During the first 20 days of development, the human embryo has no cardiovascular structures. However, very rapid and intricate development takes place over the next month to the point that the morphology of the heart and great vessels is nearly that of a term infant. This process transforms isolated angiogenic cell islets into a complex, 4-chambered heart. The single heart tube begins to beat at 23 days of gestation and blood circulates through the embryo by 30 days.1
Prior to embryonic folding, there is coalescence of angiogenic cell clusters on either side of the neural crest to form “cardiac cords.” These soon canalize to form endothelial-lined capillaries in the mesoderm of the germ disk at about day 20. These capillaries then join to form pairs of blood vessels, with 1 pair on each side of the neural crest. As the embryo folds in its lateral dimension and acquires a tubular form, 2 outer primitive vessels meet in the median of the embryo (ventral to the primitive gut) and start fusing cranially to caudally. This forms the primitive heart tube, which is complete by about day 23.
The single tubular heart develops constrictions that outline future structures: the bulbus cordis, the primitive ventricle, and the primitive atrium. The cranial-most area is the bulbus cordis, which extends cranially into the truncus arteriosus. The truncus arteriosus connects cranially to the aortic sac and through the aortic arches to the dorsal aorta. Caudal to the bulbus cordis is the primitive ventricle, which connects to the primitive atrium. The atrium communicates with the sinus venosus, which receives the vitelline veins, cardinal veins, and umbilical veins.
At approximately day 23 of gestation, the heart tube begins to form a loop, such that the cephalic end bends ventrally, caudally, and slightly to the right. This process results in a U-shaped heart, with the bulbus cordis on the right and the ventricle (ventricularis) on the left. At the junction of the bulbus cordis and the ventricle, the bulboventricular fold forms. The atrium and sinus venosus are dorsal to the heart loop. If the primitive heart tube loops to the left rather than the right, L-transposition results (see page 349).
As the cardiac looping progresses, the paired atria form a common chamber and move into a more dorsal and cranial position. The common atrioventricular junction becomes the atrioventricular canal, connecting the left side of the common atrium to the primitive ventricle. The primitive ventricle will eventually become the left ventricle and the proximal portion of the bulbus cordis will develop into the right ventricle. The distal part of the bulbus cordis will form the outflow tracts of the ventricles. The truncus arteriosus will form the roots of the great vessels. The bulbus cordis gradually migrates medially until it is located in the sulcus between and anterior to the 2 atria.
By day 21 of gestation, a common atrium has formed by way of fusion of the 2 endocardial tubes. The common atrium communicates with left and right sinus horns that represent the unfused ends of the endocardial tubes. These sinus horns form the sinus venosus, which is located dorsal to the atria. Various major fetal veins drain into the sinus venosus: the common cardinal veins (draining most of the embryo), the umbilical veins (from the placenta), and the vitelline veins (draining the yolk sac, GI system, and portal circulation). The anterior cardinal veins drain the cranial part of the embryo, and the posterior cardinal veins drain the caudal part of the embryo. The anterior and posterior cardinal veins join to form the common cardinal veins. The major systemic veins develop from portions of the sinus venosus and these fetal veins.
The sinus venosus initially communicates with the common atrium. Subsequently (week 7), the sinoatrial communication becomes more right-sided, such that it connects to the dorsal aspect of the right atrium. During this process, the right horn of the sinus venosus incorporates into the posterior wall of the right atrium. The distal end of the left cardinal vein degenerates. The more proximal portion of this vessel connects through the developing left brachiocephalic vein to the right anterior cardinal vein (i.e., the future right brachiocephalic vein), thereby forming the superior vena cava. The left posterior cardinal vein degenerates. The left sinus horn becomes the coronary sinus. The right vitelline vein becomes the inferior vena cava and the right posterior cardinal vein becomes the azygos vein. The left umbilical vein degenerates and the right umbilical vein connects to the vitelline system through the ductus venosus.
Double superior vena cavae occur as a consequence of persistence of the left anterior cardinal vein. A left superior vena cava apparently results from persistence of the left anterior cardinal vein and the left common cardinal vein, in conjunction with degeneration of the right anterior cardinal vein and the common cardinal vein. The hepatic segment of the inferior vena cava develops from the proximal part of the right vitelline vein and hepatic sinusoids; failure of development of this segment occurs in approximately 50% of fetuses with polysplenia. Double inferior vena cavae likely occur because of failure of degeneration of the inferior aspect of the left supracardinal vein.
The proximal portion of the main pulmonary artery develops from the truncus arteriosus. The distal portion of the main pulmonary artery and the proximal aspect of the right pulmonary artery develop from the ventral sixth aortic arch artery. The ductus arteriosus and the proximal segment of the left pulmonary artery arise from the sixth aortic arches. The remainder of the left pulmonary artery and the distal aspect of the right pulmonary artery develop from vessels that arise from the lung buds and surrounding mesoderm. The ductus arteriosus arises from the distal left sixth aortic arch artery.
The process by which the pulmonary veins incorporate into the left atrium is relatively complex. The early embryonic venous return from the lungs is to the cardinal and splanchnic systems. Eventually, individual pulmonary veins develop and form a confluence (the pulmonary venous plexus) immediately dorsal to the left atrium. The common pulmonary vein grows from the left atrium, and by week 5 of gestation connects with the pulmonary venous plexus. This direct connection allows atrophy of primitive complex systemic venous channels and provides pulmonary venous drainage to the left atrium through 4 central parts of the pulmonary vein (right upper, right lower, left upper, and left lower). Anomalous pulmonary venous return apparently results from abnormal development of the common pulmonary vein and persistence of the embryological pulmonary-to-systemic venous anastomosis.
The atria arise from multiple embryonic structures. The primitive atria give rise to the trabeculated atrial appendages. The smooth walled portions of the atria derive from incorporated venous structures. The posterior aspect of the left atrium forms from pulmonary vein tissue, while the posterior aspect of the right atrium develops from the sinus venosus. Division of the atrial chamber into 2 components occurs during gestation week 6.
The primitive common atrium communicates with the primitive ventricle through the atrioventricular canal. Initially located at the midline, the atrioventricular canal migrates toward the right. Concomitant with this migration, the bulbus cordis expands to develop into the right ventricle and the ventricularis (primitive ventricle) differentiates into the left ventricle. Endocardial cushion tissue forms bulges at the atrioventricular junction, one dorsal and the other ventral. The endocardial cushions grow toward each other and eventually fuse, thereby dividing the common atrioventricular canal into right and left channels. The septal surfaces of the endocardial cushion tissue form the septal leaflets of the mitral and tricuspid valves. The other leaflets of the atrioventricular valves arise from tissue surrounding the annuli.
The common atrium divides into 2 chambers in a series of steps. The septum primum arises as a thin crescentic structure from the cranial aspect of the atrium and grows toward the endocardial cushions. The opening between the leading edge of the septum and the endocardial cushions is termed the ostium primum. Prior to fusion of the septum primum with the endocardial cushions, fenestrations develop in the midportion of the septum. These fenestrations coalesce to form a single large opening, termed the ostium secundum. The septum secundum is an additional septum that arises along the cranial and posterior wall of the right atrium. This grows toward the endocardial cushions but does not fuse with them. The crescent-shaped lower margin of the septum secundum forms the foramen ovale.
Simultaneous to atrial development, differentiation of the right and left ventricles from the bulbus cordis and ventricularis is occurring. Development of the muscular interventricular septum begins on approximately day 30, as a fold of tissue that arises near the apex and grows toward the developing atrioventricular valves. Coalescence of tissue from the inlet region forms a second septum that fuses with the primary septum. Initially, the interventricular foramen allows communication between the 2 ventricles; the interventricular foramen is located between the developing interventricular septum and the fusing endocardial cushions. The foramen is closed by endocardial cushion tissue and by the bulboventricular ridges.
A ventricular septal defect can result from failure of fusion at any stage in the process of separation of the embryonic ventricle into 2 chambers. Various forms of atrial septal communications can occur. In the normal situation, elevation of the left atrial pressure after birth causes the valve of the foramen ovale to close. Conditions that cause elevated right atrial pressure may prevent this process. In addition, maldevelopment of the valve can result in a persistent communication; that is, an ostium secundum atrial septal defect. An ostium primum atrial septal defect is a result of nonfusion of the septum primum with the septum intermedium; the pathophysiology often involves maldevelopment of the endocardial cushions. Failure of appropriate septation in other portions of the atrium can also occur. Complete lack of formation of the atrial septum results in single atrium.
Abnormal development of the endocardial cushions produces a spectrum of cardiac malformations. Because this tissue contributes to atrioventricular valve formation, minor disruption in endocardial cushion development can produce an isolated valve cleft. More substantial maldevelopment can lead to an ostium primum atrial septal defect, a membranous ventricular septal defect, and/or insufficiency of the tricuspid or mitral valves.
Mitral atresia represents the most severe form of mitral valve maldevelopment. The endocardial cushions contribute to the supporting structures for the mitral valve. The valve itself is composed of an outer layer of atrial tissue, and inner layer of ventricular tissue, and a middle layer of invaginated sulcus tissue. Reduced inflow into the left ventricle in the fetus with mitral atresia results in deficient development of the ventricle.
Embryonic structures that contribute to formation of the tricuspid valve include a portion of the interventricular septum, the endocardial cushions, and a portion of the right ventricular wall. If there is a disturbance in the balance between proliferation and resorption of these tissues, failure of appropriate valve leaflet formation occurs. In the most severe form, tricuspid atresia, there is no vestige of valvar tissue and there is no communication between the right atrium and right ventricle.
The truncus arteriosus and bulbus cordis are divided by a pair of tissue ridges that grow toward the center of the truncus and fuse in the midline; these are termed the bulbar ridges. Midline fusion of the bulbar ridges divides the truncus into equal sized aortic and pulmonary arteries. The septum develops in a spiral pattern; failure of appropriate spiraling alters the relationship between the great arteries and ventricular chambers and leads to D-transposition (see page 346). Growth of the bulbar ridges across the junction between the truncus arteriosus and the developing ventricular mass serves to divide the truncal valve into separate aortic and pulmonary components. Additional inferior extension of the bulbar ridges contributes to closure of the interventricular foramen.
If septation of the truncus arteriosus fails, a single great vessel remains, receiving all the cardiac output from the left and right ventricles; this is termed persistent truncus arteriosus. Because the aorticopulmonary septum participates in the final closure of the ventricular septum, a ventricular septal defect is always present with this anomaly. Failure of normal separation of the truncus into the aorta and pulmonary artery can also result in aortopulmonary window and aortic origin of a pulmonary artery. Abnormal partitioning of the truncus arteriosus is also a component of various other anomalies of the heart and great vessels, such as tetralogy of Fallot, pulmonary stenosis, pulmonary atresia, aortic stenosis, and aortic atresia.
The cranial aspect of the truncus arteriosus connects with the aortic sac, and the aortic sac connects with paired aortic arches. The embryonic aortic arches connect with the right and left dorsal aortae. A series of 6 paired aortic arches forms in succession, from cranial to caudal. Most components involute, also in a cranial-to-caudal temporal progression. Some portions persist, however, and contribute to formation of various mature arteries. Aortic arch I: portions of the maxillary arteries; aortic arch II: portions of the stapedial arteries; aortic arch III: the common carotid arteries; aortic arch IV: the left side of the aortic arch and a portion of the right subclavian artery; aortic arch V: no mature components; right aortic arch VI: a portion of the right pulmonary artery (± right-sided ductus arteriosus); left aortic arch VI: the ductus arteriosus and proximal portion of the left pulmonary artery. Concomitant with development and involution of the 6 paired arches, the paired dorsal aortae fuse into a single vessel. This process begins in the abdomen and progresses into the thorax to form a left-sided descending aorta.
Failure of involution of the distal portion of the right dorsal aorta results in a double aortic arch. Persistence of the entire right dorsal aorta and involution of the distal segment of the left dorsal aorta leads to a right aortic arch. Anomalous origin of the right subclavian artery occurs when the vessel forms from the right seventh intersegmental artery and the distal aspect of the right dorsal aorta. This alteration apparently is caused by abnormal involution of the right fourth aortic arch and the right dorsal aorta cranial to the seventh intersegmental artery.
Each component of the sixth aortic arch gives off a branch that grows toward the developing lung. On the right, the proximal portion of this branch becomes the proximal portion of the right pulmonary artery and the distal portion regresses. On the left, the proximal portion becomes the proximal aspect left pulmonary artery. The distal portion on the left maintains union with the aorta and thereby forms the ductus arteriosus.
The aortic valve develops by enlargement of 3 tubercles in the lumen of the aorta that grow toward the center and ultimately undergo thinning by resorption of excess tissue. Hollowing out of tissue along the superior portion of the tubercle at its junction with aortic wall gives rise to the sinuses of Valsalva. Interruption of this process may result in aortic stenosis or atresia and hypoplastic left heart syndrome.
Cine cardiac MRI with electrocardiographically gated sequences provides dark-blood and bright-blood multiphase images that demonstrate cardiac wall motion and valvular motion. Cine images can be acquired in any plane. Contrast-enhanced MR angiography is useful for depicting the regional thoracic vasculature. The resulting three-dimensional (3D) dataset allows creation of 3D volume-rendered images to display complex cardiovascular anatomy or subvolume maximum-intensity projection reformatted images of specific vessels in any plane.2
There are various MR techniques for the measurement of the velocity and volume of blood flow. Velocity encoded phase-contrast MR (also termed phase-shift or VEC) makes use of the principle that magnetic spins of intravascular protons that have been exposed to a magnetic field gradient acquire a phase shift proportional to their flow velocities. Two sets of images are acquired, 1 with velocity encoding and 1 without. Subtraction of the 2 sets of phase images depicts the phase shift that is proportional to the flow velocity in the direction of the flow compensation gradient. The magnitude of the phase shift for each pixel is displayed such that antegrade flow along the encoding axis is bright, retrograde flow is dark, and stationary objects are gray (Figure 11-1). The velocity can be measured in planes that are perpendicular to the direction of flow, parallel to the direction of flow, or in 3 dimensions.3–5
Figure 11–1
Phase contrast MR.
A. An axial phase contrast image at the level of the great vessels shows bright antegrade flow in the ascending aorta (A) and main pulmonary artery (P). B. During diastole there is a signal void in the pulmonary artery (arrow) because of retrograde flow in this patient with severe pulmonary insufficiency.

Phase-contrast imaging allows the calculation of absolute velocity at locations in the plane of data acquisition at any point during the cardiac cycle. Utilizing cardiac gating, the flow volume for each time frame in a cardiac cycle is calculated. This information is accumulated for all of the pixels within a region of interest, as in the cross-section of a vessel or a valve annulus. This technique allows measurement of aortic and pulmonary arterial flow volume and thereby quantification of the pulmonary-to-systemic (Qp:Qs) flow ratio. Similarly, estimation of differential pulmonary flow is provided by measuring flow in each pulmonary artery or the main pulmonary artery and the right or left pulmonary artery. Calculation of the pressure gradient across a vascular stenosis is by use of the simplified Bernoulli equation: ΔP = 4(Vmax)2, where Vmax is the measured maximum poststenotic velocity.
Ventricular volumes and ejection fractions can be calculated from MR images obtained with spin echo or gradient-refocused echo pulse sequences. The most useful imaging planes are oblique planes that are defined by the short and long axes of the heart. The evaluation of cardiac function with spin-echo pulse sequences is performed with electrocardiographically gated multislice images acquired during various portions of the cardiac cycle. Alternatively, limiting image acquisition to end-systole and end-diastole can shorten the duration of the study. Gradient-refocused echo pulse sequences provide improved temporal resolution over standard spin-echo images as the shorter time-to-repetition (TR) and echo (TE) times and smaller flip angles allow the accumulation of greater data per unit time.
The calculation of ventricular volumes is either by direct measurement of the blood within the ventricle on the 3D dataset or by using geometric models to estimate ventricular volume from single images in 1 or 2 planes. With the first method, the intraventricular blood is traced with a region of interest at each level and the volumes of consecutive sections through the entire 3D dataset are determined. Subtracting the end systolic volume from the diastolic volume provides the ejection fraction. With the geometric model method, the ventricular volume is calculated from single images in the long axis plane of the heart.
Gradient-refocused echo MR images readily demonstrate turbulent flow distal to a stenotic valve as a signal void. Peak flow velocity and the gradient across the abnormal valve can be determined with MR velocity mapping techniques. A regurgitation jet also produces a signal void on gradient-refocused echo images. Measuring the size of the signal void provides a semiquantitative estimate of the regurgitant volume. If there is only 1 regurgitant valve, the volume of regurgitation can also be calculated from the difference between the left ventricular and right ventricular stroke volumes.
The volume of left-to-right shunting at the atrial level can be determined with MR by calculating the difference between the right ventricular and left ventricular stroke volumes. This technique is not valid, however, in patients with multiple shunts or with shunting at the ventricular level. In this situation, MR velocity mapping allows assessment of the right ventricular and left ventricular stroke volumes. In a patient with an isolated ventricular septal defect, the difference between the right ventricular and left ventricular stroke volumes represents the shunt volume; in patients with complex shunts, this measurement indicates the net shunt volume.6
Myocardial perfusion assessment with contrast-enhanced MR is useful in patients with suspected myocardial infarction or ischemia, as occurs with thromboembolism, Kawasaki disease, cardiac surgery, and coronary artery anomalies. Ischemic myocardium fails to enhance with intravenously administered contrast on dynamically acquired images (i.e., first-pass perfusion imaging). Subsequent hyperintensity at the ischemic site on delayed images (late gadolinium enhancement) is compatible with myocardial infarction (Figure 11-2).7
Figure 11–2
Myocardial infarction.
A. There is markedly deficient myocardial enhancement at the apex of the left ventricle (arrow) on this first-pass MR perfusion image. B. A delayed image shows enhancement of the scar tissue at the apex (arrows) and appropriate washout from normal myocardial tissue elsewhere. Infarction in this patient occurred 3 years earlier during mitral valve surgery.

Gated blood pool scintiangiography (multigated acquisition) provides qualitative and quantitative information about heart function. This study is performed with 99mtechnetium (99mTc)-labeled autologous red blood cells. Cine image display allows evaluation of regional wall motion. Computer analysis provides measurements of ventricular ejection fractions, stroke volume, end-diastolic volume, end-systolic volume, and cardiac output.
Myocardial perfusion scintigraphy is useful for select children with suspected myocardial ischemia or infarction. Potential causes of myocardial ischemia in children include coronary artery anomalies, Kawasaki disease, cardiomyopathy, and arterial switch procedures. Radiopharmaceuticals that can be utilized for perfusion imaging include thallium-201, 99mTc-sestamibi, and 99mTc-tetrofosmin. The injection is usually performed during exercise-induced stress or pharmacologically induced stress.
Scintigraphy utilizing radiolabeled autologous leukocytes or gallium-67 is useful for evaluating selected patients with suspected myocardial or pericardial inflammation.
Congenital cardiac anomalies occur in approximately 5 to 8 per 1000 livebirths. Congenital heart lesions that present in the neonatal period often have unique clinical and imaging features. Although there are many cardiac lesions that can produce symptoms in the newborn, 9 anomalies account for approximately three-fourths of the lesions that typically cause cyanosis or respiratory distress in these infants (Table 11-1).
Pulmonary atresia (or severe stenosis) with intact ventricular septum | Cyanotic |
Pulmonary atresia (or severe stenosis) with ventricular septal defect | Cyanotic |
Ebstein malformation | Cyanotic |
Tricuspid atresia | Cyanotic |
D-transposition of the great arteries | Cyanotic |
Coarctation syndrome | Cyanotic |
Total anomalous venous connection with obstruction | Cyanotic |
Hypoplastic left heart syndrome | Acyanotic |
Severe coarctation of aorta | Acyanotic |
Consideration of various clinical factors helps to narrow the differential diagnosis for neonates with suspected congenital heart disease. The combination of cyanosis and mild respiratory distress is common with transposition of the great arteries, pulmonary atresia, severe pulmonary stenosis and tricuspid atresia. Severe respiratory distress with only minimal cyanosis comprises the typical clinical presentation of hypoplastic left heart syndrome and total anomalous pulmonary venous connection with obstruction. A systolic murmur is usually present in infants with coarctation syndrome, Ebstein malformation, or tricuspid atresia. A substantial murmur is usually lacking in association with transposition of the great arteries or hypoplastic left heart syndrome. Reduced femoral pulse pressures in comparison to those of the upper extremities are suggestive of an aortic lesion, such as coarctation.
An important categorizing feature provided by chest radiographs of newborns with a suspected cardiac anomaly is the pulmonary vascular pattern. The usual radiographic features of diminished pulmonary vasculature are a diminished number and size of pulmonary vessels in the outer third of the lung, a right pulmonary artery that is smaller than the trachea, and a diminutive right pulmonary artery as viewed on the lateral radiograph. Diminished pulmonary vasculature suggests the presence of a right-heart outflow obstruction in association with a right-to-left shunt. The right-heart obstruction can occur at any location from the tricuspid valve to the peripheral aspects of the pulmonary arteries. The obstruction results in elevation of right ventricular pressure and, in the presence of an intracardiac communication, shunting of blood from the right heart to the left heart. This right-to-left shunting produces the clinical finding of cyanosis. If a right-to-left shunt is not present, the pulmonary vascular pattern is normal despite the presence of a right-heart outflow obstruction.8,9
Table 11-2 lists the common cardiac anomalies that include right-heart outflow tract obstruction and a right-to-left shunt (cyanosis). These include tetralogy of Fallot and the various forms of hypoplastic right heart syndrome, such as tricuspid atresia, tricuspid stenosis, pulmonary atresia, isolated hypoplastic right ventricle, and Ebstein malformation. Similar findings can occur in infants with pulmonary atresia or severe pulmonary stenosis in combination with transposition of the great arteries or single ventricle.
Cardiac anomaly | Severity of cardiomegaly |
---|---|
Tetralogy of Fallot | None or minimal |
Tricuspid atresia/stenosis | None or minimal |
Pulmonary atresia/stenosis with intact ventricular septum | Moderate to marked |
Pulmonary atresia/stenosis with ventricular septal defect | None or minimal |
Ebstein anomaly | Marked |
Transposition of the great arteries with pulmonary atresia/stenosis | Mild |
Single ventricle with pulmonary atresia/stenosis | None of minimal |
Heart size is an additional characterizing radiographic feature in neonates with congenital heart disease. The most common lesions that include cyanosis, decreased pulmonary vascularity, and substantial cardiomegaly in the neonate are Ebstein malformation and pulmonary atresia or severe pulmonary stenosis with an intact ventricular septum. With Ebstein malformation, cardiomegaly is often marked and the right atrium in particular is enlarged. If heart size is normal or there is only mild cardiomegaly in a cyanotic neonate with decreased pulmonary vascularity, the most likely diagnosis is tricuspid atresia or pulmonary atresia/severe stenosis with a ventricular septal defect.
Several congenital heart lesions are associated with increased pulmonary vascularity on chest radiographs. In general, cyanosis only occurs in these disorders if venous blood is directed into the systemic circulation. The radiographic pattern of pulmonary vascular prominence is characterized as either active or passive. The usual cause of passive congestion is either left heart failure or pulmonary venous obstruction. Active vascular prominence indicates an abnormally increased volume of blood flowing through the lungs, due to preferential pulmonary blood flow or left-to-right shunting (Table 11-3). With left-to-right shunting, recirculation of blood through the heart and lungs causes volume overload of the pulmonary circulation.
Cardiac anomaly | Severity of cardiomegaly |
---|---|
D-transposition | Mild to moderate |
Taussig Bing anomaly | Moderate |
Double outlet right ventricle | Moderate to marked |
Total anomalous pulmonary venous connection, unobstructed | Moderate to marked |
Truncus arteriosus | Moderate |
Endocardial cushion defect | Mild to moderate |
Single ventricle without pulmonary stenosis | Moderate |
Tricuspid atresia with transposition | Moderate to marked |
Important causes of passive pulmonary vascular congestion in the neonatal period include total anomalous pulmonary venous connection with obstruction (type III), hypoplastic left heart syndrome, severe isolated coarctation of aorta, interruption of the aortic arch, and critical aortic stenosis. The severity of cardiomegaly is an additional characterizing radiographic feature (Table 11-4). With hypoplastic left heart syndrome, the heart is typically normal in size for the first few days of life, after which it rapidly enlarges. Substantial cardiomegaly is already present at birth in infants with severe coarctation of the aorta or critical aortic stenosis. Passive congestion in association with a normal-size heart suggests a diagnosis of total anomalous pulmonary venous connection with obstruction.
Cardiac anomaly | Severity of cardiomegaly |
---|---|
Hypoplastic left heart syndrome | Initially normal |
Severe isolated coarctation of aorta | Moderate to marked |
Interruption of aortic arch | Moderate to marked |
Critical aortic stenosis | Moderate to marked |
Total anomalous pulmonary venous connection with obstruction | Normal |
Mitral stenosis or insufficiency | Normal |
Various congenital heart lesions typically have a normal pulmonary vascular pattern on chest radiographs during the neonatal period (Table 11-5). Examples include a small left-to-right shunt, an intracardiac admixture lesion, and an outflow obstruction of the right heart with no or minimal right-to-left shunting (e.g., pulmonary stenosis with intact ventricular septum). A neonate with right-heart outflow obstruction and a right-to-left shunt (causing cyanosis) may have a normal pulmonary vascular pattern if there is a patent ductus arteriosus that augments pulmonary blood flow. Infants with a left-sided obstructing lesion, such as aortic valvar stenosis or coarctation of aorta, usually have normal pulmonary vascularity if there is no associated left-to-right shunt; progression to cardiac decompensation and the onset of congestive heart failure may not occur until later in childhood. Other left-heart lesions that usually have a normal pulmonary vascular pattern in young infants include endocardial fibroelastosis, hypertrophic cardiomyopathy, and aberrant origin of the left coronary artery.
Cardiac anomaly | Severity of cardiomegaly |
---|---|
Small left-to-right shunt | None |
Aortic stenosis | Variable |
Mild to moderate coarctation, without left-to-right shunt | None |
Right outflow obstruction with patent ductus arteriosus | None |
Endocardial fibroelastosis | None |
Hypertrophic cardiomyopathy | Mild to severe |
Aberrant origin of the left coronary artery | Progressive |
Cayler cardiofacial syndrome refers to a specific asymmetric facial appearance, likened to a crying face, occurring in conjunction with a congenital heart lesion. The facial asymmetry (congenital asymmetric crying facies) is caused by congenital unilateral hypoplasia of the depressor anguli oris muscle, thereby impairing downward and outward motion of one corner of the mouth during crying or grimacing. The identification of the characteristic facial appearance should instigate careful evaluation for an associated cardiac defect, usually conotruncal heart disease. Cardiofacial syndrome is etiologically heterogeneous; some patients have 22q11.2 deletions.10–13
The 22q11 deletion syndrome includes DiGeorge syndrome, velocardiofacial syndrome, and Spritzen syndrome. There is extreme variability in the clinical manifestations of 22q11 deletion syndrome. Common features include congenital heart disease, abnormal facies (small mouth, tall nasal bridge, and bulbous nasal tip), thymic hypoplasia (and T-cell deficiency), cleft palate, and hypocalcemia (caused by hypoparathyroid hypoplasia); the term CATCH-22 refers to this constellation of abnormalities. Velopharyngeal insufficiency and leaning disabilities are common. Many patients with 22q11 deletion syndrome have skeletal anomalies. Vertebral anomalies in the upper portion of the cervical spine are common. Conotruncal heart malformations are common. Approximately one-third of infants with truncus arteriosus have 22q11 deletion. Other associated cardiac lesions are tetralogy of Fallot and pulmonary atresia with ventricular septal defect.14–16
CHARGE syndrome refers to coloboma of the eye, heart defects, atresia of the nasal choanae, retardation of growth/development, genital and/or urinary abnormalities, and ear anomalies/deafness. The most common responsible mutation is in CHD7 on chromosome 8. Various cardiac anomalies can occur in these patients; the most common are tetralogy of Fallot, patent ductus arteriosus, atrial septal defect, and ventricular septal defect.
The VACTERL association (VATER syndrome) includes vertebral anomalies, anal atresia, cardiovascular anomalies, tracheoesophageal fistula, esophageal atresia, renal and/or radial anomalies, and limb defects. Up to 75% of individuals with this constellation of birth defects have a cardiac anomaly. The most common heart defects are ventricular septal defect, atrial septal defect, tetralogy of Fallot, truncus arteriosus, and transposition of the great arteries.
Holt-Oram syndrome is caused by mutations in the TBX5 gene. The product of this gene is crucial for appropriate embryonic cardiac septation. Individuals with this autosomal dominant disorder have 1 or more limb anomalies, usually in the upper extremities. Approximately 75% of individuals with Holt-Oram syndrome have a clinically significant heart defect. The most common cardiac lesions are ventricular septal defect and atrial septal defect.
Up to 50% of individuals with Down syndrome have congential heart disease. The most common lesions are atrioventricular septal defect and ventricular septal defect. Congenital heart disease is also common with trisomies 13 and 18.
Approximately one-quarter of individuals with Turner syndrome have a congenital heart defect. The typical lesions are bicuspid aortic valve and coarctation of the aorta. Partial anomalous pulmonary venous drainage and ventricular septal defects are also relatively common in these patients.
The most common cause of pulmonary vascular prominence in an acyanotic child is a congenital left-to-right shunt. Left-to-right shunts are present in at least half of children with congenital heart disease. A left-to-right shunt can occur at the level of the great veins (e.g., partial anomalous pulmonary venous connection), between the atria, between the ventricles, or between the great vessels (e.g., patent ductus arteriosus) (Table 11-6). Unless associated with other substantial cardiac malformations, most left-to-right shunts are asymptomatic in infants; many patients do not present until later in childhood, when a murmur is detected.
Left atrium to right atrium or pulmonary vein to right atrium | Atrial septal defect |
Partial anomalous pulmonary venous connection | |
Aorta to pulmonary artery | Patent ductus arteriosus |
Hemitruncus arteriosus | |
Aortopulmonary fenestration (window) | |
Coronary-pulmonary fistula | |
Anomalous origin of left coronary artery from pulmonary artery | |
Aorta to right ventricle | Coronary arteriovenous fistula |
Sinus of Valsalva fistula | |
Aorta to right atrium or systemic vein | Coronary arteriovenous fistula |
Sinus of Valsalva fistula | |
Systemic arteriovenous fistula | |
Left ventricle to right ventricle | Ventricular septal defect |
Endocardial cushion defect | |
Left ventricle to right atrium | Endocardial cushion defect |
Isolated ventricular septal defect (VSD) is the most common congenital heart abnormality, accounting for approximately 20% of all congenital heart disease. Five percent of children with VSD have multiple defects, usually involving the muscular ventricular septum. VSD is a component of, or is frequently associated with, a variety of more complex anomalies, such as tetralogy of Fallot, truncus arteriosus, aortic coarctation, and tricuspid atresia. Approximately 5% of VSDs occur in children with chromosomal syndromes, such as trisomy 13, trisomy 18, and trisomy 21. The prevalence of VSD is approximately 2 per 1000 livebirths. The prevalence is higher in premature infants and is slightly higher in females.17
There are 4 types of VSD: perimembranous, supracristal (outlet septum), atrioventricular canal (inlet septum), and muscular (trabecular). Approximately 80% of VSDs are located inferior to the crista supraventricularis; these represent the perimembranous type. Most of these involve the true membranous septum and a small portion of the adjacent muscular septum between the right and posterior aortic valve cusps. This defect is located in the outflow tract of the left ventricle, immediately below the aortic valve. From the perspective of the right heart, the defect is beneath the crista supraventricularis and posterior to the papillary muscle. A subtype of perimembranous VSD is located between the papillary muscle of the conus and the crista supraventricularis.
The supracristal type accounts for approximately 5% of VSDs. This defect is located inferior to the pulmonary valve. The upper border of the defect is beneath the right coronary cusp of the aortic valve. Other terms for this type of VSD are conal defect and outlet defect. A supracristal VSD is apparently the result of incomplete and abnormal fusion of conal buds.
The atrioventricular canal type of VSD involves the inlet portion of the ventricular septum. This type is a component of the atrioventricular cushion defects that occur in association with trisomy 21 syndrome. The canal defect accounts for approximately 5% of VSDs. This defect involves the posterior ventricular septum immediately anterior to the anterior leaflet of the mitral valve and beneath the tricuspid annulus.
As the name implies, a muscular VSD occurs within the muscular ventricular septum. Approximately 10% of VSDs are of this type. The myocardium entirely borders this defect. Muscular VSDs are most often low and anterior in the septum. They can be further classified into central, posterior, and marginal types. Muscular VSDs can be single or multiple.18
The hemodynamic manifestations of a VSD predominately relate to the size of the communication and the status of the pulmonary vasculature. The pulmonary vascular resistance is relatively high in the normal newborn. Rapid decrease occurs in the immediate neonatal period because of a rise in the oxygen tension within the pulmonary arterial bed and expansion of the lungs. However, subsequent additional decline in pulmonary vascular resistance occurs over several weeks. A fully mature pulmonary vascular bed is not present until approximately 4 years of age. The characteristics at this stage include thin intima, relatively large lumen size, and diminished smooth muscle thickness.19
In some infants with a left-to-right shunt, maturation of the pulmonary vasculature proceeds in a normal fashion. In others, there is an initial period of involution in pulmonary arterial tone. However, left-to-right shunting at the ventricular level causes increased pulmonary blood flow and increased pressure, which, in turn, elicits muscular hypertrophy in arterial walls and subsequent thickening of the intima. These alterations can lead to pulmonary vascular hypertension. A third potential scenario in the infant with VSD is failure of fetal pulmonary vasculature involution and maturation, resulting in persistence of the fetal pattern of elevated pulmonary arterial pressure.
Anatomically, a VSD is considered to be “large” if it exceeds 75% of the diameter of the aortic root. A VSD of this size results in similar systolic pressures in the left and right ventricles, as well as within the aorta and pulmonary artery. The relative resistances of the pulmonary and systemic circuits in these infants determine the direction and magnitude of the shunt. The relatively high pulmonary resistance of the neonate serves to limit left-to-right shunting through the VSD. However, progressive diminution in pulmonary resistance over the next weeks to months leads to increased left-to-right shunting. The resultant increased pulmonary blood flow causes progressive enlargement of the left atrium and left ventricle. Eventually, ventricular decompensation can lead to congestive cardiac failure.
As noted above, pulmonary arteriolar disease can develop in the presence of a long-standing large VSD. There is progressive narrowing of the pulmonary arterial lumen size because of hypertrophy of the media and proliferation of the intima. The onset and progression of pulmonary arteriolar disease can occur during infancy or be delayed for years. The arterial narrowing eventually causes diminished pulmonary blood flow because of elevated right-heart pressure and diminished left-to-right shunting. Shunting ceases when the pulmonary vascular resistance approximates that of the systemic circuits. If pulmonary vascular resistance exceeds the systemic resistance, the shunt reverses; right-to-left shunting in this instance is termed Eisenmenger physiology.
An additional potential long-term consequence of a large VSD is infundibular stenosis of the right ventricular outflow tract. This occurs in approximately 3% of patients with a large VSD. This complication is most often associated with an anomalous right ventricular muscle group. A right aortic arch sometimes is present in these patients. The infundibular stenosis in affected infants can result in a clinical picture that is similar to that of tetralogy of Fallot.
With a “small” VSD (i.e., <75% of the diameter of the aortic root), the left ventricular systolic pressure is not freely transmitted to the right ventricle. In addition, the small size of the defect restricts the volume of blood that shunts into the pulmonary circuit. With these smaller defects, the volume of pulmonary blood flow is the major determinant of the pulmonary arterial pressure. With a small defect, the systolic pressure in the right ventricle is often normal, whereas larger defects lead to variable pressure elevation.
Infants and young children with a large VSD often have nonspecific clinical manifestations caused by the excessive pulmonary blood flow, such as irritability, easy fatigability, poor feeding and frequent respiratory infections. Infants who have not yet undergone elevation of pulmonary arterial pressure can have manifestations of cardiac failure. In older children with elevated pulmonary artery pressure, congestive cardiac failure is uncommon. Clinical manifestations in these older children can include growth retardation and recurrent respiratory infections. Infants typically have a loud systolic murmur, whereas the diminished flow through the defect in the older child with elevated pulmonary vascular resistance produces a softer murmur.
Patients with a small VSD are typically asymptomatic. The diagnosis is most often established by the incidental discovery of a loud pansystolic or midsystolic murmur. The murmur is usually not apparent until the second week of life or later, again relating to the dynamics of pulmonary vascular resistance and shunting across the defect. Occasional infants with a small VSD have mild tachypnea. Growth and development are usually normal. Small defects often close spontaneously, usually by the fourth year of life.
Aortic regurgitation is a potential complication of a VSD of any size; this occurs in approximately 5% of these patients. Aortic regurgitation is most strongly associated with the supracristal type of VSD. However, aortic regurgitation is most often identified patients with infracristal defects, as these are much more common than the supracristal variety.
As with the clinical features, the radiographic signs of VSD vary with the size of the defect and the status of the pulmonary vascular bed. Chest radiographs are frequently normal with a small VSD. There may be mild prominence of the central pulmonary vessels. In general, the left-to-right shunt must exceed 1.5:1 to result in detectable alterations on standard chest radiographs. Moderate-size shunts may result in mild cardiomegaly, prominence of the main pulmonary artery, central pulmonary vascular prominence (with an “active” pattern), and subtle left atrial enlargement. The radiographic differential diagnosis of this pattern includes patent ductus arteriosus, atrioventricular septal defect, aorticopulmonary window, and atrial septal defect.
A large VSD typically causes prominent cardiomegaly. This is a result of enlargement of the left ventricle and left atrium in response to an increased volume of pulmonary blood flow. Pulmonary vascular prominence includes central structures as well as peripheral pulmonary vessels (Figure 11-3). Some degree of pulmonary overaeration occurs in most patients. If there is superimposed congestive failure, the peripheral vessels have an indistinct appearance because of the presence of interstitial fluid (Figure 11-4).
Attention to the left atrium is important in the radiographic evaluation of the child with a suspected left-to-right shunt. Enlargement of the left atrium suggests shunting at the ventricular or great vessel level; left atrial enlargement is absent in most patients with an atrial septal defect. The size of the left atrium in a patient with a VSD also serves as an important indicator of the volume of pulmonary blood flow. If the left atrial enlargement is disproportionate to the pulmonary vascular prominence, the possibility of mitral valve disease should be considered.
Left atrial enlargement appears on lateral chest radiographs as a posterior bulge in the cardiac contour that elevates the left main bronchus. The frontal view shows prominence in the central aspect of the heart and elevation of the left main bronchus (Figure 11-5). Compression of the bronchus can lead to chronic or recurrent left-lower-lobe atelectasis. Enlargement of the left ventricle in patients with VSD causes downward displacement of the cardiac apex.
Figure 11–5
Ventricular septal defect.
An anteroposterior chest radiograph demonstrates mild cardiomegaly, pulmonary vascular prominence, and enlargement of the main pulmonary artery (arrow). Prominence of the left atrium (LA) results in soft-tissue density fullness in the mid-upper portion of the cardiac silhouette and slight elevation of the left main bronchus.

Progression to pulmonary hypertension is unusual in patients with a VSD, as most of these lesions are detected and treated prior to the onset of this complication. The key radiographic finding in patients with Eisenmenger physiology caused by a long-standing large VSD is marked prominence of central pulmonary vessels and rapid tapering to normal vessel size peripherally; that is, a pruned appearance (Figure 11-6). These patients also have little or no left atrial enlargement because of decrease in the magnitude of the left-to-right shunt. Decrease in volume overload also results in diminished heart size, with the heart sometimes returning to normal.
In those patients with aortic valve insufficiency in association with a VSD, the aortic arch is often prominent. (The size of the aorta is normal in patients with an uncomplicated VSD.) Patients with mild pulmonary stenosis in association with a VSD often have normal chest radiographs, as the outflow tract obstruction limits the size of the shunt. In patients with a VSD that involves the tricuspid valve, shunting occurs between the left ventricle and the right atrium; the radiographic features include left ventricular enlargement, dilation of both atria, and pulmonary vascular prominence.
Physiology | Radiology | |
---|---|---|
Small VSD | Left-to-right shunt <50% | Normal or mild pulmonary vascular prominence |
Large VSD, normal pulmonary resistance | Elevated volume of pulmonary flow | Cardiomegaly |
Left atrial enlargement | ||
Left ventricle enlargement | ||
Pulmonary vascular prominence | ||
Large VSD, elevated pulmonary resistance | Smaller volume shunt | Dilated tortuous pulmonary arteries |
Pulmonary vascular disease | Diminished cardiomegaly | |
Diminished left atrial size | ||
Eisenmenger physiology | Right-to-left shunt | Diminished heart size |
Pulmonary vascular disease | Pulmonary arterial pruning |
Echocardiography of patients with a VSD serves to characterize the anatomy of the lesion and to identify coexistent conditions. Echocardiography shows a perimembranous defect as an area of discontinuity adjacent to the septal tricuspid valve leaflet attachment. Color Doppler imaging demonstrates abnormal blood flow toward the right ventricular outflow tract. Doppler studies are also useful for detecting muscular defects. A small muscular VSD may not be detectable with standard echocardiography. In patients with a large left-to-right shunt, echocardiography shows the left atrial and left ventricular volumes to be increased. In the presence of pulmonary hypertension, the right atrium is enlarged and the wall is thickened.20
The most useful angiocardiographic projection for the demonstration of common VSDs is an angled left anterior oblique position. Optimally, the defect is visualized in profile. True anteroposterior and lateral projections often provide the best visualization of a supracristal defect. Injection of the left ventricle shows shunting of contrast-opacified blood into the right heart (Figure 11-7). In patients with pulmonary hypertension, contrast angiography should be avoided or performed with caution.21
MRI serves to supplement echocardiographic information in selected patients with VSD (Figure 11-8). Coronal and axial imaging planes are usually the most useful. Cine images show a flow jet at the site of the defect. The magnitude of left-to-right shunting can be estimated with phase-shift velocity mapping of flow within the pulmonary artery and aorta. Pulmonary hypertension is characterized with MR velocity mapping of the pulmonary artery as 2 peaks of forward systolic flow and extensive rapid retrograde flow during systole and diastole.22–25
Quantitative radionuclide angiocardiography is a noninvasive method to quantify left-to-right shunts. In normal individuals, an injected bolus of radiopharmaceutical sequentially passes through the superior vena cava, right atrium, right ventricle, lung, left atrium, left ventricle, and aorta. In the presence of a left-to-right shunt, there is abnormal persistence of activity in the lung and right heart because of early recirculation. Time-activity curves are generated from regions of interest created over the superior vena cava and the right lung. The curve from the superior vena cava serves to assess the integrity and compactness of the injected bolus. In normal individuals, the pulmonary time-activity curve increases and decreases sharply as the bolus passes through the pulmonary circulation. Subsequently, there is a second peak of lesser amplitude and greater duration, corresponding to the normal recirculation of the bolus from the systemic circulation. In the presence of a left-to-right shunt, early recirculation causes a broadening of the downslope of the first peak. Computer analysis allows estimation of the early recirculation activity and thereby the magnitude of the shunt.
The medical and surgical management of the child with VSD varies with the size and effects of the lesion. Management of small muscular VSDs is usually conservative; the defect often closes spontaneously. Larger defects are repaired primarily. Pulmonary artery banding is an optional temporizing measure for infants with a large defect. Outlet VSDs usually are repaired early in life to prevent development of aortic regurgitation. The standard corrective surgery for VSD is closure by a synthetic patch or, if the defect is small, direct suturing. Transcatheter deployment of a septal closure device is an option for selected patients, particularly those with muscular defects.
Following successful repair of a VSD, radiographs typically show rapid improvement in the pulmonary vascular pattern and normalization of heart size. In patients with increased pulmonary resistance, radiographic improvement occurs more gradually; a few or several years may pass until heart size normalizes. Persistent cardiomegaly after a VSD repair can occur as a consequence of incomplete closure of the defect, breakdown of a patch, or tricuspid insufficiency. MRI is sometimes useful to assess for abnormal flow across a patched VSD. Fibrous endothelialization of surgical patches results in low signal intensity on gradient echo images.26
Atrial septal defect (ASD) is a congenital opening in the septum between the right and left atria. This lesion results in a pretricuspid left-to-right shunt (Table 11-7). ASD accounts for approximately 10% of congenital heart disease. This is the most common form of congenital heart disease to present in adulthood. The male-to-female ratio is 2:3. ASD is a component of Holt-Oram syndrome and is sometimes associated with Ellis-van Creveld syndrome. The ostium primum type of ASD can occur in association with trisomy 21.
There are several types of ASD. Most common (>80%), is the ostium secundum defect. This is usually a large lesion and is located near the fossa ovalis. A margin of atrium separates the defect from the atrioventricular valves. Associated cardiac anomalies are present in less than 10% of patients with an ostium secundum defect. The most common associated conditions are partial anomalous pulmonary venous connection, persistent left superior vena cava, and prolapse of the mitral valve.
The ostium primum type of ASD is located in the inferior atrial septum caudal to the fossa ovalis. The pathophysiology involves failure of fusion of the septum primum to the septum intermedium. The ostium primum type accounts for approximately 10% of ASDs. The ostium primum defect is a component of the spectrum of abnormalities that occur with endocardial cushion defect; that is, atrioventricular septal defect. Cleft mitral valve and mitral insufficiency often occur in association with an ostium primum ASD.
A superior sinus venosus ASD is located above the fossa ovalis, adjacent to the entrance of the superior vena cava. There is deficiency in the sinus venosus septum that in normal individuals separates the sinus venosus portion of the right atrium from the right pulmonary veins and systemic veins; that is, deficiency of the common wall between the superior vena cava and the right upper pulmonary vein. This type accounts for 5% to 10% of ASDs. The superior vena cava may straddle the defect, such that systemic blood is directed into both atria. In approximately 90% of patients with a sinus venosus defect, there is anomalous pulmonary venous drainage. In addition, the proximity and orientation of the right pulmonary veins to the defect result in preferential flow of pulmonary venous return through the defect into the right atrium, while blood from the left pulmonary veins courses toward the left atrium.
Defects of the posterior inferior portion of the atrial septum are rare. This lesion is usually associated with absence of the wall between the coronary sinus and the left atrium; that is, coronary sinus septal defect. A persistent left superior vena cava empties into the left atrium. Defects can also occur at the junction of the inferior vena cava with the right atrium (inferior sinus venosus septal defect), as well as posterior to the fossa ovalis.
A patent foramen ovale is an additional potential cause of atrial-level shunting. Failure of appropriate closure of the foramen ovale in the neonate can occur as a consequence of an anatomic abnormality of the valve mechanism or abnormal atrial pressure relationships. In some instances, the valve is too short to completely cover the foramen ovale. In others, there is enlargement of the foramen ovale, a result of elevated left atrial pressure and volume, as occurs with patent ductus arteriosus, VSD, or left ventricular outflow obstruction. If the cause of the elevated left atrial pressure is relieved in these infants, shunting at the atrial level diminishes or disappears completely. With some congenital heart defects, patency of the foramen ovale after birth occurs because of elevated right-heart pressures. Right-to-left shunting through a patent foramen ovale is essential for survival prior to surgical repair with some types of congenital heart disease. Examples include tricuspid atresia, mitral atresia, total anomalous pulmonary venous connection, and some forms of transposition. In newborns who fail to undergo normal decrease in pulmonary vascular resistance after birth, pulmonary hypertension causes elevated right atrial pressure and persistent right-to-left shunting across an anatomically normal foramen ovale.
In normal infants, functional closure of the foramen ovale occurs soon after birth. There is anatomical closure of the septum primum and septum secundum by approximately 1 year of age. However, the foramen ovale is probe-patent in greater than 25% of normal adults. Minor left-to-right shunting through a patent foramen ovale is present in 10% to 15% of asymptomatic children and adults evaluated with Doppler or contrast echocardiography. Detection of a patent foramen ovale is also possible utilizing contrast-enhanced dynamic MRI. The small shunt associated with a patent foramen ovale is usually of no hemodynamic significance. There is, however, a weak association with migraine headaches, transient ischemic events, and stroke. The risk for a neurological event is greater in those individuals with a large shunt or an associated atrial septal aneurysm.27
Fetal cardiac hemodynamics are normal despite the presence of an ASD, as right-to-left shunting normally occurs across the patent foramen ovale. However, failure of appropriate closure of the atrial septal communication after birth in response to elevation of the left atrial pressure leads to a left-to-right shunt. The left atrium does not enlarge despite the prominent blood flow, as it is decompressed by the communication with the right atrium. In general, right ventricular hypertrophy does not occur in patients with an ASD, unless there is pulmonary vascular hypertension. With a large ASD, the pressures in both atrial chambers are equal; therefore, ventricular compliance is a major determinant of the magnitude of the shunt. Because the right ventricular wall is thinner than the left and the right ventricle is more distensible, more blood tends to empty into the right ventricle, thereby facilitating left-to-right shunting.
Infants with an isolated ASD are frequently asymptomatic. If cardiac failure occurs during infancy, it is usually precipitated by coexistent cardiac defects or another complication. In infants with pulmonary hypertension and an atrial septal communication, right-to-left shunting can result in clinically evident cyanosis. This is particularly common in preterm infants with congenital or acquired lung disease. Children with an isolated ASD occasionally exhibit manifestations of failure to thrive. Exercise intolerance may develop, but often not until adolescence. ASD is the most common congenital heart lesion to first clinically present during the adult years. In general, blood flow through an ASD does not produce a murmur. However, prominent flow across the right ventricular outflow tract and pulmonary valve in these patients often produces a long systolic ejection murmur. A loud first heart sound precedes the murmur. The second heart sound is widely split and fixed.
As with other left-to-right shunts, the shunt caused by an ASD must be at least 1.5:1 in order to produce radiographically detectable alterations in the pulmonary vascular pattern. Most patients with a small secundum defect have normal chest radiographs. With a moderate or large shunt, both the central and peripheral pulmonary vessels are prominent. In some patients, the vessels are ill defined because of interstitial fluid. In those patients with cardiomegaly, chamber enlargement consists of the right atrium and right ventricle (Figure 11-9). This pattern occurs with all forms of pretricuspid left-to-right shunts. The left atrium does not dilate in patients with an ASD. This is an important differentiating feature from other left-to-right shunts, such as VSD or patent ductus arteriosus.
Right ventricular enlargement in patients with an ASD causes uplifting of the cardiac apex that is often visible on frontal radiographs. Clockwise rotation of the heart causes the right ventricle to form the left cardiac border. The left upper heart border is straight or convex because of prominence of the right ventricular outflow tract. The main pulmonary artery is prominent and the aortic arch is normal in size. On lateral radiographs, right ventricular prominence fills the inferior retrosternal space (Figure 11-10). Enlargement of the right ventricular outflow tract is best visualized on the right anterior oblique view. A lateral esophagram view sometimes shows indentation or displacement of the esophagus by the left atrium; this is a result of displacement of the normal size left atrium by the enlarged right atrium. In patients with a sinus venosus defect, an abnormal horizontal orientation of the right upper pulmonary vein is occasionally visible on frontal radiographs.
Figure 11–10
Atrial septal defect.
A. An anteroposterior radiograph of a 2-year-old child with a systolic ejection murmur shows cardiomegaly and pulmonary vascular prominence. The main pulmonary artery segment of the left cardiomediastinal border is prominent. The aortic knob is normal. B. Pulmonary vascular prominence is also evident on the lateral view. Right ventricular enlargement causes fullness of the anterior aspect of the heart.

Physiology | Radiology |
---|---|
Left-to-right shunt | Active pulmonary vascular prominence |
Prominent main pulmonary artery | |
Increased volume and pressure in right atrium, right ventricle | Enlargement of right heart |
Left atrium decompressed into right atrium | Normal-size left atrium |
The atrial septum is visualized with echocardiography on the subcostal 4-chamber, apical, and subcostal short axis views. Most ASDs are easily demonstrated with echocardiography, although accurate assessment of the size is sometimes difficult. In the presence of an ASD, there is usually enlargement of the right atrium, while the left atrial size is normal. Echocardiographic manifestations of right ventricular volume overload include an increased diastolic dimension of the ventricular chamber and abnormal anterior motion of the interventricular septum during systole. Color Doppler evaluation shows left-to-right blood flow at the atrial level. Transesophageal echocardiography can provide the detailed measurements required for treatment with a transcatheter septal occluder.28
Echocardiography is the primary imaging technique for children with an ASD. For selected patients, MRI provides supplemental information concerning the morphology of the defect and the magnitude of the shunt. MRI is an alternative to transesophageal echocardiography for obtaining the detailed measurements of ASD that are required in planning for the placement of a transcatheter occlusion device.
With MR, axial, long axis, and short axis views are all helpful for demonstrating an ASD and for evaluating the right ventricle (Figure 11-11). In evaluating the atria with MRI, it is important to recognize that lack of visualization of the atrial septum in the region of the fossa ovalis is normal. The normal fossa ovalis has gradually tapered borders. An ASD can be differentiated from a normal fossa ovalis by the abrupt termination at the septum in the presence of a true defect. Dynamic imaging with a gradient-echo technique allows documentation of shunt flow. The spatial orientation, size, and shape of an ASD change during the cardiac cycle. Phase-shift velocity mapping of flow within the pulmonary artery and aorta provides information regarding the magnitude of the left-to-right shunt. On dynamic contrast-enhanced imaging, right-to-left shunting at the atrial level results in the appearance of contrast in the left atrium prior to the pulmonary veins. Imaging during the Valsalva maneuver accentuates shunting. MRI is particularly useful for demonstrating the pathological anatomy in patients with a sinus venosus defect and partial anomalous pulmonary venous connection (Figure 11-12).22,25,27,29–32
Figure 11–11
Secundum atrial septal defect.
An axial T1-weighted image of an 11-month-old child shows a large atrial septal defect (large arrow). There is a small residual segment of the septum primum (small arrow). The right atrium (RA) is markedly dilated. The right (RV) and left ventricular (LV) chambers are also prominent. (LA, left atrium.)

Figure 11–12
Sinus venosus defect and partial anomalous pulmonary venous connection.
A, B. Axial and sagittal ECG-triggered study-state free precession cine MR images of a 17-year-old patient with exertional chest pain and a heart murmur show a wide defect (arrows) in the tissue that separates the posterior wall of the superior vena cava and the anterior wall of the right upper pulmonary vein at its insertion with the left atrium. C. Anomalous drainage of the right upper pulmonary vein (arrow) to the superior vena cava is visible on this oblique coronal image. LA, left atrium; RA, right atrium; RPA, right pulmonary artery; RPV, right upper pulmonary vein; SVC, superior vena cava.

The therapeutic approach to ASDs is tailored according to the size of the defect, the clinical manifestations, and the presence or absence of associated lesions. Generally, repair during childhood is appropriate for an ASD that causes shunting of at least 50%, thereby preventing the onset of pulmonary vascular disease. Treatment is usually not required for an asymptomatic ASD in an infant, as up to half of these defects close spontaneously. The surgical technique involves either direct suture closure of the defect or the placement of a patch composed of pericardium or synthetic material. Some ASDs are amenable to treatment with transcatheter closure devices (Figure 11-13).
Endocardial cushion defect encompasses a spectrum of cardiac anomalies that result from maldevelopment of the embryonic endocardial cushion structures. Synonyms for endocardial cushion defect include atrioventricular septal defect and atrioventricular canal defect. Most of these patients have a defect that involves portions of both the atrial and the ventricular septa, as well as various abnormalities of the mitral and tricuspid valves. Endocardial cushion defect accounts for 4% to 5% of congenital heart lesions. The gender incidence is equal. Endocardial cushion defect is the most common cardiac anomaly in patients with trisomy 21.
The endocardial cushions are 2 masses of tissue, one dorsal and the other ventral, that grow into the embryonic atrioventricular canal and fuse to form the atrioventricular septum. Development of the atrial septum divides the embryonic single atrium into 2 chambers. The primitive single ventricle separates into 2 parts by way of fusion of the membranous portion of the ventricular septum, the endocardial cushions, and the bulbus cordis. The muscular portion of the ventricular septum grows cephalad to meet with the 2 ridges of the bulbus cordis. The endocardial cushions develop concomitantly and eventually fuse with the bulbar ridges and muscular portion of the septum. The final stage of ventricular separation occurs by growth of the fibrous tissue of the membranous portion of the interventricular septum.
Maldevelopment of the endocardial cushions results in a spectrum of malformations (Table 11-8). Structures that derive from the endocardial cushions include the lower atrial septum (the septum primum), the upper ventricular septum (membranous septum), the anterior leaflet of the mitral valve, and a septal leaflet of the tricuspid valve. Failure of normal growth of the endocardial cushions results in a low intraatrial communication inferior to the free margin of the septum primum. In most patients, there is also a defect in the uppermost portion of the ventricular septum, thereby creating an atrioventricular canal. If there is complete lack of endocardial cushion fusion, a common atrioventricular valve orifice results, with a continuous common leaflet that passes through the septal defect from one ventricle to the other. At the other end of the spectrum, partially deficient fusion produces a cleft in the anterior leaflet of the mitral valve.
Partial atrioventricular canal | Ostium primum defect |
Ostium primum defect, cleft mitral valve | |
Common atrium, atrioventricular valve deformity | |
Anterior mitral valve leaflet cleft | |
Anterior tricuspid valve leaflet cleft | |
Atrioventricular canal VSD | |
Complete atrioventricular canal |
The most common type of endocardial cushion defect is complete atrioventricular canal. There is a single contiguous defect in the lower portion of the atrial septum and the adjacent portion of the upper ventricular septum. The atrioventricular valve development is always abnormal in these patients. There are bridging leaflets that pass through the septal defect because of fusion of portions of the septal leaflets of the developing mitral and tricuspid valves. The single atrioventricular valve of complete atrioventricular canal is composed of 5 leaflets, 2 of which are entirely contained in the right ventricle, 1 of which is entirely contained in the left ventricle, and the other 2 are bridging.
With partial atrioventricular canal, there are 2 atrioventricular valves and 2 valve rings. The anterior mitral leaflet is cleft and attaches to the crest of the incompletely developed ventricular septum. The most common form of incomplete atrioventricular canal is termed ostium primum defect; in these patients, the anterior leaflet of the mitral valve is divided equally. The intermediate type of endocardial cushion defect is characterized by the presence of a limb of atrioventricular valve tissue that bridges common anterior and posterior leaflets.
In all patients with endocardial cushion defect, the left ventricular outflow tract is narrowed and elongated. There is concavity of the interventricular septum below the mitral valve, producing a shortened left ventricular inflow tract. These features result in an angiographic appearance likened to that of a goose neck. This morphology is a result of the attachment of the anterior mitral valve leaflet to the crest of the incompletely developed ventricular septum. Other features shared by all forms of endocardial cushion defect include normal development of the inferolateral leaflets of the mitral and tricuspid valves and inferior displacement of the septal portions of the atrioventricular valve annulus.33
There are 5 potential hemodynamic alterations of an endocardial cushion defect: left-to-right atrial shunt; left-to-right ventricular shunt; left-to-right shunt from the left ventricle to the right atrium; mitral regurgitation; and pulmonary hypertension. In patients with partial atrioventricular canal, the hemodynamics are similar to those of ASD with mitral regurgitation. If the ASD is large, the elevated pressure related to mitral valve incompetence is mainly transmitted to the right side of the heart. If the ASD is small, the left atrium and left ventricle enlarge approximately in proportion to the severity of the mitral regurgitation. With complete atrioventricular canal, all 4 cardiac chambers communicate. Because the right ventricle is more compliant than the left, prominent left-to-right shunting causes volume overload of the right atrium, right ventricle, and pulmonary circulation.34
The clinical manifestations of endocardial cushion defect vary with the specific anatomy and the presence or absence of associated lesions. In those patients with an isolated ostium primum defect and only minimal insufficiency of the mitral valve, the clinical course is similar to that of an ostium secundum ASD: few or no symptoms during childhood and progression to heart failure and arrhythmias during adult life. When there is substantial mitral valve insufficiency in association with an ostium primum defect, the onset of symptomatic congestive heart failure is earlier in life.
At the other end of the clinical spectrum of endocardial cushion defect are patients with complete atrioventricular canal (complete atrioventricular septal defect). This is usually accompanied by the onset of severe heart failure early during infancy. These children have manifestations of failure to thrive and frequently suffer recurrent respiratory infections. If the lesion is not corrected, pulmonary hypertension develops during childhood. Approximately 70% of infants with complete atrioventricular canal have trisomy 21.35
In keeping with the spectrum of hemodynamic consequences of endocardial cushion defect, there is variability in the radiographic findings. An ostium primum defect with little or no mitral regurgitation results in cardiomegaly caused by a large volume left-to-right shunt. Enlargement involves the right ventricle, right atrium, main pulmonary artery, and central pulmonary arteries; the size of the left atrium is normal.
An ostium primum defect that is accompanied by substantial mitral regurgitation also leads to right ventricular enlargement and pulmonary vascular prominence because of a large volume left-to-right shunt. However, mitral valve dysfunction causes increased strain on the left ventricle, and this chamber enlarges as well. The left atrium may also be prominent. In addition to pulmonary vascular prominence related to increased volume from the left-to-right shunt, left-heart failure often results in pulmonary venous distention and indistinct vascular margins.
Complete atrioventricular canal is associated with substantial cardiomegaly. There is disproportionate enlargement of the right atrium. The main pulmonary artery segment is prominent and the aorta is small (Figures 11-14 and 11-15).
Pathology | Radiology |
---|---|
Left-to-right shunt | Right ventricular enlargement |
Right atrial enlargement | |
Pulmonary vascular prominence | |
± Mitral regurgitation | Left ventricular enlargement |
Left atrial enlargement | |
Pulmonary venous distention | |
Complete atrioventricular canal | Marked right-heart enlargement |
Figure 11–15
Complete atrioventricular canal.
A, B. Anteroposterior and lateral chest radiographs of a 4-month-old child with trisomy 21 demonstrate cardiomegaly, pulmonary vascular prominence, enlargement of the main pulmonary artery, and an inconspicuous aortic arch. Right-heart border prominence on the frontal view is predominately a result of enlargement of the right atrium.

In patients with an ostium primum ASD, echocardiography shows the lower portion of the atrial septum to be absent. The apical and subcostal views usually provide optimal visualization of the cleft anterior mitral valve leaflet. The right atrium and right ventricle are enlarged. Paradoxic motion of the interventricular septum indicates the presence of right ventricular volume overload. Echocardiography of the complete form of atrioventricular canal shows a large central defect that involves the lower portion of the atrial septum and the upper portion of the ventricular septum. There is lack of complete separation of the mitral and tricuspid valves, and a bridging leaflet spans the defect. Images in the plane of the aortic root show the classic gooseneck deformity of the left ventricular outflow tract.
With angiocardiography, the gooseneck deformity of the left ventricular outflow tract in patients with endocardial cushion defect is visible on the frontal view (Figure 11-16). The best projection for visualization of the anomalous valve apparatus is the hepatoclavicular view. This projection shows the atrial septum in profile. Blood sampling from right-heart catheterization demonstrates abnormally high oxygen saturation because of shunting. Pulmonary artery systolic pressure is at or near systemic pressure in patients with complete atrioventricular canal.36
MR examination of patients with endocardial cushion defect shows absence of portions of the atrial and ventricular septa (Figure 11-17). Visualization of the cleft septal leaflet of the mitral valve that is a feature of incomplete atrioventricular canal often is not possible with MR. With complete atrioventricular canal, there is a common atrioventricular valve orifice, with contiguous valve leaflets. MR shows secondary signs of volume overload in the right cardiac chambers in patients with endocardial cushion defect. Coronal images may demonstrate the classic gooseneck deformity of the left ventricular outflow tract. Dynamic first-pass contrast agent signal-time studies show rapid recirculation and shunting. Volumetric and phase contrast cine methods allow quantification of shunting by comparing the pulmonary artery flow to the aortic flow.37,38
Persistent ductus arteriosus is the second most common congenital heart defect in full-term infants, after VSD. In most patients, a patent ductus arteriosus causes left-to-right shunting. This lesion often occurs as an isolated anomaly. The most common associated cardiac lesion is a restrictive VSD. With some cardiac anomalies, a patent ductus arteriosus provides a pathway for lifesaving blood flow into the pulmonary arteries (e.g., pulmonary atresia with intact ventricular septum).
In the fetus, the ductus arteriosus permits right ventricular blood to bypass the nonaerated lungs. Approximately 60% of fetal right ventricular output is diverted through the ductus into the aorta. At the time of delivery, expansion of the lungs causes most of the pulmonary artery blood flow to be redirected into the low resistance pulmonary vascular system. A decrease in circulating prostaglandins and an increase in arterial oxygen tension stimulate smooth muscle contraction and closure of the ductus. During the first few hours of life, there is usually small volume right-to-left or bidirectional shunting through the ductus arteriosus. With progressive decrease in pulmonary vascular resistance, mild left-to-right shunting occurs. Functional closure of the ductus normally occurs by 12 to 24 hours of age. Structural obliteration occurs by 2 weeks in 35% of infants, by 3 months in 95% of infants, and by 1 year in 99% of infants. The fibrosed ductus arteriosus constitutes the ligamentum arteriosum.39,40
Patent ductus arteriosus accounts for 5% to 10% of congenital heart lesions. The male-to-female ratio is approximately 1:2. The prevalence is about 1 in 1600 term livebirths. Maternal rubella virus infection during the first trimester is a risk factor for patent ductus arteriosus. Patent ductus arteriosus is more common in preterm infants than in those born at term, with a prevalence of 20% to 30% in this group. The prevalence rises with earlier gestational age and lower birth weight; approximately 75% of infants of 28 to 30 weeks’ gestation have patent ductus arteriosus. Patent ductus arteriosus is much more common in preterm infants with respiratory distress syndrome than in those without.41,42
Patent ductus arteriosus in the preterm infant can cause retrograde blood flow in the descending aorta during diastole, leading to diminished systemic flow and a predisposition to end-organ ischemia. Therefore, this lesion can contribute to morbidity from necrotizing enterocolitis, abnormal cerebral perfusion, respiratory distress syndrome, and chronic lung disease. In term infants and older children, a small patent ductus arteriosus is sometimes hemodynamically insignificant and associated with no symptoms. A large patent ductus with no other congenital heart lesions causes a left-to-right shunt. If left ventricular volume overload ensues, there is progressive congestive heart failure. As with other large left-to-right shunts, an untreated hemodynamically significant patent ductus arteriosus can lead to the eventual development of pulmonary arteriolar hypertension and Eisenmenger physiology. Older patients with patent ductus arteriosus are at increased risk for infective endocarditis.
The clinical manifestations of a patent ductus arteriosus predominantly relate to the size of the ductus, the pulmonary vascular resistance, and the presence or absence of additional cardiovascular anomalies. The narrow lumen of a small ductus restricts the volume of left-to-right shunting, and the child may be asymptomatic. These lesions are most often discovered incidentally at the time of auscultation, typically as a continuous “machinery” murmur. A moderate-sized patent ductus arteriosus often is associated with progressive increase in left-to-right shunting during the first few months of life, as the pulmonary vascular resistance falls. Common clinical manifestations in these patients include failure to thrive, recurrent upper respiratory tract infections, and exertional fatigue. A large ductus usually causes left ventricular decompensation during the first few weeks of life, producing tachypnea, tachycardia, poor feeding, and hepatomegaly. Preterm infants with patent ductus arteriosus often exhibit manifestations of congestive cardiac failure, as the incompletely developed left ventricle is unable to manage the volume overload. Physical examination often demonstrates prominent peripheral pulses and an active precordium.
As with the clinical findings, the conspicuity of the radiographic features of patent ductus arteriosus is roughly proportional to the degree of left-to-right shunting and the size of the ductus. The chest radiograph of a child who has a small patent ductus arteriosus is usually normal. As with other left-to-right shunts, a shunt magnitude of at least 1.5:1 is generally required for radiographic detection. A moderate-size patent ductus results in slight prominence of the main pulmonary artery and the central pulmonary vessels, with a normal-size heart. Potential findings with a large patent ductus include dilation of the left atrium and left ventricle, prominent pulmonary vascular markings, and enlargement of the main pulmonary artery (Figure 11-18). Because the shunted blood also courses through the aortic arch, this structure eventually enlarges as well; when present, this finding helps in the differentiation of patent ductus arteriosus from an intracardiac left-to-right shunt (Figure 11-19). Likewise, dilation of the left atrium helps to differentiate this lesion from an ASD. In those patients with congestive cardiac failure, the pulmonary vascular markings are indistinct and there are fluffy parenchymal opacities due to pulmonary edema. Although the shunt does not directly involve the right ventricle, long-term increase in pulmonary artery pressure eventually causes right ventricular hypertrophy.
Physiology | Radiology |
---|---|
Left-to-right shunt | Active pulmonary vascular prominence |
Enlarged main pulmonary artery | |
Enlarged left ventricle and left atrium | |
Enlarged aortic arch | |
Left ventricle volume overload | Congestive failure |
Occasionally, a patent ductus arteriosus is radiographically visible as a small mass along the left upper mediastinum, adjacent to the aortic arch. A similar appearance can occur with a ductus diverticulum, which is a funnel-shaped widening of the aortic origin of a ductus that is occluded at the pulmonary end. Aneurysm formation is an additional rare ductus anomaly; aneurysms can rupture or become infected. Calcification of the ligamentum arteriosum is an occasional incidental finding on imaging studies of infants and children. Although this can persist into adulthood, the calcification most often disappears with age.
Echocardiography readily demonstrates a patent ductus arteriosus in most affected patients. Occasionally, the long and tortuous patent ductus of a premature infant is less conspicuous. Doppler studies demonstrate the direction of flow through the ductus. Ratios of the left atrial diameter to that of the aortic root provide an indication of the hemodynamic significance of left-to-right shunting in patients with patent ductus arteriosus. In normal infants, this ratio is between 0.9 and 1.0. A ratio of greater than 1.2:1 is generally considered clinically significant in the premature infant with a patent ductus. With more substantial left-to-right shunting at the ductus, this ratio is 1.4 or greater. Echocardiography occasionally demonstrates minimal flow through a ductus arteriosus in a patient with no symptoms and no evidence of cardiovascular or pulmonary consequences; this is termed a silent ductus.43–45
Aortography is not required for the routine diagnostic evaluation of a patent ductus arteriosus. This study shows a patent ductus as a tubular structure that connects the anterior wall of the aorta to the proximal portion of the pulmonary artery, paralleling the course of the aortic arch (Figure 11-20). Often, the aortic end of the ductus is larger than the pulmonary end. An isolated patent ductus usually does not opacify with pulmonary angiography, as the shunt is entirely in the left-to-right direction. However, unopacified aortic blood streaming through the ductus causes dilution of contrast along the superior aspect of the opacified pulmonary artery. In patients with patent ductus arteriosus in combination with aortic arch obstruction, right-to-left shunting occurs through the ductus, and contrast from the pulmonary artery opacifies the aorta.
MR demonstrates a patent ductus arteriosus as a tubular structure coursing between the proximal aspect of the descending aorta (the aortic isthmus) and the distal portion of the main pulmonary artery or the proximal aspect of the left pulmonary artery. Most often, optimal viewing of the ductus is on axial or right anterior oblique images. Patent ductus arteriosus is sometimes difficult to visualize with MR because of the short length of the lesion, the turbulent blood flow, and the presence of large adjacent vessels. Turbulent flow within the adjacent portions of the pulmonary artery or aorta may provide a clue to the presence of a patent ductus. Secondary findings of a patent ductus arteriosus on MR include dilation of the left atrium and left ventricle, pulmonary vascular prominence, and enlargement of the aortic arch.46
In premature infants, indomethacin administration effectively facilitates closure of the ductus arteriosus; indomethacin is an inhibitor of prostaglandin synthetase.47,48 Indomethacin therapy is of limited usefulness, however, in full-term infants. Most patients with patent ductus arteriosus who are not candidates for, or fail, medical therapy undergo surgical ligation.49 Various transcatheter occlusion devices are also available (Figure 11-21). There is a small risk for a hemodynamically significant residual shunt after deployment of these devices.50
Partial anomalous pulmonary venous connection refers to the insertion of 1 or more pulmonary veins into the right atrium or a systemic vein. Hemodynamically, this is a left-to-right shunt, with fully oxygenated blood recirculated through the lung via the right heart. Patients with partial anomalous pulmonary venous connection are acyanotic. The prevalence of this anomaly is less than 1% of livebirths.
Partial anomalous pulmonary venous connection is frequently associated with a superior sinus venosus ASD or, less commonly, an ostium secundum defect. Approximately 10% of patients with ASD have coexistent partial anomalous pulmonary venous connection. Approximately 80% of partial anomalous venous connections involve veins from the right lung. Anomalous drainage from both lungs is rare.
Potential sites of anomalous pulmonary venous connection from the right lung include the superior vena cava (common), right atrium (common), inferior vena cava, coronary sinus, azygos vein (rare), ductus arteriosus (rare), and portal vein (rare). Connection of a right pulmonary vein to the inferior vena cava immediately below the diaphragm, in association with a hypoplastic ipsilateral lung and small right pulmonary artery, represents scimitar syndrome (hypogenetic lung syndrome). Connection of all of the right pulmonary veins to the right atrium in association with an ASD is common in children with polysplenia. Potential sites of anomalous connection of left pulmonary veins include a persistent left superior vena cava, the left brachiocephalic vein, the left subclavian vein, the coronary sinus, and the inferior vena cava (rare).51,52
Because only a portion of pulmonary venous return shunts into the right heart in patients with partial anomalous pulmonary venous connection, many individuals with this anomaly are asymptomatic. Those who are symptomatic usually have clinical manifestations that are identical to those of an ASD. In fact, many symptomatic patients have a coexistent ASD. The most common complaint is exercise intolerance. Symptoms often do not occur until adolescence or adulthood. Some patients have recurrent pneumonias. Failure of proper diagnosis and treatment of partial anomalous pulmonary venous connection can lead to congestive heart failure, atrial arrhythmias, and pulmonary vascular disease in adulthood. Cyanosis only occurs if there is progression to pulmonary artery hypertension. Auscultation demonstrates systolic murmurs because of increased blood flow across the tricuspid valve and right ventricular outflow tract.
The radiographic features of partial anomalous pulmonary venous connection vary with the size of the shunt and the presence or absence of associated anomalies. Occasionally, a somewhat prominent pulmonary vein is visible following an anomalous course. This finding is most common when an entire lung drains anomalously. If there is a high-volume shunt, radiographs may demonstrate pulmonary vascular prominence and mild to moderate cardiomegaly (enlargement of the right atrium and right ventricle).
In patients with scimitar syndrome, the anomalous pulmonary vein courses obliquely along the inferior aspect of the right lung toward the medial aspect of the diaphragm (Figure 11-22). Pulmonary hypoplasia results in rightward deviation of the heart and elevation of the right hemidiaphragm (Figure 11-23). The right pulmonary artery is small and the hypoplastic lung may appear oligemic. The pulmonary vessels in the contralateral lung are often slightly prominent. The shunt volume determines the heart size.53
Figure 11–22
Partial anomalous pulmonary venous connection (scimitar syndrome).
A frontal chest radiograph of an asymptomatic child with a heart murmur shows an anomalous right pulmonary vein (arrow) along the right-heart border. The vessel is wider inferiorly than superiorly, resulting in a resemblance to a scimitar sword.

Figure 11–23
Scimitar syndrome.
A. A posteroanterior chest radiograph of a 7-year-old boy with a history of fatigability shows a small right lung with an anomalous pulmonary vein (arrow) coursing along the inferior aspect. The pulmonary artery branches are small on the right, and slightly prominent on the left. B. The lateral view confirms the small volume of the right lung (arrow indicates anterior margin of lung). C, D. Coronal contrast-enhanced MR images show 2 anomalous right pulmonary veins (small arrows) draining into the inferior vena cava near its insertion into the right atrium. The right main pulmonary artery (large arrow) is small. The right lung hypoplasia results in elevation of the right hemidiaphragm and rightward extension of the upper mediastinal margin.

Standard echocardiography is sometimes equivocal for determining the number or sites of anomalous pulmonary veins in patients with partial anomalous pulmonary venous connection; consequently, cardioangiography is often used. Less-invasive techniques such as transesophageal echocardiography, helical CT, and cardiac MR can also be helpful (Figures 11-24 and 11-25). MR also allows quantification of the right-to-left shunting and differential lung perfusion. The major pulmonary veins are usually best visualized on axial and short axis views. The vessels between the anomalous connection and the right atrium are usually enlarged, for example, prominence of the superior vena cava as a result of anomalous pulmonary venous connection with the brachiocephalic vein.54,55
Figure 11–24
Partial anomalous pulmonary venous connection.
A. A chest radiograph of an 8-year-old child shows enlargement of the lower aspect of the superior vena cava (arrow). Additional findings include pulmonary vascular prominence bilaterally, enlargement of the main pulmonary artery, and slight right heart prominence. B. A cine cardiac MR image shows a dilated anomalous right pulmonary vein (arrow) that drains nearly the entire right lung. C. A more anterior image demonstrates dilation of the superior vena cava (arrow) beginning at the level of the anomalous connection.



Pathology | Radiology |
---|---|
Anomalous pulmonary vein | ± Abnormal vein course; prominent vein |
Left-to-right shunt | Mild cardiomegaly |
Mild pulmonary vascular prominence | |
Scimitar syndrome | Small right lung |
Sinus of Valsalva fistula is a left-to-right shunt that occurs because of rupture of a sinus of Valsalva aneurysm. A sinus of Valsalva aneurysm is a localized dilation of one of the aortic sinuses of Valsalva. The lesion can be congenital or acquired. Congenital sinus of Valsalva aneurysm accounts for less than 3% of congenital heart disease. These patients often have additional cardiac defects, such as VSD. Alteration in valve cusp function because of a sinus of Valsalva aneurysm can cause aortic insufficiency. At least 80% of sinus of Valsalva aneurysms involve the right coronary sinus. Most sinus of Valsalva aneurysms are asymptomatic. However, rupture into the right ventricle (75%) or right atrium is common late in childhood, resulting in a fistula and a symptomatic left-to-right shunt.56,57
Chest radiographs of patients with a sinus of Valsalva aneurysm are normal. After rupture of the lesion, nonspecific signs of a left-to-right shunt develop: cardiomegaly, prominent pulmonary vascularity, and congestive failure. Echocardiography demonstrates a left-to-right shunt and aortic valvular insufficiency.
Cardiac anomalies that result in pulmonary venous distention without cyanosis predominantly consistent of those that obstruct the flow of blood from the lungs into the left ventricle and lack a communication that provides a pathway for right-to-left shunting (Table 11-9). The obstruction can occur at the confluence of pulmonary veins, within the left atrium, or at the mitral valve. Obstructive lesions of the left ventricle or aortic arch can cause pulmonary venous congestion if there is progression to left ventricular failure (Table 11-10). Although usually acyanotic at the time of presentation, the lesions discussed in this section can cause cyanosis when there is superimposed pulmonary edema.
Site of obstruction | Lesion |
---|---|
Pulmonary veins | Congenital stenosis |
Postoperative stricture | |
Left atrium | Cor triatriatum |
Supravalvar ring | |
Atrial tumor (e.g., myxoma) | |
Mitral valve | Congenital atresia |
Congenital stenosis | |
Parachute mitral valve | |
Left ventricle | Hypoplastic left ventricle |
Diffuse subaortic stenosis | |
Focal subaortic stenosis | |
Aortic valve Aortic arch | Valvar aortic stenosis |
Supravalvar aortic stenosis | |
Coarctation of the arch | |
Interruption of the aortic arch |
Lesions that cause elevation of pulmonary venous pressure lead to redistribution of blood flow into the upper portions of the lungs, with enlargement of the upper lobe pulmonary veins. The pulmonary response to chronic elevation of pulmonary venous pressure predominantly consists of thickening of the intima and media of the pulmonary veins. Communications between pulmonary veins and the bronchial venous system progressively enlarge, sometimes precipitating hemoptysis. Elevation of pulmonary capillary pressure causes fluid, serum, and erythrocytes to move into the alveolar spaces; that is, pulmonary edema. Dilation of pulmonary lymphatic channels ensues. Pulmonary arterial pressure also increases and, eventually, right ventricular hypertrophy ensues.
Mitral valve disease in pediatric patients most often occurs as an acquired phenomenon, a result of conditions such as rheumatic heart disease, infective endocarditis, and cardiomyopathy. Congenital stenosis of the mitral valve occurs in 2 basic forms: hypoplasia and a developmental lesion termed parachute mitral valve, in which the cordae tendineae from both valve leaflets converge into a single bulky papillary muscle. With hypoplasia of the mitral valve, the margins of the valve leaflets are thickened and rolled, the cordae tendineae are short and thickened, the papillary muscles are underdeveloped, and the valve leaflets are fused.58
Congenital mitral stenosis often occurs in association with other anomalies, such as coarctation of aorta, valvar aortic stenosis, VSD, tetralogy of Fallot, and endocardial fibroelastosis. A form of parachute mitral valve is often associated with the Shone complex (coarctation of aorta, subaortic stenosis, supravalvar mitral ring, and parachute mitral valve). In those patients with hypoplasia of all components of the mitral valve apparatus including the annulus, the resultant outflow obstruction causes manifestations of hypoplastic left heart syndrome.59–61
The major hemodynamic effects of congenital mitral valve stenosis occur during diastole. With a substantial gradient, pressures are elevated in structures proximal to the mitral valve, including the left atrium and pulmonary veins. The severity of symptoms relate to the degree of obstruction and the presence of coexistent anomalies. With severe stenosis, diminished cardiac output causes manifestations of congestive heart failure. In other patients, the major clinical manifestation is exercise intolerance. Pulmonary venous congestion in infants and young children with congenital mitral stenosis results in a propensity for recurrent respiratory infections.
Elevation of left atrial pressure in patients with congenital mitral stenosis often produces radiographically demonstrable left atrial enlargement. The pulmonary vascular pattern relates to the severity of obstruction, ranging from redistribution of flow into the upper lobes to frank pulmonary edema. The left atrial enlargement is disproportionate to the degree of pulmonary vascular prominence. Overall cardiac size is normal or only minimally prominent, unless there is right ventricular decompensation.
Echocardiography of congenital mitral stenosis shows thickened mitral leaflets with poor diastolic motion. The left atrium is dilated. There may be diastolic fluttering of the mitral valve leaflets. With the classical form of parachute mitral valve, there is a single papillary muscle in the left ventricle into which all cordae tendineae insert. There are also transitional forms in which there are partially or completely fused papillary muscles.62
Angiocardiography of congenital mitral stenosis shows varying degrees of valve leaflet thickening, as well as shortening and thickening of the cordae tendineae. The left ventricle is relatively small. During the levo phase of pulmonary angiography, enlargement of the left atrium is visible.
As with other imaging techniques, MR shows a thickened, often crescent shaped, mitral valve. The left atrium is enlarged. There is thickening of the right ventricular myocardium. Central pulmonary vessels are prominent. The lungs often appear somewhat hyperintense on T2-weighted images because of prominent interstitial fluid.
Pathology | Radiology |
---|---|
Mitral obstruction | Enlarged left atrium |
Elevated pulmonary venous pressure | Pulmonary venous distention |
Right ventricular hypertrophy | Normal heart size |
MR, echocardiography: thick right ventricle wall |
Supravalvar stenosis of the left atrium is a rare anomaly that shares hemodynamic, clinical, and radiographic features with congenital mitral stenosis. This is a circumferential ring of connective tissue that is attached to the base of the atrial surfaces of the mitral valve leaflets. Most often, this lesion is nonobstructive and asymptomatic. In some patients, there is hemodynamically significant obstruction to blood flow from the left atrium into the left ventricle. This obstruction can result in symptomatic pulmonary venous congestion.63–65
Supravalvar stenosis of the left atrium is most often associated with other anomalies. This is a common component of Shone syndrome (subaortic stenosis, coarctation of aorta, parachute mitral valve, and supravalvar left atrial ring). Other reported associations include tetralogy of Fallot, VSD, and corrected transposition of the great vessels.
The radiographic findings of an obstructive supravalvar stenosing ring of the left atrium are identical to those of congenital mitral stenosis: pulmonary venous congestion and left atrial enlargement. The presence of a coexistent cardiovascular anomaly can substantially alter the radiographic pattern. Echocardiography is diagnostic.
Cor triatriatum, or triatrial heart, refers to division of 1 of the atria into 2 chambers. The most common form is cor triatriatum sinister, in which a membrane partially divides the left atrium into a posterior-superior chamber that receives the 4 pulmonary veins and an anterior-inferior chamber that empties into the left ventricle. Cor triatriatum sinister accounts for approximately 0.1% of congenital cardiac malformations. This lesion is caused by faulty incorporation of the embryonic pulmonary venous confluence into the left atrium. Each of the pulmonary veins connects into the pulmonary venous confluence, which persists as an accessory chamber. The accessory chamber communicates through one or more orifices with the left atrium (or, rarely, the right atrium). The anatomy of the connection with the atrium occurs in 3 forms: (a) a fibromuscular diaphragm partially divides the left atrium from the accessory chamber; (b) a constriction between the atrium and the chamber produces an hourglass deformity, or (c) a tubular channel connects the atrium and the accessory chamber.66
Most often, cor triatriatum sinister occurs as an isolated anomaly. Potential associated cardiovascular anomalies include partial anomalous venous connection, VSD, ASD, atrioventricular canal, and coarctation of the aorta. Uncommon associations with cor triatriatum include tetralogy of Fallot, Ebstein malformation, hypoplastic left heart syndrome, and asplenia.
The hemodynamic consequences and resultant clinical manifestations of cor triatriatum sinister primarily relate to the severity of obstruction at the level of the connection between the accessory chamber and the left atrium. Pulmonary venous pressure is elevated. Patients may suffer tachypnea, chronic cough, and recurrent pneumonia; the features often suggest pulmonary rather than cardiac pathology. Affected infants may be irritable and suffer failure to thrive. Episodes of pulmonary edema and congestive cardiac failure can occur.
The major radiographic feature of cor triatriatum sinister is that of pulmonary venous congestion. In infants, the imaging findings can mimic those of respiratory distress syndrome; however, air bronchograms are usually lacking and the onset of the radiographic abnormality is delayed for at least 24 hours. With mild obstruction, there is only minimal vascular prominence or pulmonary vascular redistribution. In contradistinction to mitral stenosis, the left atrium is smaller than normal. The enlarged accessory chamber may or may not cause radiographic alterations. Although right ventricular hypertrophy is a feature of cor triatriatum, this is usually not radiographically demonstrable. Heart size is typically normal except in those patients with decompensation. Rarely, there is a double density along the right cardiac border because of the prominent accessory chamber.
The major echocardiographic feature of cor triatriatum sinister is the presence of a membrane or narrowing between a posterior-superior chamber that receives the pulmonary veins and the adjacent left atrium. In some patients, the lesion appears as a membrane stretching across the left atrium. The membrane moves toward the mitral valve during diastole and away from the mitral valve with systole. The left atrial diaphragm of cor triatriatum is demonstrated on angiocardiography with either pulmonary artery or left atrial injections. The diaphragm is best visualized on frontal or right anterior oblique views. With pulmonary angiography, there is prolonged transit of contrast through the lungs and there is prolonged opacification of the accessory chamber.
With MRI, the anatomy of the connection between the accessory chamber of cor triatriatum sinister and the adjacent left atrium is often best visualized with axial images. The accessory chamber is posterior and superior to the true left atrium. The membrane dividing the 2 chambers bulges into the left atrial cavity. When there is severe obstruction, there may be high signal intensity in the proximal chamber on T1-weighted images. T2-weighted images show increased water content of the lungs because of interstitial edema.67
Pathology | Radiology |
---|---|
Persistent pulmonary venous confluence | Right-heart border double density (uncommon) |
Obstruction at left atrium communication | Passive pulmonary congestion Pulmonary edema |
Diaphragm between accessory chamber and left atrium | Left atrium diaphragm on echocardiography, angiocardiography, and MR |
Cor triatriatum dexter refers to partial membranous division of the right atrium into 2 chambers. This is less common than the left atrial form of cor triatriatum. The pathogenesis of cor triatriatum dexter likely involves persistence of the right valve of the sinus venosus during embryonic development. The clinical manifestations of cor triatriatum dexter vary with the degree of partitioning of the right atrium. Many patients are asymptomatic throughout life. A large membrane can cause right-sided heart failure and elevated central venous pressure. Associated symptomatic cardiac defects, such as ASD, are sometimes present. Echocardiography and cardiac MRI demonstrate the thin right atrial membrane. The lesion is usually best visualized on transverse images.68
There are multiple cardiovascular anomalies that cause cyanosis and radiographic evidence of active (arterial) pulmonary vascular prominence (Table 11-11). This pattern indicates the presence of a bidirectional shunt. A right-to-left shunt causes cyanosis, while a left-to-right shunt results in increased pulmonary arterial flow. Cardiac anomalies with bidirectional shunts are termed admixture lesions. The admixture of systemic and pulmonary venous returns can occur at any level of the heart or great vessels (i.e., the pulmonary veins, the atria, the ventricles, or the great vessels). With the exception of transposition of the great vessels, there is an inverse relationship between the volume of pulmonary blood flow and the degree of cyanosis in children with admixture lesions: greater volumes of pulmonary flow are associated with lesser degrees of cyanosis.
Cyanosis and active pulmonary vascular prominence |
---|
D-transposition of the great vessels |
L-transposition of the great vessels |
Persistent truncus arteriosus |
Double-outlet right ventricle |
Single ventricle, without pulmonary outflow obstruction |
Total anomalous pulmonary venous connection |
Double-outlet left ventricle |
Single atrium |
Tricuspid atresia with transposition of the great vessels |
Pulmonary arteriovenous fistula |
D-transposition of the great arteries is the most common cyanotic congenital heart anomaly to present during the first day of life. The prefix D indicates that the aorta is located to the right of the pulmonary artery. The great arteries are transposed, the atria are normal, the ventricles are normally related, and the atrioventricular connections are normal. Therefore, the ventriculoarterial connections are discordant and the atrioventricular connections are concordant. The aorta (including the coronary arteries) originates from the morphological right ventricle and the pulmonary artery arises from the morphological left ventricle. This anatomy results in parallel pulmonary and systemic circuits: systemic venous blood enters the right atrium, passes into the right ventricle, and is ejected into the aorta, while venous return from the lungs empties into the left atrium, passes into the left ventricle, and is ejected into the pulmonary artery. D-transposition is incompatible with life unless there is an associated communication between the 2 circulations. Potential communications include a patent foramen ovale, ASD, VSD, patent ductus arteriosus, systemic collateral arteries to the lung, or any combination of these pathways.
There are 3 major classifications of D-transposition: (a) D-transposition of the great arteries with an intact ventricular septum. This anomaly may or may not be accompanied by left ventricular outflow obstruction (i.e., subpulmonic stenosis). (b) D-transposition with a VSD and no left ventricular outflow tract obstruction. (c) D-transposition of the great arteries in association with a VSD and a variable degree of left ventricular outflow tract obstruction (complex transposition). Severe subpulmonic stenosis or pulmonic atresia in these infants results in relatively equal pressures in the ventricles.
D-transposition accounts for approximately 10% of congenital heart disease in children. Additional cardiac defects occur in somewhat less than half of these patients. The most common associated anomalies are VSD (often in combination with pulmonary stenosis) and lesions of the aortic arch. Other cardiac anomalies that sometimes occur in association with D-transposition include tricuspid atresia, atrioventricular canal, ASD, double-outlet right ventricle (Taussig-Bing anomaly), subvalvar aortic stenosis, pulmonary stenosis, pulmonary atresia, interrupted aortic arch, coarctation, and patent ductus arteriosus. A right aortic arch is present in only 4% of children with D-transposition and an intact ventricular septum. A right arch is present in 10% of those with a VSD in the absence of substantial pulmonic stenosis. There is a strong association (particularly in patients with dextrocardia) between single ventricle, D-transposition of the great vessels, and pulmonary stenosis or pulmonary atresia. Extracardiac congenital anomalies occur in only approximately 10% of children with D-transposition. There is an association with maternal diabetes.
In utero, the hemodynamics of the fetus with D-transposition of the great arteries are nearly normal, as blood returning from the systemic and pulmonary veins passes into the atria and ventricles in a normal manner. Blood from the right ventricle passes into the ascending aorta and subsequently into the systemic arteries and placenta. Blood from the left ventricle enters the pulmonary artery, but most diverts through the ductus arteriosus into the descending aorta because of high pulmonary resistance. There are 2 minor variations from normal fetal circulation: (a) poorly saturated blood from the superior vena cava is preferentially shunted to the cerebral circulation rather than through the ductus arteriosus, and (b) more highly saturated blood from the inferior vena cava is shunted to the lung.
The newborn with D-transposition of the great arteries is dependent on mixing between the pulmonary and systemic circulations for survival. For a brief period after birth, the ductus arteriosus and foramen ovale suffice for mixing. However, progressive decrease in pulmonary vascular resistance facilitates shunting of hypoxemic blood from the aorta to the pulmonary artery through the ductus arteriosus. There is obligatory shunting of pulmonary venous return from the left atrium to the right atrium. Therefore, there is a bidirectional shunt that mitigates severe cyanosis, with blood flowing from the aorta to the pulmonary artery and from the left atrium to the right atrium.
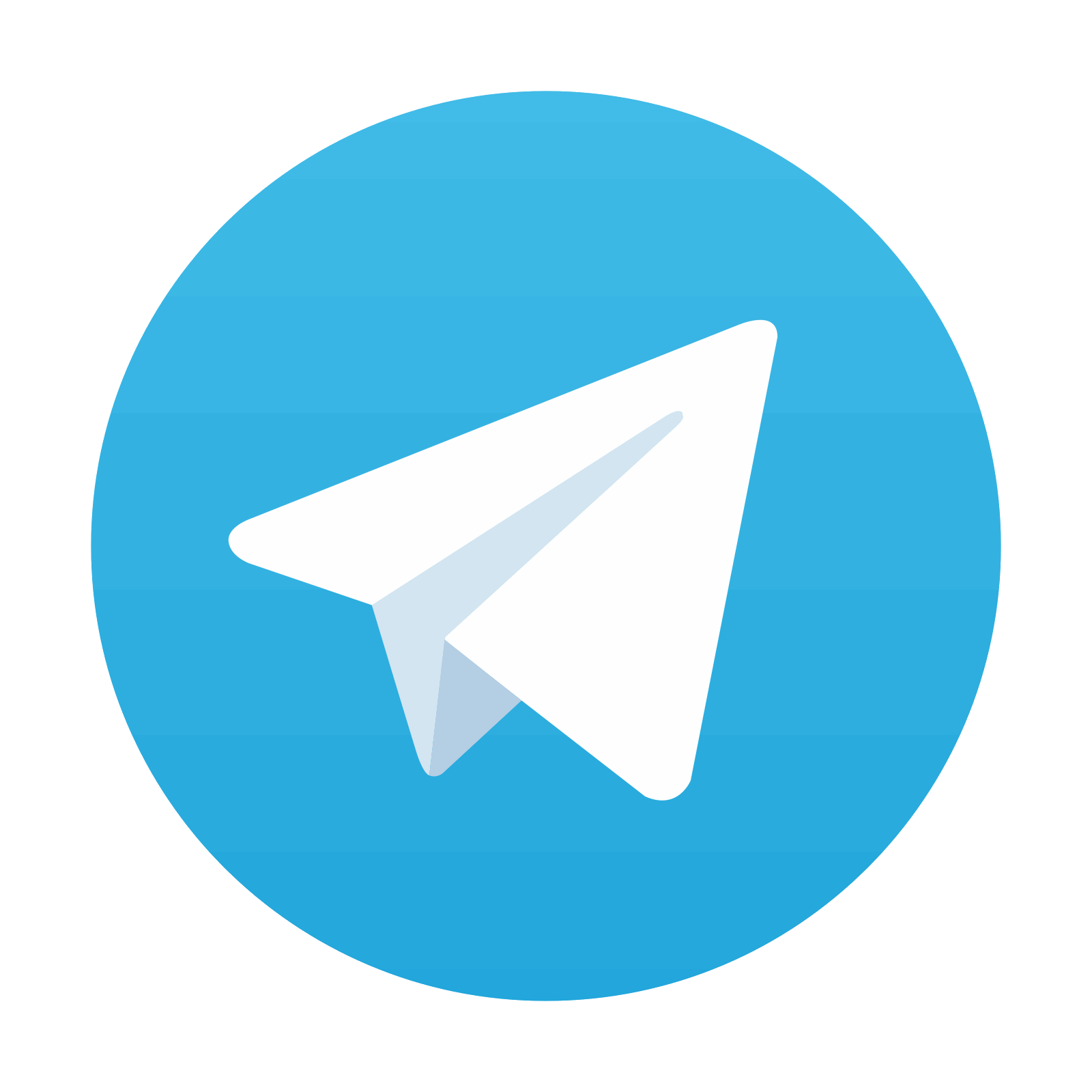
Stay updated, free articles. Join our Telegram channel

Full access? Get Clinical Tree
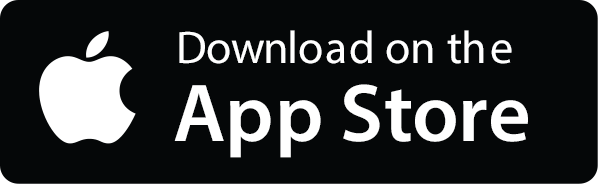
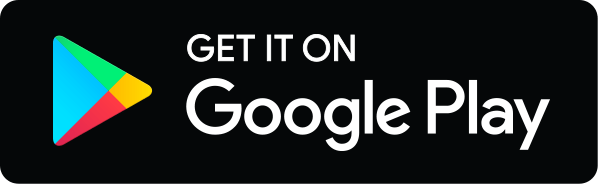