Computed tomography (CT) was developed in the early 1970s. The first computed axial tomography (CAT) scanners developed by Sir Geoffrey Hounsfield and colleagues required long acquisition and reconstruction times and were only suited for (small) stationary body parts like the head (Fig. 12-1). However, within the same decade, scanners dedicated to cardiac imaging were developed that were able to acquire images in 100 milliseconds or less, which was sufficient to virtually freeze cardiac motion. Contrary to mechanical CT scanners, these electron-beam CT systems lacked mechanically rotating parts. Instead of a rotating tube-detector unit, a beam of electrons was electromagnetically swept along a stationary tungsten target ring around the patient. As the electrons hit the tungsten ring, a roentgen fan beam was created. On the opposite side of the gantry, attenuated roentgen rays were collected by a stationary ring of roentgen detectors. For over 2 decades, these scanners were in operation, primarily to image coronary calcium,1 but also to image the coronary lumen after intravenous contrast injection.2 Technical and other practical challenges to electron-beam CT, such as difficulties expanding the number of simultaneously acquired slices and expanding the longitudinal scan range, stalled further exploitation.
In the 1990s, the introduction of slip-ring technology for cableless exchange of energy between the rotating and stationary scanner components of mechanical CT scanners allowed for faster, uninterrupted scanner rotation and the development of spiral CT imaging techniques.3 Spiral or helical scan protocols, through continuous table movement and data acquisition, allow for faster longitudinal coverage and propelled the possibilities for contrast-enhanced CT applications, including coronary imaging. Just before the turn of the millennium, the first 4-slice spiral CTs with electrocardiogram (ECG) synchronization for cardiac imaging were introduced.4,5 Over the next decade, the technical capabilities of cardiac CT improved rapidly, with faster rotating scanners and increasing numbers of detector rows for better and easier coronary imaging (Fig. 12-2). However, concerns about the increasing radiation burden associated with cardiac CT emerged, which motivated technical innovations to reduce the radiation dose while maintaining sufficient diagnostic accuracy.
In addition to noninvasive assessment of coronary stenosis, coronary CT also allows for assessment of the atherosclerotic changes in the vessel wall. Endeavors to noninvasive identify vulnerable plaques are ongoing. As the technologic accuracy of coronary CT angiography (CTA) matured and the radiation exposure reached acceptable levels, scientific attention shifted toward the clinical validation of the technique to define its most effective role in cardiovascular medicine. Several trials, both small and large, have been performed to assess the value of coronary CT (eg, in patients with stable angina or acute chest pain). Over the past decade, practicing physicians have become increasingly aware of the importance of functional stenosis assessment in the management of coronary artery disease. Although coronary CTA is an excellent means to exclude coronary disease, imaging of anatomy does not allow direct interpretation of the hemodynamic relevance. Several functional CT applications, such as coronary attenuation patterns, virtual fractional flow simulations, and stress myocardial perfusion imaging, are currently being investigated as options to complement CTA to provide a comprehensive assessment of patients and guide therapy.
CT is an imaging technique based on the roentgen attenuation characteristics of tissues. While the emitting roentgen tube and opposing detectors rotate around the patient, a large number of roentgen projection profiles are collected. From these projection profiles that represent the cumulative attenuation under varying angular degrees, the regional attenuation contribution, which depends on atomic composition and density, can be calculated using sophisticated reconstruction algorithms. The resulting image is the spatial distribution of the attenuation properties throughout a cross-section, relative to the attenuation properties of water, expressed in Hounsfield units (HU). By definition, the attenuation value of water is 0. Fat tissue has attenuation values just below 0, while in the relative absence of matter (air), attenuation values are very low (conventionally represented by dark shades of gray). Most (nonenhanced) soft tissues have positive attenuation values just above water, whereas tissues containing heavier atoms (eg, calcium, iodine, metals) have substantially higher attenuation values (bright shades).
Because the attenuation values of blood and the vessel wall are similar, the lumen cannot be imaged without the use of a contrast medium. Iodine-containing contrast media are usually injected through a peripheral vein. Arterial imaging is best during the first passage of contrast medium, and the required duration of the enhancement plateau depends on the scan duration. While with 4-slice scanners a cardiac scan took 30 to 40 seconds, current 64- to 320-detector technology requires only a few seconds, which allows for a smaller contrast bolus and more comfortable breath hold. Optimal timing of the scan relative to the arrival of contrast medium can be achieved by first performing a test bolus injection or by a bolus tracking technique, in which case the data acquisition is automatically started as soon as aortic enhancement is detected on a monitoring scan.
The main challenge for coronary imaging by CT lies in the continuous movement of the coronary arteries. The temporal resolution of CT is relatively modest, at least in comparison to some other cardiac imaging techniques, which increases vulnerability to artifacts caused by coronary displacement. The temporal resolution of cardiac CT is determined by the time necessary to acquire the minimal number of projections for image reconstruction. Partial scan reconstruction algorithms can reconstruct images based on an approximately 180° gantry rotation. Consequently, for single-source scanners with a rotation time of 250 to 350 milliseconds, the fundamental temporal resolution is around 125 to 175 milliseconds. The temporal resolution can be improved in several ways. By combining 2 tube-detector systems at an angular off-set of 90°, dual-source CT scanners collect all projections over a 90° rotation, improving the temporal resolution by a factor of 2 (down to 65 milliseconds). Alternatively, phase-consistent data of the same section that were acquired over 2 or more heart cycles can be combined for image reconstruction, which improves the effective temporal resolution, albeit in a less predictable manner and at the expense of a higher radiation dose. Finally, the effective temporal resolution can also be improved by slowing the heart rate using β-blockers or other medication.
Despite efforts to improve the temporal resolution, the maximum achievable temporal resolution of CT does not allow for motion-free imaging of the coronary arteries throughout the cardiac cycle. Coronary displacement is less during mid-diastole and at the end of systole. Because most scanners cannot cover the heart in a single beat, multiple stacks of data are acquired over a number of heart cycles. To assure reconstruction of images during the optimal motion-sparse phases within the heart cycle, consistently over several heart cycles, some form of ECG synchronization is required. When coronary imaging using mechanical 4-slice CT emerged at the beginning of the 21st century, a spiral CT scan mode was applied for continuous, temporally overlapping data acquisition, after which images were reconstructed for the desired cardiac phases based on a recorded ECG trace. This robust technique allows for correction for rhythm irregularities. Often, it was necessary to reconstruct many phases to find a dataset with minimal motion artifacts, given the slower scanner rotation at the time. Although retrospectively ECG-gated spiral-mode cardiac CT is still available and applied in patients with arrhythmia or when full-cycle data are needed, for routine coronary imaging, this scan mode has been largely replaced by more dose-considerate techniques. The current default scan technique for coronary CTA is the prospectively ECG-triggered axial scan mode, also referred to as the prospective scan mode, or step-and-shoot mode (Fig. 12-3). Contrary to the spiral scan mode, using prospectively ECG-triggered axial imaging, each consecutive stack of data is acquired by roentgen exposure limited to the desired cardiac phase, based on the preceding heart rhythm, while the table remains in a stationary position. Contemporary axial scan protocols include arrhythmia recognition algorithms, which interrupt and/or repeat scans in case of a rhythm disturbance, as well as the option to extend the exposure window for reconstruction of different cardiac phases. Although spiral scan protocols have also been modified with the objective to lower dose, axial scan protocols generally achieve the lowest exposure. Second- and third-generation dual-source CT scanners can perform an ECG-triggered spiral imaging at very fast table advancement to cover the entire heart in a single heart cycle. Scanners with very wide detector arrays that completely cover the heart require no table advancement during the scan.
FIGURE 12-3
Cardiac computed tomography (CT) scan techniques. The spiral scan mode can be performed by continuous exposure (green) or with electrocardiogram (ECG)-triggered tube modulation and nominal exposure limited to the phase(s) of interest. Depending on the selected table speed and heart rate, a degree of overlap will be created between the consecutive acquisitions per heart cycle. Axial scan protocols minimize the exposure to the phase of interest but can be extended to allow for reconstruction of more phases (padding), with minimal longitudinal overlap between acquisitions. Second and third dual-source CT systems can perform high-pitch spiral scans, in which case all data are acquired (over a longer interval) within a single heart cycle. The purple bar superimposed over the ECG represents the minimal exposure duration for reconstruction of a single dataset/phase.

Until recently, limitations in computer performance necessitated the use of filtered back-projection techniques for the reconstruction of CT images. As a result of accelerated computer processing power, computationally more demanding iterative reconstruction algorithms can now be applied to CT data at acceptable reconstruction times. The advantage of iterative reconstruction algorithms is the improved image quality. In addition to axial scan protocols, more dose-aware operating techniques, and more efficient tube settings, iterative reconstruction algorithms have contributed to the lower radiation exposure of contemporary coronary CTA by reducing noise levels at lower tube output settings.6,7 Reported dose values peaked at around 15 mSv with the first 64-slice scanners8 but, since then, have gradually dropped to well below 5 mSv using standard CT technology and even below 1 mSv using state-of-the-art technology in selected populations.9
A cardiac CT scan is essentially a large stack of overlapping axial slices. Each image slice consists of 512 × 512 image elements, whereas the dimensions of each 3-dimensional image element, called a voxel, are approximately 0.3 × 0.3 × 0.4 mm. The images can be assessed slice by slice or with the use of secondary postprocessing techniques to visualize longer sections of the coronary arteries and facilitate interpretation of obstructive disease. These secondary reconstruction techniques include double-oblique cuts, curved cross-sections along the axis of the vessel, and 3-dimensional volume-rendered reconstructions. Often a combination of techniques is used for interpretation. Guidelines recommend classification of the stenosis severity into categories rather than exact percentages of lumen narrowing (Table 12-1).10 Standardized coronary classification systems may be used to specify the location of disease. The recently published Coronary Artery Disease Reporting and Data System (CAD-RADS) criteria can be used to classify coronary CTA findings.11
0 | Normal | Absent plaque and no lumen narrowing |
1 | Minimal | Plaque with <25% narrowing |
2 | Mild | 25%-49% narrowing; probably not flow limiting |
3 | Moderate | 50%-69% narrowing; possibly flow limiting |
4 | Severe | 70%-99% narrowing; probably flow limiting |
5 | Occluded | 100% occluded |
Over the past 2 decades, noninvasive coronary CTA has gradually evolved into a reliable technique for the assessment of obstructive coronary artery disease (Fig. 12-4). Many single-center studies, as well as a number of multicenter trials, have been performed to test the technical accuracy of coronary CTA against invasive coronary angiography (Table 12-2).12-17 The sensitivity of CTA is high (93%-100%) on a per-patient level, and the probability of coronary disease is very small in case of a normal CT angiogram because the per-patient negative predictive value approaches 100%. The specificity of CTA is slightly lower (78%-96%), which means that the angiographic disease severity is overestimated in comparison to invasive angiography. There are several contributory factors to this. The spatial resolution of CTA prevents visualization of small vessels, but also increases the apparent size of calcifications and other high-attenuating material in combination with filtering effects. The appearance of obstruction may be created by artifacts, including motion blurring and misalignment between data stacks. The propensity toward overcalling stenosis severity may also be the indirect result of the visualization of atherosclerotic plaque. Similar to intravascular ultrasound the perceived stenosis severity on CTA may increase in the presence of large plaques and outward vessel remodeling (Fig. 12-5). Naturally, the performance of CTA will improve with better image quality. Nonmodifiable technical and patient characteristics, such as temporal resolution and longitudinal coverage or the heart rhythm and size of the patient, need to be factored into the expectations for the examination. A high calcium burden decreases the diagnostic performance of CTA,18 and patients with a very high calcium score may benefit more for another type of testing than CTA. Aspects amenable to optimization include patient cooperation and heart rate. Adequate patient instructions and practice can avoid breathing and other patient movement during the scan. β-Blockers are recommended for all patients with a heart rate over 65 to 70 bpm to reduce motion artifacts and allow for more dose-efficient scan protocols. A fast-acting β-blocker may be administrated orally or intravenously. Alternatively, calcium antagonists or sinus node inhibitors may be used to slow the heart rate. Nitroglycerin is administrated sublingually for coronary vasodilation and improves image quality.
Sensitivity | Specificity | Positive Likelihood Ratio | Negative Likelihood Ratio | |
---|---|---|---|---|
Segments | 91% (86%-95%) | 96% (94%-97%) | 22 (15-31) | 0.09 (0.06-0.15) |
Vessels | 97% (95%-98%) | 93% (89%-96%) | 14 (9-22) | 0.03 (0.02-0.05) |
Patients | 100% (98%-100%) | 89% (85%-92%) | 9 (7-13) | 0.00 (0.00-0.02) |
FIGURE 12-5
Invasive angiography (A and C) and computed tomography angiography (CTA) (B and D). In the proximal left anterior descending coronary artery (LAD), a noncalcified lesion (arrow) can be observed on CTA, corresponding with a similarly severe lesion on invasive angiography. CTA also shows a large calcified plaque in the distal left main (arrowhead). Stenosis appears more severe on computed tomography compared to invasive angiography due to a combination of outward vessel remodeling and blooming effect of the highly attenuating calcium. D1, first diagonal branch; LCX, left circumflex coronary artery.

Cardiac CT is regarded as the clinical reference for the in vivo anatomic depiction of coronary anomalies (see Fig. 12-2). Without the need for selective catheter intubation, aberrant branches will opacify regardless of whether the origin of the vessel is known. The exact course, the relation with other structures, and the termination of fistulas can be visualized unambiguously. Mechanical effects like intra-arterial compression may be displayed using dynamic cardiac CT reconstructions. Myocardial bridging is frequently observed on CT angiograms.
Without injection of contrast medium, ECG-synchronized CT can be used to image and quantify calcium deposits in the coronary artery wall. Although there are many ways to quantify the coronary calcium burden, the semi-quantitative Agatston score remains the most widely used method (Fig. 12-6).1 Population studies have demonstrated an age-, sex-, and race/ethnicity-dependent distribution and progression of coronary calcium.19,20 The calcium score is associated with the risk of adverse cardiac events, adds (substantial) prognostic value to conventional risk predictors, and appears to outperform other secondary risk predictors such as C-reactive protein, N-terminal pro-B-type natriuretic peptide, or carotid intima-media thickness.21-23 The calcium score can correctly reclassify the risk of asymptomatic individuals and appears most effective in those at intermediate risk by conventional factors.24,25
FIGURE 12-6
A. Coronary calcium imaging. Nonenhanced cardiac computed tomography (CT) shows calcified disease in the aortic root and the coronary arteries (3-dimensional [3D] rendered reconstruction). B. Automatically highlighted tissues with an attenuation value above 130 HU and belonging to the coronary arteries can be manually selected, after which an Agatston score is calculated based on the calcified lesion areas and maximum attenuation value per lesion. C. 3D-rendered CT angiogram of the same patients showing the extensive calcification in relation to the coronary anatomy. LAD, left anterior descending coronary artery; LCX, left circumflex coronary artery; RCA, right coronary artery.

In addition to the calcified components, CTA also has the ability to show noncalcified plaque tissue (see Figs. 12-4 and 12-5). The global angiographic disease burden is associated with clinical outcome.26-28 In addition to the stenotic disease burden, the presence of nonobstructive plaque also predicts adverse events. When coronary disease is absent on CTA, the probability of an adverse cardiac event is extremely low over the subsequent 6 years.29 Because differentiation from the perivascular tissues is more difficult, the sensitivity for the detection of noncalcified plaque is lower than for calcified plaque, and absolute quantification remains only moderately reproducible between different software packages.30 Based on measured attenuation values, the differentiation of lipid-rich (lower attenuation values) and fibrous-rich plaques (higher attenuation values) has been demonstrated in several studies.31,32 However, absolute values depend on contrast enhancement and scan conditions; within studies, overlap between plaque types is considerable, and between studies, the reported thresholds vary substantially.33 Various plaque characteristics associated with plaque rupture can be reproduced by CTA, including plaque size, large low-attenuating plaque, outward vessel remodeling, and calcified nodules.34,35 Identification of large lipid cores on CTA has been reported by a number of investigators. While it is not possible to image several other parameters of plaque instability, such as cap thickness, macrophages, or intraplaque hemorrhage, prospective studies suggest that the aforementioned CT characteristics, in addition to plaque progression, predict the occurrence of adverse cardiovascular events.36,37
Based on the diagnostic performance of CTA, in particular the high negative predictive value in comparison to invasive angiography, the technique is thought to be most effective in patients with a low to intermediate probability of coronary disease.16,38 The 2014 American College of Cardiology (ACC)/American Heart Association (AHA) guidelines for stable ischemic heart disease included several recommendations on the use of coronary CTA (Table 12-3).39 Coronary CTA angiography was considered a reasonable first-line option in patients with an intermediate probability of coronary artery disease (CAD), in particular for patients with contraindications to stress testing. Coronary CTA may also be considered for patients with a nonconclusive stress test result. Coronary CTA should only be considered if adequate CT scanner technology and expertise are available and patient characteristics suggest that diagnostic image quality can be achieved. Since publication of the American and European guidelines, the results of several randomized controlled trials on the clinical effectiveness of coronary CTA compared with current standard diagnostic management were published. The largest of these, the National Institutes of Health–sponsored PROMISE trial, randomized over 10,000 patients between coronary CTA and standard care (mostly stress imaging).40 Both strategies performed equally well in terms of hard end points, which occurred at a lower rate than originally anticipated. The use of CTA was associated with a higher rate of catheterizations and revascularizations, but a lower rate of normal invasive angiograms. The Scottish SCOT-HEART trial tested whether the addition of cardiac CT to standard care (including exercise ECG) improved diagnostic confidence.41 In addition to an increased certainty about the diagnosis, secondary analysis demonstrated a lower event rate in patients in whom CTA was added.42 The CRESCENT trial randomized 350 patients between a tiered cardiac CT protocol and standard care (mostly exercise ECG).43 In this study, CTA was only performed in patients with a positive calcium scan or high pretest probability, which reduced radiation exposure and cost, without a negative effect on outcome. Meta-analyses of these trails suggest an overall increase in subsequent catheterization and revascularization rates after CTA, but also suggest a lower myocardial infarction rate.44
Recommendation | Class (Level of Evidence) |
---|---|
2012 ACC/AHA: Stable Ischemic Heart Disease39 | |
Patients with suspected stable IHD who require noninvasive testing | |
CCTA is reasonable for patients with an intermediate pretest probability of IHD who: a) have continued symptoms with prior normal test findings, or b) have inconclusive results from prior exercise or pharmacologic stress testing, or c) are unable to undergo stress with nuclear myocardial perfusion imaging or echocardiography | IIa (C) |
CCTA might be reasonable for patients with an intermediate pretest probability of IHD who have at least moderate physical functioning or no disabling comorbidity. | IIb (B) |
For patients with a low to intermediate pretest probability of obstructive IHD, noncontrast cardiac CT to determine the CAC score may be considered. | IIb (C) |
Patients with known stable IHD who require noninvasive testing for risk assessment | |
CCTA can be useful as a first-line test for risk assessment in patients with stable IHD who are unable to exercise to an adequate workload regardless of interpretability of ECG. | IIa (C) |
CCTA can be useful for risk assessment in patients with stable IHD who have an indeterminate result from functional testing. | IIa (C) |
CCTA may be reasonable for risk assessment in patients with stable IHD who are able to exercise to an adequate workload but have an uninterpretable ECG. | IIb (B) |
CCTA might be considered for risk assessment in patients with stable IHD unable to undergo stress imaging or as an alternative to invasive coronary angiography when functional testing indicates a moderate- to high-risk result and knowledge of angiographic coronary anatomy is unknown. | IIb (C) |
Pharmacologic stress imaging (nuclear MPI, echocardiography, or CMR) or CCTA is not recommended for risk assessment in patients with stable IHD who are able to exercise to an adequate workload and have an interpretable ECG. | III (C) |
2014 ACC/AHA Non–ST-Segment Elevation Myocardial Infarction52 | |
In patients with possible ACS and a normal ECG, normal cardiac troponins, and no history of CAD, it is reasonable to initially perform (without serial ECGs and troponins) coronary CT angiography to assess coronary artery anatomy. | IIa (A) |
2013 ACC/AHA Assessment of Cardiovascular Risk53 | |
If, after quantitative risk assessment, a risk-based treatment decision is uncertain, assessment of 1 or more of the following may be considered to inform treatment decision making: family history, hs-CRP, CAC score, or ABI. | IIb (B) |
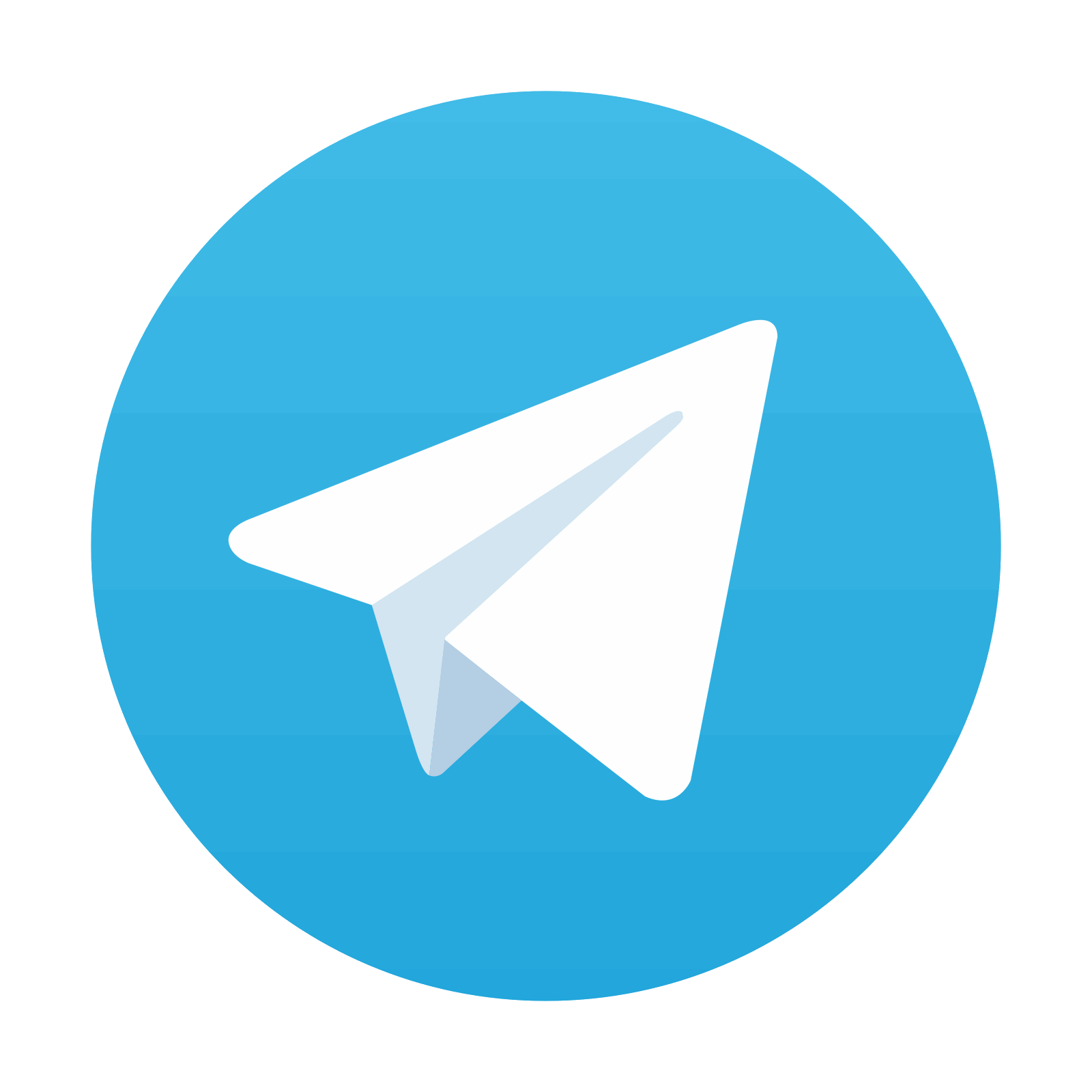
Stay updated, free articles. Join our Telegram channel

Full access? Get Clinical Tree
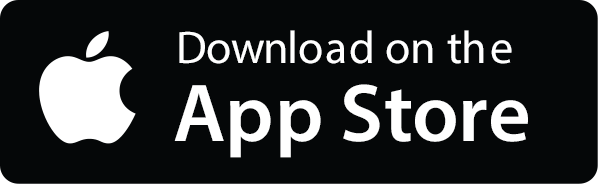
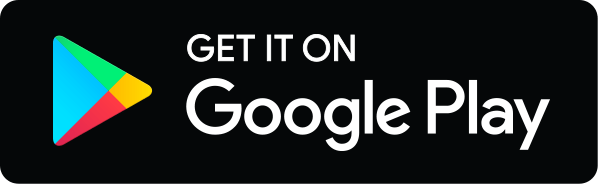
