Computed Tomography/Magnetic Resonance Imaging
Srikanth Koneru
Milind Y. Desai
I. INTRODUCTION
Multimodality imaging has been increasingly used to advance our knowledge of causes and consequences of valvular heart disease. Echocardiography is now the standard modality for evaluation of patients with valvular heart disease because it is widely accessible, cost-effective, and portable. However, there are significant limitations in precisely assessing the morphology and severity of valvular dysfunction especially in patients with poor acoustic windows. In addition, the assessment of valvular disease with echocardiography is operator dependent. Over the past two decades, cardiac computed tomography (CT) and magnetic resonance imaging (MRI) are emerging as alternative imaging modalities by allowing excellent visualization of the morphologic features and function of a wide range of valvular diseases.
In this chapter, we emphasize the role of cardiac CT and MRI in assessing cardiac valves, along with their role in preprocedural assessment.
II. AORTIC STENOSIS
A. Morphology
1. The morphology of the aortic valve is clearly assessed by echocardiography; however, it may be challenging to discern if the image quality is suboptimal or there is technical difficulty secondary to patient body habitus. In these patients, cardiac CT and MRI are immensely helpful to precisely assess the aortic valve structure.
2. In using cardiac CT to assess the aortic valve, both systolic and diastolic phases have to be evaluated carefully as there could be a bicuspid aortic valve with a raphe, which could mimic normal tricuspid leaflets in the diastolic phase. The raphe of a bicuspid aortic valve is usually indicative of a fusion line between the two leaflets of the bicuspid aortic valve (e.g., fusion of the left to right coronary cusps). A typical fish-mouth appearance is clearly viewed during the systolic phase of data acquisition. Most commonly, the two cusps of a bicuspid aortic valve are unequal in size because of congenital fusion of the leaflets. Rarely, no raphe is identified, and this type of bicuspid aortic valve is termed true or pure bicuspid aortic valve. It is important clinically to diagnose bicuspid or unicuspid and, on rare occasions, quadricuspid (4 leaflets) aortic valve, because this would signal a further evaluation of the patient’s thoracic aorta to rule out associated thoracic aortic aneurysm, which could readily be evaluated within the same CT study.
3. Cardiac MRI has an advantage in evaluating young patients with suspected abnormal aortic valve morphology without the risk of radiation and also gives an excellent signal-to-noise ratio and better spatial resolution compared with transesophageal echocardiogram and transthoracic echocardiogram (TTE) for anatomic aortic valve assessment. Steady-state free precession (SSFP) acquisition with electrocardiography (ECG) gating is used to assess the aortic valve morphology. Once
again, cardiac MRI also has the advantage of enabling evaluation of the thoracic aorta for a possible aortopathy.
again, cardiac MRI also has the advantage of enabling evaluation of the thoracic aorta for a possible aortopathy.
B. Aortic valve calcification
1. Slow progression of calcification along with sclerotic thickening of the aortic valve reduces aortic valve excursion and impedes left ventricular (LV) outflow. CT provides high spatial resolution in assessment of aortic annular and leaflet calcification. Aortic valve calcium is defined as calcification within the valve leaflet or aortic annulus. In CT images, calcific deposits are displayed as bright regions within the image and are quantified by using Agatston units (AUs). The threshold for a calcific lesion was set at a CT density of 130 Hounsfield units having an area ≥1 mm2 with a density of 3 to 4 pixels. Area and volume of calcifications should be calculated per slice and summated. A recent study has shown that a calcium score <700 AU excludes severe aortic stenosis (AS) with a high negative predictive value and a score >2,000 AU suggests severe AS. However, according to the current guidelines, CT is not recommended for diagnosing AS, although calcification is the primary underlying pathology.
2. Measuring the aortic valve area planimetry by CT is also feasible and should be performed during midsystolic frames, ideally at 5% to 25% of the R-R interval to infer stenosis severity. Although CT is rarely used in clinical practice for this purpose, it has been shown to have a promising correlation with stenosis severity as assessed by TTE and is a useful guide in assessing the aortic valve when aortic valve calcification is seen on routine CT scans.
3. Cardiac MRI can be utilized in uncertain cases with inconclusive echocardiography results. The aortic valve planimetry can be performed from a series of sequential high-resolution SSFP or gradient echo cines acquired every 4 mm from a transverse plane. The smallest systolic opening during peak systole is planimetered. Low-dose dobutamine can be administered at the dose similar to echocardiography (up to 20 µg/kg/min) to differentiate pseudo-AS from true AS and also to assess contractile reserve. Cardiac MRI cannot quantify aortic valve calcification as there will be a signal void in the standard SSFP cine images.
C. Quantification of severity
1. Severity of AS can be assessed by echocardiography through measurement of transaortic valve flow velocity and insertion of the value into the continuity equation to obtain aortic valve area. The echocardiogram-based measure of aortic valve area is considered both reliable and accurate. However, it is increasingly common to underestimate transaortic flow velocity if the alignment of the transducer curser is not parallel to the flow, making it technically difficult to obtain the highest Doppler velocity.
2. Cardiac MRI has an advantage in this regard by allowing slice selection at any angle to measure an accurate flow velocity across the valve. Phase-contrast velocity mapping makes it possible to measure the flow of interest in an en face image of the aortic valve to determine the severity of the aortic valve stenosis by peak velocity. The phase-contrast imaging assesses the flow by calculating a shift of the precession between the stationary protons and protons moving in a magnetic field. The magnitude of this phase shift is proportional to the velocity of interest. The velocity must be sampled at 25 to 50 cm/s increments by setting the velocity encoding to reach the determined peak aortic valve velocity where there is no aliasing. A misalignment of more than 20 degrees between the phase direction and flow direction makes the velocities inaccurate. The functional and hemodynamic assessment of flow is not feasible and is still a research tool in the field of cardiac CT.
D. Assessing ventricular function
1. AS increases the afterload on the left ventricle, an effect that leads to compensatory LV hypertrophy. The chronicity of AS leads to ventricular dysfunction followed by irreversible LV injury, which is characterized histologically by fibrosis. Severity
and chronicity of AS can be assessed by measuring LV mass, volume, and ejection fraction. A reduced LV ejection fraction and increased LV mass have been associated with poor outcome in asymptomatic patients. The fast technical development of cardiac CT scanner hardware along with multisegmental image reconstruction has led to rapid improvement of spatial and temporal resolution and significantly faster cardiac scans. ECG-gated four-dimensional (4D) cine image reconstruction in systolic and diastolic phases can help assess LV functional parameters. The end-diastolic and end-systolic frames are used to calculate the LV volumes and ejection fraction, whereas an end-diastolic frame should be used to calculate LV mass. However, considering the accompanying contrast administration, radiation, and limited temporal resolution, referring a patient to cardiac CT only for evaluation of LV function is not warranted but adds important clinical information if the CT data are acquired for a different diagnostic utility (e.g., coronary artery disease, assessing aorta).
and chronicity of AS can be assessed by measuring LV mass, volume, and ejection fraction. A reduced LV ejection fraction and increased LV mass have been associated with poor outcome in asymptomatic patients. The fast technical development of cardiac CT scanner hardware along with multisegmental image reconstruction has led to rapid improvement of spatial and temporal resolution and significantly faster cardiac scans. ECG-gated four-dimensional (4D) cine image reconstruction in systolic and diastolic phases can help assess LV functional parameters. The end-diastolic and end-systolic frames are used to calculate the LV volumes and ejection fraction, whereas an end-diastolic frame should be used to calculate LV mass. However, considering the accompanying contrast administration, radiation, and limited temporal resolution, referring a patient to cardiac CT only for evaluation of LV function is not warranted but adds important clinical information if the CT data are acquired for a different diagnostic utility (e.g., coronary artery disease, assessing aorta).
2. Cardiac MRI is very attractive in assessing LV function because there is no radiation exposure, though the risk of gadolinium is only evident in patients with renal dysfunction. A complete set of short-axis sequential cine images is acquired from base to apex (every 8 mm) using SSFP pulse sequence. The advantage of cardiac MRI is (1) it can assess LV and right ventricular (RV) function by planimetry of LV and RV volumes in end-diastole and end-systole slices, in a three-dimensional fashion without the need for geometric assumptions, and (2) the volumes are calculated by summing all the slices, thus determining ventricular stroke volume, cardiac output, and ejection fraction with standard equations. Furthermore, planimetry of epicardial contours can be performed on all the slices to obtain a highly accurate ventricular mass measurement. Late gadolinium-delayed enhancement imaging in patients with AS has shown patchy mid-wall enhancement in patients with severe stenosis. This likely reflects focal areas of fibrosis, which have also been correlated in autopsy studies, and has been associated with worse prognosis. Also, diffuse myocardial fibrosis can be quantified using T1 mapping and other newer MRI techniques currently being investigated.
E. Procedural assessment
1. Calcific and degenerative AS is a dynamic and progressive disease, with risk factors similar to atherosclerosis. Currently, there are no options to slow the progression of this disease, and the only solution is aortic valve replacement for advanced disease stages. Surgical aortic valve replacement is the treatment of choice for the majority of patients with severe symptomatic AS suitable for surgery. However, there are a significant number of patients with high operative mortality secondary to advanced age and comorbidities, as estimated from a number of different scoring systems such as the Society of Thoracic Surgeons (STS) risk estimate or EuroSCORE. The poor outcomes with medical management in patients with high surgical risk have led to the development of less invasive transcatheter interventional procedures, including percutaneous balloon aortic valvuloplasty and transcatheter valve replacement.
2. For the transcatheter approach to AS, the cardiac CT evaluation and imaging acquisition protocols are unique and are integrated into the work-up of transcatheter aortic valve replacement (TAVR) patients. They typically include imaging the entire aorta, including the aortic root, thoracoabdominal aorta, and iliofemoral arteries, along with a high-resolution (4D) root acquisitions for better detail of the valve/root imaging. ECG gating is critical to avoid image degeneration secondary to motion artifact as this could affect the precise measurement of the aortic root and annulus. The ECG gating could be achieved by either prospective gating or retrospective gating. Prospective gating has an advantage of reduced radiation exposure; however, it limits image acquisition to specific phases of the cardiac cycle. Though retrospective gating has the disadvantage of higher radiation exposure, it
has the advantage of acquiring the entire cardiac cycle to subsequently reconstruct images to a specific phase of the cycle. In addition, retrospective gating allows 4D image reconstruction of multiple image planes to evaluate the aortic root and annulus at different cardiac phases (i.e., aortic annulus during systolic phase) and helps to evaluate LV and left valvular function.
has the advantage of acquiring the entire cardiac cycle to subsequently reconstruct images to a specific phase of the cycle. In addition, retrospective gating allows 4D image reconstruction of multiple image planes to evaluate the aortic root and annulus at different cardiac phases (i.e., aortic annulus during systolic phase) and helps to evaluate LV and left valvular function.
3. Radiation exposure is an important aspect to consider, though less concerning in the elderly patient population being evaluated for TAVR. Advanced scanner technology and imaging protocols allow a better image resolution with lower doses of radiation. Another critical aspect to consider is administration of contrast. Although contrast is necessary for precise measurement of luminal and annular diameters, the risk and benefit should be weighed before administering this in an elderly population with underlying renal dysfunction. A standard contrast dose of 80 to 120 mL is administered for a typical TAVR protocol. However, lower contrast-volume protocols are being evaluated; these use only 30 mL of contrast to visualize the aortic root with retrospective ECG gating, followed by a subsequent high-pitch data acquisition of the same contrast bolus. Alternatively, using an intra-arterial injection of contrast for iliofemoral CT angiography through a catheter left in place during coronary angiography may reduce the contrast required to <15 mL.
4. Annular measurements have been historically performed by using two-dimensional (2D) transthoracic or transesophageal echocardiography and aortic angiography, assuming the annulus is a circular orifice. From the procedural point of view, the annulus is defined as the plane at the lowest insertion point of the aortic valve leaflets, just above the LV outflow tract (LVOT). The acquired three-dimensional (3D) data from the CT scan are reconstructed along precisely defined 2D imaging planes perpendicular to the center axis, immediately below the hinge point of the aortic valve cusps. These double-oblique reconstructed planes reveal an ovoid to elliptical shape of the LVOT, from which the long- and short-axis diameters are measured, allowing for the calculation of the mean diameter. In addition, perimeter and area of the annulus can be obtained by planimetry, thus guiding the selection of correct prosthesis size and type (Fig. 17.1). Though currently there are no definite exclusion criteria based on the risk of coronary obstruction during TAVR, measuring the distance from the coronary ostia to the annulus helps in identifying patients at risk for coronary occlusion. The CoreValve (Medtronic, Minneapolis, MN) extends beyond the sinotubular junction into the aorta (in contrast to Edwards Lifesciences [Irvine, CA] valve), and hence measuring the ascending aorta should be part of the evaluation, as currently the maximal diameter of the proximal ascending aorta should not exceed 40, 42, and 43 mm for the three sizes of valves available.
Finally, assessing the iliofemoral arteries for access is a major component of TAVR evaluation as this is the major route of delivery for the most commonly used devices. Vascular complications have emerged as the major cause of mortality and morbidity associated with percutaneous femoral interventions using older large sheaths (#22 French to #24 French) compared with the currently available vascular sheaths (#18 French). Assessing calcification is particularly important. If arranged in a circumferential or horseshoe-like pattern, such calcification limits arterial expandability to accommodate large-profile delivery sheaths, likely increasing the risk of dissection or perforation. CT provides multiple measurements throughout the course of the iliofemoral system bilaterally, with the minimum luminal diameter measured on each side and specific areas with reduced luminal size or plaque burden also assessed (Fig. 17.2).
5. Newer delivery systems, along with integrating CT into the TAVR work-up, further reduce the vascular complications, and in the setting of unfavorable iliofemoral anatomy, a subclavian or transapical approach may be selected.
6. Though the standard of care involves cardiac CT for TAVR evaluation, rarely cardiac MRI can be used for this purpose because it carries no risk of radiation, provides
superior hemodynamic information, and may be performed without contrast in patients with renal insufficiency. SSFP MRI sequences including the short axis at the level of the aortic valve, along with a 3D whole-heart image covering up to the ascending aorta, allow for reconstruction of the aortic annulus in orthogonal views, to precisely measure the aortic annular dimensions.
superior hemodynamic information, and may be performed without contrast in patients with renal insufficiency. SSFP MRI sequences including the short axis at the level of the aortic valve, along with a 3D whole-heart image covering up to the ascending aorta, allow for reconstruction of the aortic annulus in orthogonal views, to precisely measure the aortic annular dimensions.
III. AORTIC REGURGITATION
A. Morphology
Bicuspid aortic valve and ectasia of the aortic root are the most common causes of aortic regurgitation (AR) in the developed countries, whereas rheumatic heart disease is more common in the developing countries. As mentioned earlier, the aorta and aortic valve structure are well visualized by both CT and MRI and can elucidate the mechanism of AR by demonstrating the valve cusp characteristics, and the status of aortic root and ascending aorta. Both imaging modalities demonstrate the regurgitant orifice in the case of moderate to severe AR. The area of the regurgitant orifice can be quantified with planimetry in the diastolic phase of the cardiac cycle and correlates with the severity of regurgitation estimated by echocardiography. An area of 0.28 cm2 has a sensitivity and specificity of 90% and 91%, respectively, in detecting severe AR (Fig. 17.3). In MRI, the presence of a regurgitant jet can also be visualized in the long-axis LVOT cine images either through SSFP or by gradient echo (Fig. 17.4). This flow turbulence produces a signal void on the MRI cine images because of dephasing of protons. Early studies have shown some correlation between the signal void and the echocardiographic method of grading AR. A narrow jet width at the origin suggests lower degrees of regurgitation, whereas a wide jet with a core of high signal void
suggests more severe regurgitation. However, the signal void is highly dependent on the type of MRI pulse sequence parameters and can be misleading if it is the only method used to quantitate AR.
suggests more severe regurgitation. However, the signal void is highly dependent on the type of MRI pulse sequence parameters and can be misleading if it is the only method used to quantitate AR.
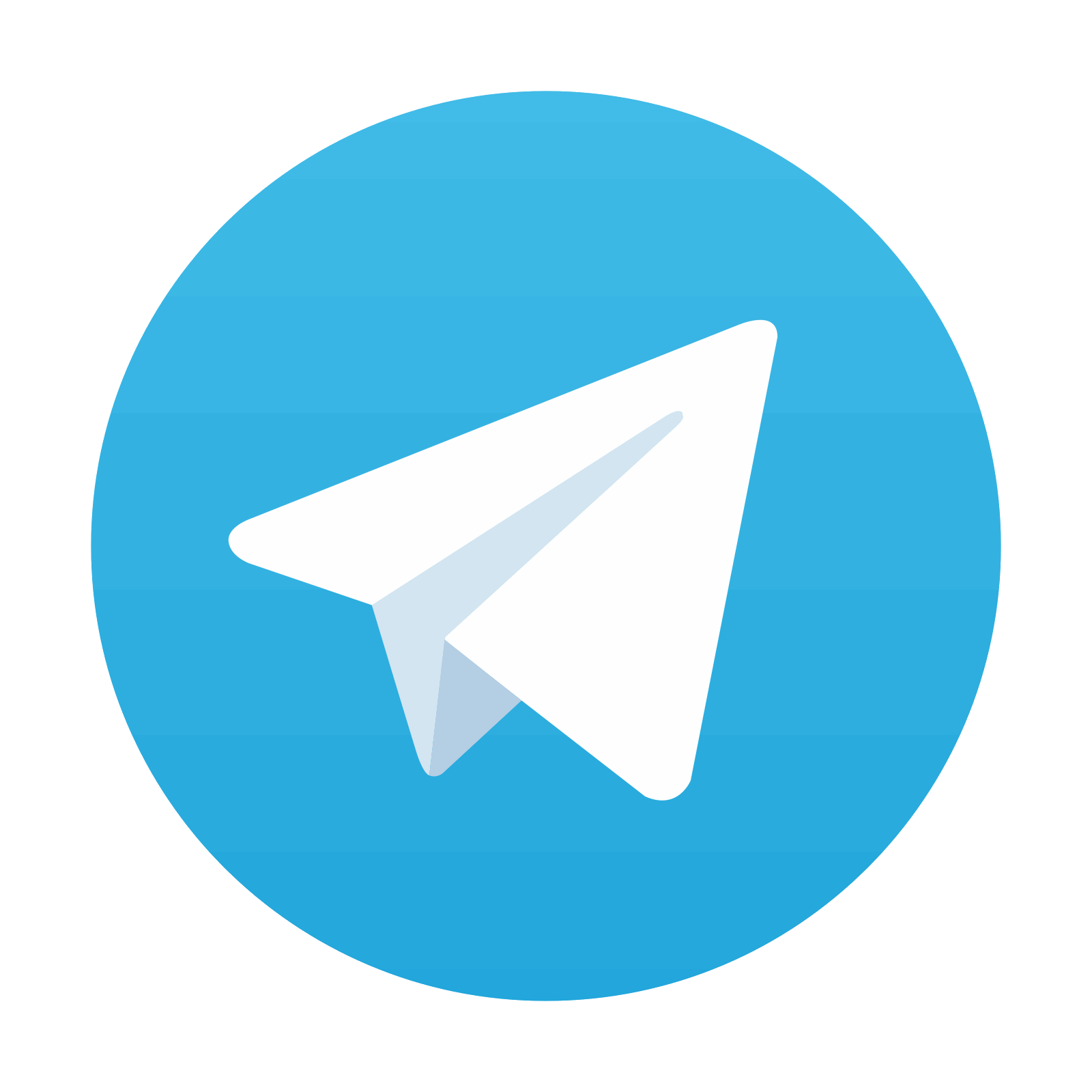
Stay updated, free articles. Join our Telegram channel

Full access? Get Clinical Tree
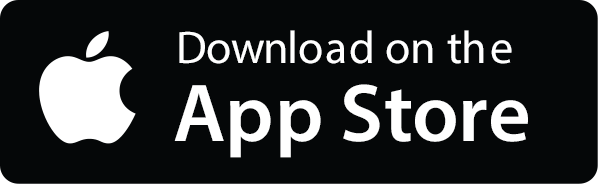
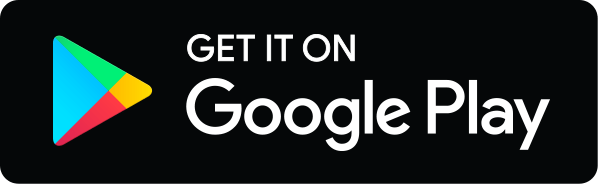