellular matrix composition leading to altered chamber compliance.
The rate of pressure decline within the ventricular chamber can be determined by invasive methods. Tau (τ), or pressure half-time, is the period of time for pressure to fall by 50% of its initial measured value. Impaired relaxation leads to a decreased rate of ventricular pressure decline. Lengthening of isovolumic relaxation time (IVRT) (defined as the time interval between aortic valve closure and mitral valve opening) is also characteristic of abnormal diastolic filling and can be evaluated noninvasively by M-mode or pulsed-wave Doppler echocardiography. However, neither IVRT nor τ elucidates whether dysfunction occurs in active or passive relaxation, which act in concert to cause a fall in ventricular pressure and augment filling. As diastolic disease progresses, increased ventricular end diastolic pressure further increases τ and pressure halt time, while concomitant changes in atrial compliance and filling pressure act to shorten IVRT duration.
Echocardiography, and in particular Doppler echocardiography, historically has been an essential noninvasive tool in the quantitative assessment of left ventricular (LV) diastolic function. Abnormalities of ventricular compliance and relaxation can be demonstrated by characteristic changes in mitral inflow and pulmonary venous Doppler patterns. The addition of newer methodologies including tissue Doppler echocardiography and flow propagation velocities enhanced the ability of echocardiographers to define and quantitate these adverse changes in diastolic performance. Because diastolic dysfunction often precedes systolic dysfunction, careful assessment of diastolic function is essential in the noninvasive characterization and evaluation of patients with congenital heart disease.
Normal Diastolic Function in Children
Normal maturation of the neonatal myocardium occurs over the first year of life, resulting in improved ventricular compliance with aging. Noninvasive evaluation of normal diastolic function in infants and children is influenced by several factors, including age, heart rate, and the respiratory cycle. Researchers have established reference values detailing both mitral and pulmonary venous Doppler velocities in a large cohort of children with unimpaired hearts ( Tables 25-1 , 25-2 , and 25-3 ). Similar to many echocardiographic parameters, these Doppler velocities also are significantly affected by loading conditions, making determination of diastolic dysfunction using these parameters alone very challenging in patients with congenital heart disease.
3-8 yrs (N = 75) | 9-12 yrs (N = 72) | 13-17 yrs (N = 76) | ||||
---|---|---|---|---|---|---|
FAC TOR | Mean | 1 SD | Mean | 1 SD | Mean | 1 SD |
E velocity (cm/sec) | 92 | 14 | 86 | 15 | 88 | 14 |
E TVI (cm) | 12.0 | 2.6 | 12.3 | 2.9 | 14.0 | 2.9 |
A velocity (cm/sec) | 42 | 11 | 41 | 9 | 39 | 8 |
A TVI (cm) | 3.7 | 1.1 | 3.7 | 1.0 | 3.7 | 1.1 |
A duration (msec) | 136 | 22 | 142 | 21 | 141 | 22 |
E at A velocity (cm/sec) | 16 | 7 | 14 | 5 | 12 | 4 |
E to A velocity ratio | 2.4 | 0.7 | 2.2 | 0.6 | 2.3 | 0.6 |
E to A TVI ratio | 3.7 | 2.0 | 3.7 | 1.5 | 4.2 | 1.7 |
Deceleration time (msec) | 145 | 18 | 157 | 19 | 172 | 22 |
End mitral A to R wave interval (msec) | 34 | 16 | 29 | 15 | 27 | 19 |
LV IVRT (msec) | 62 | 10 | 67 | 10 | 74 | 13 |
3-8 yrs (N = 75) | 9-12 yrs (N = 72) | 13-17 yrs (N = 76) | ||||
---|---|---|---|---|---|---|
FAC TOR | Mean | 1 SD | Mean | 1 SD | Mean | 1 SD |
Systolic velocity (cm/sec) | 46 | 9 | 45 | 9 | 41 | 10 |
Systolic TVI (cm) | 11.1 | 2.3 | 11.5 | 2.2 | 10.8 | 2.8 |
Diastolic velocity (cm/sec) | 59 | 8 | 54 | 9 | 59 | 11 |
Diastolic TVI (cm) | 8.8 | 1.8 | 9.2 | 2.5 | 12.1 | 3.1 |
Ratio of systolic to diastolic velocity | 0.8 | 0.2 | 0.8 | 0.2 | 0.7 | 0.2 |
Ratio of systolic to diastolic TVI | 1.3 | 0.3 | 1.3 | 0.4 | 0.9 | 0.3 |
Atrial reversal velocity (cm/sec) | 21 | 4 | 21 | 5 | 21 | 7 |
Atrial reversal duration (msec) | 130 | 20 | 125 | 20 | 140 | 28 |
Atrial reversal TVI (cm) | 1.7 | 0.5 | 1.6 | 0.6 | 2.0 | 0.9 |
UNIVARIATE ASSOCIATIONS | BIVARIATE PARTIAL ASSOCIATIONS * | |||
---|---|---|---|---|
VARIABLE | Age | RR | Age | RR |
Mitral E velocity | — | ↑ | ↓ | ↑ |
Mitral A velocity | ↓ | ↓↓ | ↑ | ↓↓ |
End A to R interval | ↑ | — | ↑ | — |
Duration of A wave | — | ↑ | — | — |
Mitral deceleration time | ↑↑↑ | ↑↑↑ | ↑ | ↑ |
Mitral E wave TVI | ↑↑ | ↑↑↑ | — | ↑↑↑ |
Mitral A wave TVI | — | ↓ | ↑ | ↓ |
Mitral E at A velocity | ↓ | ↓↓ | — | ↓↓ |
Mitral E to A ratio (velocity) | — | ↑↑↑ | ↓ | ↑↑↑ |
Mitral E to A ratio (TVI) | ↑ | ↑↑↑ | ↓ | ↑↑↑ |
LV IVRT | ↑↑ | ↑↑ | ↑ | ↑ |
PV systolic peak velocity | ↓ | — | ↓ | — |
PV diastolic peak velocity | — | ↑ | — | ↑ |
Peak PVAR velocity | — | — | — | — |
PVAR duration | ↑ | ↑↑ | — | ↑↑ |
PV systolic TVI | — | ↑ | — | ↑ |
PV diastolic TVI | ↑↑↑ | ↑↑↑ | ↑ | ↑ |
PVAR TVI | ↑ | ↑ | — | ↑ |
PV systolic to diastolic ratio (velocity) | ↓ | — | — | — |
PV systolic to diastolic ratio (TVI) | ↓↓ | ↓ | ↓ | ↓ |
Ratio of PVAR to mitral A wave duration | — | — | — | — |
Ratio of PVAR to mitral A wave TVI | ↓ | ↓↓ | — | ↓↓ |
* Univariate associations column demonstrates the association between age or heart rate (RR interval) and each dependent variable without accounting for other influences. Bivariate partial associations column demonstrates the effect of age or heart rate on each dependent variable after controlling for the influence of the other independent variable (age or heart rate). Upward arrows indicate positive associations between age or RR interval and the measured variable; downward arrows indicate negative associations. The number of arrows shown increases as the degree of association increases.
Mitral Inflow Doppler
Mitral inflow Doppler represents the diastolic pressure gradient between the left atrium and the left ventricle ( Fig. 25-1 ) (see Chapter 10 ). The early diastolic filling wave, or E wave, is the dominant diastolic wave in children and young adults and represents the peak left atrial (LA)-to-LV pressure gradient at the onset of diastole. The deceleration time (DT) of the mitral E wave reflects the period of time needed for equalization of LA and LV pressures. The late diastolic filling wave, or A wave, represents the peak pressure gradient between the left atrium and the left ventricle in late diastole at the onset of atrial contraction. Normal mitral inflow Doppler is characterized by a dominant E wave, a smaller A wave, and a ratio of E and A waves (E/A ratio) between 1 and 3. Normal durations of mitral DT and IVRT vary with age and have been reported in both pediatric and adult populations. , Mitral inflow Doppler velocities are affected not only by changes in LV diastolic function but also by a variety of additional hemodynamic factors, including age, altered loading conditions, heart rate, and changes in atrial and ventricular compliance. , Interpretation of characteristic patterns of mitral inflow must be carefully evaluated with particular attention paid to the potential impact of each of these hemodynamic factors on mitral inflow Doppler velocities.

As in the adult population, the earliest stage of LV diastolic dysfunction demonstrated by mitral inflow Doppler in children is abnormal relaxation. This Doppler pattern is characteristic of normal aging in adults and represents a mild decrease in the rate of LV relaxation with continued normal LA pressure. It is characterized by a reduced E-wave velocity, increased A-wave velocity, decreased E/A ratio less than 1, and a prolonged mitral DT and IVRT.
As diastolic dysfunction progresses, further changes in ventricular relaxation and compliance occur, leading to an increase in LA pressure. Increased LA pressure normalizes the initial transmitral gradient between the left atrium and the left ventricle, producing a “pseudonormalized” mitral inflow Doppler pattern with increased E-wave velocity and E/A ratio and normalized mitral DT and IVRT intervals. This pseudonormal Doppler pattern may be difficult to distinguish from normal mitral inflow Doppler; however, maneuvers that decrease ventricular preload, like the Valsalva maneuver, as well as additional evaluation of pulmonary venous inflow Doppler can help unmask this advanced degree of LV diastolic dysfunction.
Further deterioration of LV diastolic function results in restrictive ventricular filling with an additional increase in LA pressure and a concomitant decrease in ventricular compliance. The Doppler pattern of restrictive LV filling is characterized by additional increases in E-wave velocity, reduction in A-wave velocity, an increased E/A ratio greater than 3, and significant shortening of both mitral DT and IVRT.
Pulmonary Venous Doppler
Pulmonary venous Doppler combined with mitral inflow Doppler provides a more comprehensive assessment of LA and LV filling pressures (see Fig. 25-1 ). Pulmonary venous inflow consists of three distinct Doppler waves: a systolic wave (S wave), a diastolic wave (D wave), and a reversal wave with atrial contraction (Ar wave). In adolescents and adults with normal hearts, the characteristic pattern of pulmonary venous inflow consists of a dominant S wave, a smaller D wave, and a small Ar wave of low velocity and brief duration. In neonates and younger children, a dominant D wave is often present with a similar brief low-velocity, or even absent, Ar wave.
With worsening LV diastolic dysfunction, LA pressure rises, leading to diminished systolic forward flow into the left atrium from the pulmonary veins with relatively increased diastolic forward flow, resulting in a diastolic dominance of pulmonary venous inflow. More importantly, both the velocity and the duration of the pulmonary venous Ar wave are increased. Pediatric and adult studies have demonstrated that an Ar-wave duration more than 30 msec longer than the corresponding mitral A-wave duration or a ratio of pulmonary venous Ar-wave duration to mitral A-wave duration greater than 1.2 is predictive of elevated LV filling pressure ( Fig. 25-2 ).

Tissue Doppler Imaging
Tissue Doppler imaging is particularly well suited to the quantitative evaluation of LV diastolic function ( Fig. 25-3 ) (see Chapter 12 ). Both early (Ea) and late (Aa) annular diastolic velocities can be readily obtained by tissue Doppler echocardiography. Similar to systolic tissue Doppler velocities, differences in diastolic velocities exist (1) between the subendocardium and the subepicardium, (2) from cardiac base to apex, and (3) among various myocardial wall segments. Previous studies have reported an excellent correlation between early annular diastolic mitral velocity and simultaneous invasive measures of diastolic function at cardiac catheterization. Early annular diastolic velocities also appear to be less sensitive to changes in ventricular preload compared with corresponding early transmitral Doppler inflow velocities. Significant alterations in preload, however, have been shown to affect these diastolic tissue Doppler velocities. The effect of afterload on tissue Doppler velocities is less controversial, with many studies documenting significant changes in systolic and diastolic annular velocities with changes in ventricular afterload. Therefore, the clinical use of tissue Doppler velocities in patients with valvular stenosis or other etiologies of altered ventricular afterload need to be interpreted carefully in light of this limitation.

Tissue Doppler velocities are helpful in the discrimination between normal and pseudonormal transmitral Doppler filling patterns ( Fig. 25-4 ). In addition to changes incurred by loading conditions, alterations in LA pressure and LV end diastolic pressure also affect the early transmitral diastolic velocity. However, the corresponding tissue Doppler velocity is typically decreased in patients with pseudonormal filling, allowing differentiation of this abnormal filling pattern from one of normal transmitral Doppler inflow. Clinical reports have suggested a ratio of the early transmitral inflow Doppler signal to the lateral mitral annular early diastolic velocity (mitral E/Ea) as a noninvasive measure of LV filling pressure. Nagueh and colleagues demonstrated a significant correlation of mitral E/Ea with invasively measured mean pulmonary capillary wedge pressure, while subsequent studies have further validated this ratio and reported its applicability in a variety of hemo-dynamic settings ( Fig. 25-5 ). Additional novel indices of LV diastolic function using tissue Doppler echocardiography have recently been reported that may further expand the role of this modality in the clinical evaluation of LV filling pressures.


Tissue Doppler also is of considerable clinical value in the differentiation of constrictive from restrictive LV filling (see Chapter 24 ). Evaluation of patients with constrictive pericarditis and restrictive cardiomyopathy with two-dimensional echocardiography and even invasive cardiac catheterization may fail to confidently differentiate between these two disease states. Because the myocardium in patients with constrictive pericarditis is commonly normal, the corresponding tissue Doppler velocities are also normal. However, patients with restrictive cardiomyopathy have significantly decreased early diastolic and systolic tissue Doppler velocities, allowing separation of these two distinct clinical entities.
Tissue Doppler Studies in Children with Healthy Hearts
To date, a number of studies have established baseline reference values of tissue Doppler velocities in children ( Tables 25-4 and 25-5 ). , Similar to previously published adult reports, pediatric tissue Doppler velocities vary with age, heart rate, wall location, and myocardial layer ( Fig. 25-6 ). In addition, pulsed-wave tissue Doppler velocities are highly correlated with parameters of cardiac growth, most notably LV end diastolic dimension and LV mass, with the most significant changes in these velocities occurring during the first year of life ( Fig. 25-7 ). In a recently published large study of infants and children, tissue Doppler velocities did not correlate significantly with other more commonly utilized measures of systolic and diastolic ventricular performance, including LV shortening fraction, LV and RV myocardial performance indices, and transmitral inflow Doppler. This lack of correlation is likely due in part to pulsed-wave tissue Doppler assessments of longitudinal ventricular function, while other, more traditional, two-dimensional and Doppler methods assess radial and global measures of ventricular performance.
DEMOGRAPHICS | <1 yr | 1-5 yrs | 6-9 yrs | 10-13 yrs | 14-18 yrs | TOTAL |
---|---|---|---|---|---|---|
N | 63 | 68 | 55 | 58 | 81 | 325 |
Male | 29 | 39 | 27 | 38 | 44 | 177 |
Age (y) | 0.40 ± 0.30 | 3.05 ± 1.51 | 7.91 ± 1.12 | 11.99 ± 1.11 | 16.0 ± 1.40 | 7.8 ± 6.0 |
Weight (kg) | 6.6 ± 2.7 | 15.1 ± 5.4 | 33.8 ± 14.9 | 47.2 ± 16.3 | 66.1 ± 15.5 | 33.3 ± 25.2 |
BSA (m 2 ) | 0.34 ± 0.08 | 0.62 ± 0.14 | 1.07 ± 0.27 | 1.37 ± 0.29 | 1.73 ± 0.25 | 1.0 ± 0.6 |
HR (bpm) | 124 ± 16 | 105 ± 17 | 80 ± 11 | 75 ± 12 | 69 ± 16 | 90 ± 26 |
Echocardiographic | ||||||
LV EDD (cm) | 2.3 ± 0.3 | 3.1 ± 0.4 | 3.9 ± 0.4 | 4.3 ± 0.4 | 4.7 ± 0.4 | 3.6 ± 1.0 |
LV ESD (cm) | 1.4 ± 0.2 | 1.9 ± 0.3 | 2.4 ± 0.3 | 2.7 ± 0.3 | 3.0 ± 0.4 | 2.3 ± 0.6 |
LV PWT (cm) | 0.4 ± 0.1 | 0.6 ± 0.1 | 0.7 ± 0.1 | 0.8 ± 0.1 | 0.9 ± 0.2 | 0.7 ± 0.2 |
LV SWT (cm) | 0.5 ± 0.1 | 0.6 ± 0.1 | 0.8 ± 0.1 | 0.8 ± 0.2 | 1.0 ± 0.2 | 0.7 ± 0.2 |
LV mass (g/m 2 ) | 18.9 ± 6.5 | 43.6 ± 16.4 | 82.3 ± 28.3 | 110.1 ± 32.9 | 158.4 ± 48.5 | 81.8 ± 58.9 |
Mitral E velocity | 79.7 ± 18.8 | 95.2 ± 19.5 | 94.4 ± 14.8 | 94.5 ± 16.0 | 90.3 ± 17.8 | 90.8 ± 18.5 |
Mitral A velocity | 65.3 ± 13.3 | 61.3 ± 12.1 | 49.4 ± 12.5 | 49.5 ± 13.8 | 45.5 ± 13.2 | 54.4 ± 15.0 |
Mitral E/A ratio | 12.4 ± 0.30 | 1.60 ± 0.46 | 1.99 ± 0.51 | 2.02 ± 0.58 | 2.13 ± 0.65 | 1.79 ± 0.61 |
PV S-wave velocity | 44.6 ± 10.3 | 48.0 ± 8.9 | 50.7 ± 11.3 | 49.0 ± 11.1 | 47.7 ± 7.3 | 48.7 ± 9.2 |
PV D-wave velocity | 46.0 ± 9.5 | 54.5 ± 11.0 | 53.3 ± 11.4 | 58.4 ± 12.1 | 57.9 ± 15.0 | 54.6 ± 12.9 |
PV A-reversal velocity | 16.4 ± 6.3 | 20.6 ± 4.3 | 20.2 ± 3.8 | 21.2 ± 4.9 | 20.0 ± 5.2 | 20.5 ± 5.1 |
Tricuspid E velocity | 53.3 ± 12.3 | 61.6 ± 12.5 | 60.5 ± 13.9 | 59.6 ± 11.4 | 60.4 ± 10.9 | 59.2 ± 12.4 |
Tricuspid A velocity | 53.2 ± 13.0 | 48.3 ± 12.3 | 42.4 ± 10.8 | 39.2 ± 11.3 | 34.5 ± 11.2 | 43.3 ± 13.5 |
Tricuspid E/A ratio | 1.01 ± 0.38 | 1.27 ± 0.31 | 1.49 ± 0.40 | 1.61 ± 0.47 | 1.88 ± 0.56 | 1.47 ± 0.53 |
SF (%) | 38.9 ± 4.1 | 38.0 ± 3.6 | 37.4 ± 3.8 | 37.4 ± 4.2 | 36.4 ± 4.3 | 37.6 ± 4.1 |
LV MPI | 0.33 ± 0.08 | 0.34 ± 0.07 | 0.32 ± 0.06 | 0.34 ± 0.06 | 0.34 ± 0.08 | 0.33 ± 0.08 |
RV MPI | 0.29 ± 0.09 | 0.28 ± 0.07 | 0.29 ± 0.08 | 0.28 ± 0.08 | 0.29 ± 0.08 | 0.28 ± 0.08 |
AGE GROUP | N | E * -WAVE VELOCITY | A * -WAVE VELOCITY | S * -WAVE VELOCITY | ICT | IRT | E/E * RATIO |
---|---|---|---|---|---|---|---|
Mitral annular | |||||||
<1 yr | 63 | 9.7 ± 3.3 (8.8-10.5) | 5.7 ± 1.8 (5.3-6.2) | 5.7 ± 1.6 (5.3-6.1) | 77.4 ± 18.4 (72.7-82.0) | 57.0 ± 14.8 (53.1-60.8) | 8.8 ± 2.7 (8.1-9.5) |
1 — 5 yrs | 68 | 15.1 ± 3.4 † (14.3-15.4) | 6.5 ± 1.9 (6.1-7.0) | 7.7 ± 2.1 † (7.2-8.2) | 76.9 ± 15.9 (72.8-80.9) | 62.1 ± 13.2 (58.9-65.4) | 6.5 ± 2.0 † (6.0-7.0) |
6-9 yrs | 55 | 17.2 ± 3.7 † (16.2-18.3) | 6.7 ± 1.9 (6.2-7.3) | 9.5 ± 2.1 † (8.9-10.1) | 77.9 ± 18.9 (72.4-83.4) | 62.9 ± 11.9 (59.5-66.3) | 5.8 ± 1.9 (5.3-6.4) |
10—13 yrs | 58 | 19.6 ± 3.4 † (18.7-20.5) | 6.4 ± 1.8 (5.9-6.9) | 10.8 ± 2.9 * (10.0-11.5) | 76.6 ± 16.2 (72.4-80.9) | 62.6 ± 12.4 (59.4-65.9) | 4.9 ± 1.3 (4.6-5.2) |
14-18 yrs | 81 | 20.6 ± 3.8 (19.7-21.4) | 6.7 ± 1.6 (6.3-7.1) | 12.3 ± 2.9 † (11.6-12.9) | 78.9 ± 15.4 (75.4-82.3) | 69.5 ± 15.5 * (66.1-73.0) | 4.7 ± 1.3 (4.4-5.0) |
Total | 325 | 16.5 ± 5.3 (16.0-17.1) | 6.4 ± 1.9 (6.2-6.6) | 9.3 ± 3.4 (8.9-9.7) | 77.5 ± 16.7 (75.7-79.5) | 63.2 ± 14.4 (61.7-64.9) | 6.1 ± 2.4 (5.9-6.4) |
Septal | |||||||
<1 yr | 63 | 8.1 ± 2.5 (7.5-8.7) | 6.1 ± 1.5 (5.7-6.4) | 5.4 ± 1.2 (5.1-5.7) | 77.5 ± 17.5 (73.0-82.0) | 53.0 ± 11.7 (50.0-56.0) | 10.3 ± 2.7 (9.7-11.0) |
1 — 5 yrs | 68 | 11.8 ± 2.0 † (11.3-12.3) | 6.0 ± 1.3 (5.7-6.4) | 7.1 ± 1.5 † (6.8-7.5) | 80.1 ± 15.5 (76.3-83.9) | 59.8 ± 12.0 (56.9-62.7) | 8.1 ± 1.8 † (7.7-8.5) |
6-9 yrs | 55 | 13.4 ± 1.9 † (12.8-13.9) | 5.9 ± 1.3 (5.5-6.3) | 8.0 ± 1.3 (7.6-8.4) | 82.8 ± 15.3 (78.4-87.2) | 65.6 ± 10.7 (62.5-68.7) | 7.2 ± 1.6 (6.8-7.7) |
10—13 yrs | 58 | 14.5 ± 2.6 (13.8-15.2) | 6.1 ± 2.3 (5.6-6.7) | 8.2 ± 1.3 (7.9-8.5) | 87.9 ± 16.4 * (83.6-92.2) | 72.5 ± 12.3 (69.3-75.8) | 6.6 ± 1.4 (6.3-7.0) |
14-18 yrs | 81 | 14.9 ± 2.4 (14.3-15.4) | 6.2 ± 1.5 (5.9-6.6) | 9.0 ± 1.5 (8.7-9.3) | 88.4 ± 15.6 (84.9-91.9) | 77.5 ± 14.5 (74.3-80.8) | 6.4 ± 1.5 (6.1-6.8) |
Total | 325 | 12.6 ± 3.4 (12.2-13.0) | 6.1 ± 1.6 (5.9-6.3) | 7.6 ± 1.9 (7.4-7.8) | 83.5 ± 16.5 (81.7-85.4) | 66.1 ± 15.3 (64.4-67.9) | 7.7 ± 2.3 (7.5-8.0) |
Tricuspid annular | |||||||
<1 yr | 63 | 13.8 ± 8.2 (11.7-15.9) | 9.8 ± 2.4 (9.1-10.5) | 10.2 ± 5.5 (8.8-11.7) | 68.7 ± 18.2 (63.9-73.5) | 52.0 ± 12.9 (48.5-55.4) | 4.4 ± 2.3 (3.8-5.0) |
1 — 5 yrs | 68 | 17.1 ± 4.0 † (16.1-18.1) | 10.9 ± 2.7 (10.2-11.6) | 13.2 ± 2.0 † (12.7-13.7) | 77.7 ± 15.0 (73.9-81.5) | 59.0 ± 13.9 (55.4-62.5) | 3.8 ± 1.1 (3.5-4.1) |
6-9 yrs | 55 | 16.5 ± 3.0 (15.7-17.4) | 9.8 ± 2.7 (9.0-10.6) | 13.4 ± 2.0 (12.8-14.0) | 91.8 ± 21.5 † (85.5-98.0) | 58.5 ± 17.5 (53.4-63.6) | 3.6 ± 0.8 (3.4-3.9) |
10—13 yrs | 58 | 16.5 ± 3.1 (15.7-17.4) | 10.3 ± 3.4 (9.3-11.2) | 13.9 ± 2.4 (13.2-14.5) | 98.1 ± 21.7 (92.2-103.9) | 61.7 ± 19.9 (56.4-67.1) | 3.5 ± 1.4 (3.2-3.9) |
14-18 yrs | 81 | 16.7 ± 2.8 (16.0-17.3) | 10.1 ± 2.6 (9.5-10.7) | 14.2 ± 2.3 (13.7-14.7) | 101.9 ± 20.4 (97.2-106.6) | 62.9 ± 18.9 (58.5-67.3) | 3.7 ± 1.0 (3.5-3.9) |
Total | 325 | 16.1 ± 4.7 (15.6-16.7) | 10.2 ± 2.8 (9.9-10.5) | 13.0 ± 3.4 (12.6-13.4) | 88.2 ± 23.1 (85.6-90.8) | 59.0 ± 17.2 (57.0-60.9) | 3.8 ± 1.4 (3.6-4.0) |
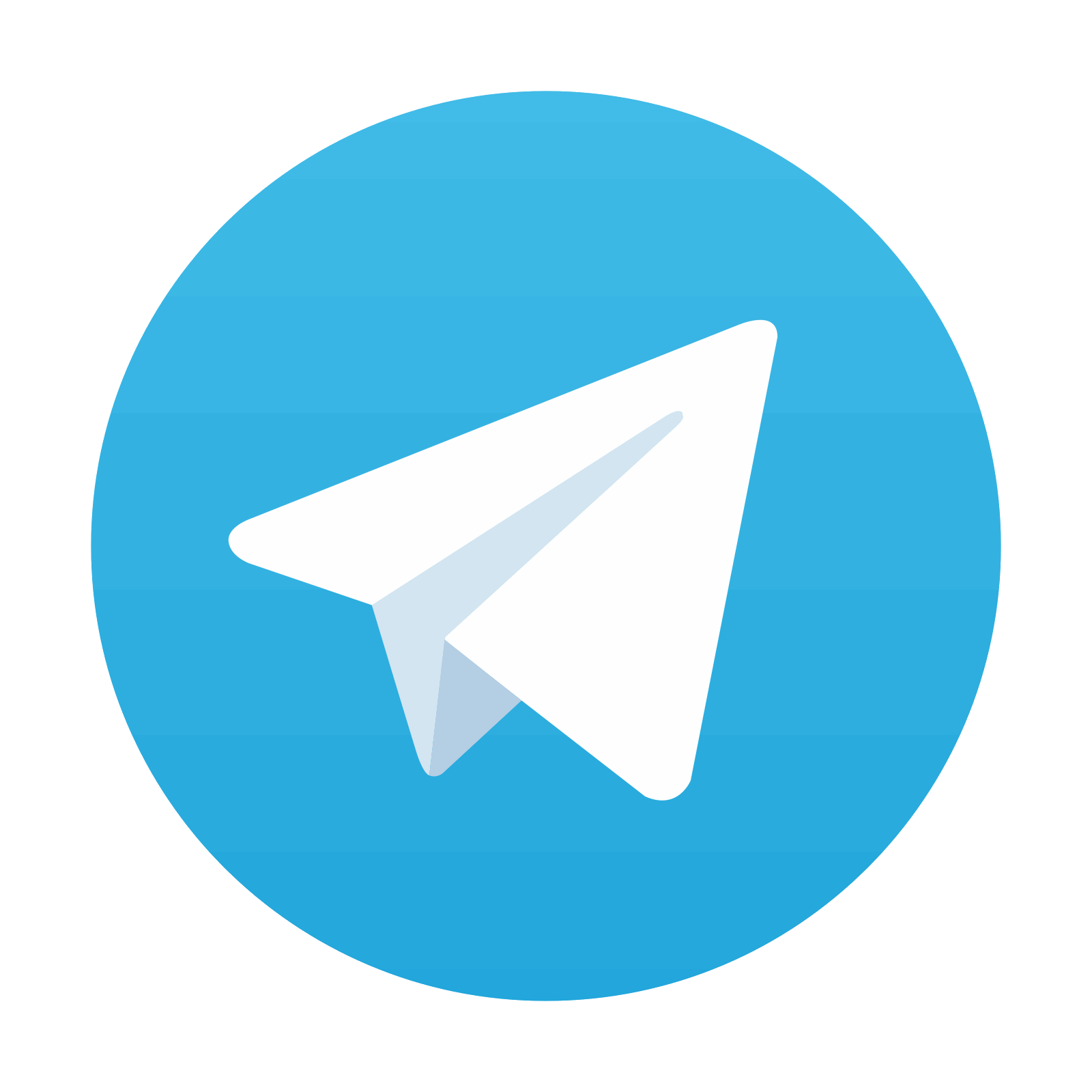
Stay updated, free articles. Join our Telegram channel

Full access? Get Clinical Tree
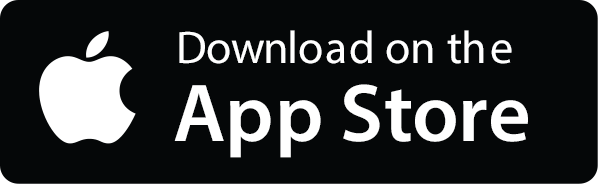
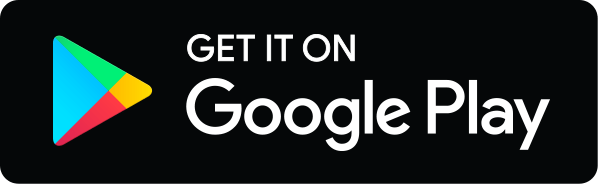
