Advances in computed tomography (CT) technology have revolutionized the diagnosis of cardiovascular disease. CT has dramatically reduced, and for some clinical scenarios eliminated, the need for additional testing such as diagnostic arterial catheterization. In the process, CT has become invaluable in cardiac diagnosis and surgical planning.
CT is based on an x-ray source and detector system mounted on the “CT gantry” that rotates around the patient. Major technology advances have enabled CT to image the beating heart, and routine CT at Brigham and Women’s Hospital (BWH) has noninvasively excluded coronary artery disease in one heart beat (Fig. 6-1) for over 7 years.1 However, the role of CT extends far beyond the coronary arteries alone. Using roughly the same CT acquisition strategies, native coronary imaging can be extended to coronary bypass grafts, the beating myocardium, valve motion, the ventricles and ventricular outflow tracks, and cardiac lesions.
FIGURE 6-1
Selected coronary computed tomography angiography (CTA) image of the proximal left coronary arterial system in a patient scheduled for isolated mitral valve surgery. Using the protocol detailed in this chapter, CT demonstrated normal coronary arteries in this patient, eliminating the need for arterial catheterization.

In order to understand the clinical contribution of CT and to avoid pitfalls in image interpretation, it is essential for the surgeon to appreciate the basic principles of CT used in cardiac imaging. This chapter is divided into two parts. The first part describes the technical considerations for cardiac CT. By understanding each component, the surgeon will be better able to distinguish image artifacts from pathology. The second part reviews those CT examinations that are most frequently performed in the noninvasive cardiovascular imaging program at BWH, detailing the strengths and limitations of each exam.
Most advances in cardiac CT, for example in coronary CT angiography (CTA), have focused on the development of protocols consistent with the rapid incremental technology improvements. One of the major technological advances has been the incorporation of multiple elements into the CT detector system, called Multi-Detector CT (MDCT). MDCT is synonymous with multislice CT. Since all modern scanners have multiple detectors, the semantics can and should be eliminated; this chapter simply uses “CT” to describe the technology.
Data from each of CT detector is used to reconstruct an axial slice perpendicular to the long axis, or z-axis, of the patient. The width of the detectors determines the minimum slice thickness and thus the ability to resolve small anatomic detail (spatial resolution) of the scanner. Thinner slices yield superior spatial resolution; however, comparing two scanners that produce the same number of slices, the scanner with thinner slices will have less z-axis (ie, craniocaudal) coverage per gantry rotation and thus will have a longer scan time. To date, the minimum detector width and largest number of detector rows is 0.5 mm and 320, respectively.2,3 This yields 16 cm (0.5 mm × 320) z-axis coverage per gantry rotation, and thus the entire heart can be imaged with data acquired over a single R-R interval.
Successful cardiac imaging by any modality relies on the ability of the hardware to produce motion-free images or, in other words, to image faster than the heart beats. Because it requires that the gantry be rotated around the patient, CT is inherently slower than digital subtraction angiography (DSA) where each frame corresponds to a single projection image. As described below, CT has become faster so that cardiac CT is now being routinely performed.
Temporal resolution is the metric that measures imaging speed. For a CT scanner with a single photon source, the temporal resolution is one half of the CT gantry rotation time. This is because image reconstruction requires CT data acquired from one half (180°) of a complete gantry rotation. All manufacturers have gantry rotation times on order of 300 ms or less. Using this gantry rotation time as an example, an electrocardiogram (ECG)-gated cardiac image can be reconstructed (using single-segment reconstruction, described below) with CT data acquired over 150 ms of the cardiac cycle. Thus, the reconstructed images inherently display the average of the cardiac motion over the roughly 150 ms during which the data was acquired. This is how ECG gating enables cardiac CT. Without gating, cardiac images are nondiagnostic because the reconstruction “averages” the motion over the entire R-R interval, for example, over 1000 ms for a patient with a heart rate of 60 beats per minute.
There are important strategies to improve temporal resolution. The first uses two independent x-ray CT sources and two independent (64 or greater slice) detector systems built into the CT gantry.4 The second x-ray source is positioned 90° from the first x-ray source, and the second detection system is positioned 90° from the first detection system. With respect to temporal resolution, the practical consequence of this CT configuration is that 180° of gantry rotation can be achieved in half the time (eg, 75 ms as opposed to 150 ms). This halves the temporal resolution (to 75 ms), and thus for this “dual-source” CT configuration, motion is averaged over only 75 ms. Another strategy uses both X-ray CT source-detector systems to sample the entire heart within a single R-R interval by rapidly moving the patient through the scanner.5 Both implementations have technical advantages and disadvantages that are beyond the scope of this chapter. However, cardiac imaging in a single heart beat can be achieved using the same heart rate control as noted below.
For single-source scanners, temporal resolution can be improved by adopting a so-called “multisegment” image reconstruction. The difference between single-segment and multisegment reconstruction is that in the former, 180° of data is acquired from a single heart beat, while multisegment reconstruction uses several heart beats to obtain the one half gantry CT data. For example, in a two-segment reconstruction, two heart beats are used to generate a single axial slice, and thus the temporal resolution is halved. Similarly, if four heart beats are used (four-segment reconstruction), only 45° of data are used from each heart beat. This yields a fourfold reduction in the effective temporal resolution, making it theoretically possible to perform high spatial resolution cardiac CT in patients with a rapid (eg, >70 beats per minute) heart rate. However, since multiple heart beats are used to fill the 180° of gantry rotation necessary for the reconstruction, stable periodicity of the heart is essential. When beat-to-beat variations in heart rate occur, image quality is degraded significantly. In our experience, multisegment reconstruction works well in patients with high heart rates who are being studied for clinical indications where the highest image quality may not be required (eg, studies of graft patency or pericardial calcification). For more demanding applications (eg, native coronary CTA) we still routinely employ beta-blockade for heart rates >60 beats per minute.
As suggested above, beta-blockade is an important component of most coronary-based CT examinations. As the temporal resolution of cardiac CT improves, the dependence on lowering the heart rate will naturally be mitigated. However, the speed of all CT scanners is inferior to coronary catheterization, and thus beta-blockade is recommended for the large majority of patients in whom it is safe. In our experience, many surgical patients have standing orders for beta-blockers as part of their medical therapy, and image quality is excellent. When this is not the case, either oral and/or IV metoprolol is routinely administered.
ECG gating refers to the simultaneous acquisition of both the patient’s ECG tracing and CT data. By acquiring both pieces of information, CT images can be reconstructed using only a short temporal segment of the R-R interval. Each segment is named by its “phase” in the cardiac cycle; the common nomenclature is to name the percentage of a specific phase with respect to its position in the R-R interval. For example, reconstruction of 20 (equally spaced) phases would be named as 0%, 5%, 10%, …, 95%. The period in which the heart has the least motion is usually (but not always) in mid-diastole, near 75%. Thus, the CT exposure (and subsequent patient radiation level) can be lowered by limiting the exposure to a small part of the R-R interval where coronary motion is expected to be a minimum. This is termed “prospective” ECG gating since the reconstruction phase and width is determined prospectively. The disadvantage of this approach is that cine loops of the entire R-R interval cannot be reconstructed because a complete data set is not acquired throughout the R-R interval. If this is desired, the so-called “retrospective” ECG gating can be used, at the expense of higher radiation levels.
For patients who require imaging of bypass grafts, it is important to note that periodic displacement of both saphenous vein grafts (SVG), radial grafts, and internal mammary artery (IMA) grafts is far less than the motion of the native coronary arteries. Thus, for these vessels, a single reconstruction at mid-diastole is usually sufficient (Fig. 6-2), and prospective ECG gating is routinely used. However, the benefits of lower radiation in this population are relatively moot, since the latent period for a radiation-induced malignancy is roughly a decade for blood tumors and significantly longer for solid tumors. Patients under consideration for repeat bypass surgery typically have a shorter life expectancy based on cardiac status. Thus, it is essential that the surgeon not only recognizes that motion has degraded image quality, but also realizes that additional reconstructions, and even repeat imaging, can and should be performed. If the entire course of the graft is not clear to the surgeon at a single cardiac phase, it is almost always the case that another phase will yield motion-free depiction of the graft segment that was poorly seen. Open communication between the radiologist and the surgeon for every case has eliminated this pitfall and ensures that the maximum amount of imaging data is incorporated into presurgical planning.
FIGURE 6-2
ECG-gated CT images from a single reconstruction at mid-diastole for a patient scheduled for redo CABG. The patient is status post-LIMA to LAD coronary bypass grafting. (A) Axial image demonstrates the LIMA graft coursing between two staples and adherent to the posterior table of the sternum. (B) Multiplanar reformatting is now performed routinely to detect and illustrate cases where repeat thoracotomy through the sternal incision is likely to damage a patent LIMA graft. An alternate surgical approach was required for this patient. (C) Selected image from a three-dimensional (3D) volume rendering again demonstrates the course of the graft. Volume rendering fully surveys the thoracic landmarks and is useful for spatial relationships and the communication of important findings.



In cine CT, such as imaging the aortic valve over the entire R-R interval, images are acquired with retrospective ECG gating and subsequently reconstructed throughout the cardiac cycle and then played, in cine mode, to demonstrate function. Each individual image (Fig. 6-3) offers an outstanding assessment of the aortic valve and root structure. Cine CT can also be used to assess ventricular-wall motion. In comparison with magnetic resonance imaging (MRI), the reference standard for global- and regional-wall motion abnormalities, CT often has inferior temporal resolution. However, it is important to emphasize that cine CT does not require a separate image acquisition. The entire CT data set (coronary, valve, myocardium, and pericardium) is acquired in a single breath hold; cine CT is simply part of the image postprocessing.
FIGURE 6-3
ECG-gated CT image through the left ventricle and the aortic valve in a patient status post aortic root repair. Note the pacemaker (right heart wires); magnetic resonance imaging (MRI) was contraindicated. The repair is well visualized and without complication, with only mild aortic valve calcification (cine images showed a tricuspid valve with no significant stenosis). This image also demonstrates a punctate calcified plaque along the superior course of the proximal left main coronary artery, without a significant stenosis.

For surgical patients, CT has the distinct advantage over MR, in that it is by far the best imaging modality to identify and quantify calcification. Also, the most common contraindications for cardiac CT (eg, impaired renal function as measured by glomerular filtration rate or alternatively by serum creatinine) differ from those for MR (pacemaker), and thus CT can often be used for patients who cannot have MR. As noted above, single heart beat cardiac CT is now a clinical reality, with an entire cardiac acquisition in approximately one second.1 In addition to the fact that patient radiation is decreased, multiple scans can be performed with the same injection of iodinated contrast material, creating the opportunity for a host of additional studies including myocardial perfusion6–10 that are, at present, largely in the domain of cardiac MR and nuclear cardiology.
Because ECG gating is required, ascending aorta and cardiac CT delivers more patient irradiation than CT of any other body part. While details regarding cardiac CT dosimetry are beyond the scope of this chapter, discussions regarding CT dose must be based on sound principles. The radiation risk most commonly quoted relates to the probability that the CT scan will result in the development of a fatal radiation-induced neoplasm.11 Human data for radiation at this low level (the level delivered in ECG-gated cardiac CT) is very sparse; all anecdotal reports support a long latency period as described above. For this reason, patients should be separated into two groups: those with a life expectancy of roughly 10 to 15 years or less, and those with a longer life expectancy. In the former group, the only dose consideration is whether the radiation could cause a skin burn (the only short-term complication of any consequence). X-ray skin burns are extremely uncommon, particularly in CT (even for ECG-gated studies), and typically result from multiple exams repeated at short-term intervals. Thus, for this subset of patients, radiation dose should not be a consideration in determining a modality for cardiac imaging. For those patients for whom radiation is an important consideration, prospective ECG gating should be used. X-ray current modulation is standardly used to lower the radiation dose. The tube current (expressed as the mA) is modulated over the course of the cardiac cycle so that the desired (high) diagnostic current is delivered only in diastole. The patient dose is decreased because the tube current is reduced for the remainder of the cardiac cycle. While current modulation is helpful in many cases (eg, pediatric patients), the decision to use it should be made after consultation between surgeon and radiologist because the potential drawbacks are significant. Most importantly, when current modulation is used, images reconstructed during phases with low tube current are relatively noisy because less tube current is used to generate them.
The scan time refers to the time required to complete the CT acquisition along the z-axis of the patient. As described above, better temporal resolution decreases the scan time, not only decreasing cardiac motion, but also enabling breath hold CT. This is important in cardiac CT because in comparison to nongated CT, ECG gating not only increases patient radiation but also increases the scan time.
In practical terms, a 64-slice ECG-gated cardiac CT scan (craniocaudal, or z-axis imaging over ~15 cm) can be performed in roughly 10 seconds, versus 20 to 25 seconds with a 16-slice scanner. One great benefit of wide area detector CT is faster (single heart beat) scans. If this option is not available, increasing the thickness of the detectors increases the z-axis coverage per rotation and thus decreases the scan time. For example, in a patient that cannot perform the breath hold, using thicker detectors (eg, 1 mm thickness as opposed to 0.5 mm thickness) will decrease the scan time by providing more z-axis coverage per rotation. However, routinely increasing the width of the detectors for cardiac applications is undesirable since it degrades the spatial resolution of the examination. In general, spatial resolution refers to the ability to differentiate small detail in an image. This is essential component to coronary imaging since the diameter of the proximal coronary arteries are on the order of 3 to 4 mm. Substitution of 0.5 mm reconstructed images with 1 mm images thus impacts the ability to see detail that may be required for accurate diagnoses. Routine consultation between the surgeon and radiologist is essential to best understand and optimize the tradeoff between scan time and slice thickness. For example, imaging of the myocardium and aorta almost never requires submillimeter slices, because the pathology is larger. Thus, for dyspneic patients who require only imaging of the ascending aorta, thicker slices should be used to cut the scan time.
The scanning parameters that primarily determine the number of photons used to create a CT image are termed “mAs,” or milliamperes-seconds and “kV,” or kilovolts. The former represents the X-ray tube current; the latter refers to the voltage applied within the tube. For the surgeon, choosing the best numbers (typical values are 550 to 700 effective mAs, 120 kV) is far less important than understanding the fact that modern cardiac CT pushes the limits of technology, and thus creates tradeoffs with respect to the x-ray CT source. The source generates photons that are either attenuated by the patient or reach the detectors. When more photons reach the detectors, the image quality is higher because there is less noise. The decision to image with thinner slices (eg, 0.5 mm as opposed to 1 mm) means that fewer photons reach the detector; thus, thinner slices have more noise. This is especially important in obese patients because their increased body mass absorbs more photons than thin patients. For the same effective mAs and kV, images of obese patients can be dramatically degraded by greater image noise.
If there were no limit to the number of photons that an X-ray CT source could produce, the solution would be to simply increase the number of photons (and the radiation dose) until image noise was satisfactory. Unfortunately, because the X-ray CT tube heats excessively when pushed to its maximum, there is a limit to the number of photons that can be produced. This is why image noise becomes problematic with thin slice imaging of obese patients. When this is the case, consultation between the surgeon and radiologist is important because diagnostic images can often be obtained by increasing the image thickness, scanning a smaller z-axis field of view (FOV), or both. The latter can be particularly useful if the examination can be tailored to the most important structure. Scanning a smaller z-axis means that more photons can be generated and used before the X-ray CT tube reaches its heat limit.
On the other hand, whenever possible, the z-axis FOV should be generous, as unexpected pathology can extend in both cranially and caudally. For example, an ECG-gated cardiac and ascending aorta examination to evaluate extension of the intimal flap into the coronary arteries can reveal extension into the great vessels. Also, scanning must allow for variations in the FOV induced by breath holding. As a general rule, for scanning the native coronaries alone, the superior border of the FOV is set at the axial slice corresponding to the top of the carina. This is typically 2 to 3 cm superior to the origin of the left main coronary artery. The inferior border should scan through the entire inferior wall of the heart and should include several slices of the liver to account for cardiac displacement during breath holding. For bypass graft imaging, the superior border of the FOV must include the subclavian arteries and the origin of both IMAs.
Most CT examinations are performed with iodinated contrast material. The exceptions are scans performed solely for the assessment of cardiac and aortic calcification, plus imaging of the aorta and great vessels for aneurysm size measurements alone. Contrast is administered from a peripheral vein, typically with a dual injection system. This injector has two reservoirs to inject contrast followed by saline. For coronary imaging, the contrast and saline delivery are timed so that the left heart, aorta, and coronary arteries are enhanced with contrast while the right heart is filled with saline. The use and the timing of the saline are essential parts of the examinations because artifacts that limit interpretation of the RCA will be induced if the right heart and central veins are densely enhanced with contrast (as opposed to saline).
One of the most common clinical indications for cardiac CT is to evaluate the native coronary arteries for stenosis (Figs. 6-4 to 6-7). Numerous validation studies have evaluated cardiac CT for this purpose.12–25 In these studies, data are typically reported on a per coronary artery segment basis, comparing CTA and DSA. A significant stenosis is generally defined as >50%, determined by quantitative coronary angiography. Data are also analyzed on a per patient basis regarding the value of CTA in ruling-in or excluding CAD. Literature to date reports on patient populations with a relatively high prevalence of CAD (ie, patients already scheduled for DSA). Among the most consistent findings is a very high negative predictive value (NPV) of coronary CTA when performed with 64 or greater detector row scanners. The data and our experience with 320 row scanners suggest a very high NPV, arguing that cardiac CTA can effectively exclude CAD in patients with low to intermediate pretest probability of disease. Furthermore, noncontrast CTA for evaluation of coronary calcium score, an independent predictor of adverse cardiac events and all-cause mortality, is considered to be appropriate for intermediate-risk patients as well as for low-risk patients with family history of premature CAD.26–28
FIGURE 6-4
Proximal RCA 50% stenosis diagnosed by coronary CT angiography and confirmed by conventional angiography. Double oblique maximum intensity projection image (4 mm thick) through proximal RCA (A) and LAO projection still image from conventional angiogram (B) demonstrate a segment of approximately 50% stenosis (arrows).


FIGURE 6-5
Proximal left circumflex greater than 50% stenosis diagnosed by coronary CT angiography and confirmed by conventional angiography. Double oblique maximum intensity projection image (4 mm thick) through proximal LCX (A) demonstrates a segment of calcified and noncalcified plaque with significant luminal narrowing (arrow). Finding is confirmed by true vessel short axis multiplanar reformatted images through the proximal reference (B-left) and the lesion (B-right) which demonstrate minimal residual lumen at the level of the lesion. AP-Caudal projection still image from conventional angiogram (C) confirms a greater than 50% in the proximal LCX (arrow).



FIGURE 6-6
Right coronary artery greater than 50% stenosis diagnosed by CT and confirmed by conventional angiography. Double oblique maximum intensity projection images (4 mm thick) through ongoing RCA at 90° angles (A, B) demonstrate a segment of noncalcified plaque with nonvisualization of lumen (black arrows). The PIV is also partially demonstrated (white arrow). Finding is confirmed by LAO (C) and AP-cranial (D) projection still images conventional angiogram (black arrow). The PIV is also seen (white arrow).




FIGURE 6-7
Proximal LAD less than 50% stenosis diagnosed by coronary CT angiography. Double oblique maximum intensity projection image (4 mm thick) through proximal LAD (A) demonstrates a segment of noncalcified plaque that is not associated with any significant luminal narrowing (arrow). True vessel short axis multiplanar reformatted images through the lesion (B-right) and through proximal reference segment (B-left) confirm minimal luminal narrowing. Low-density (ie, noncalcified) plaque with positive vessel remodeling is seen (arrow). This case highlights the ability of CTA to detect early stages of subclinical atherosclerosis. This lesion would presumably not have been detected at a conventional angiogram.


Consequently, CTA has become increasingly useful for the cardiac surgeon in managing patients scheduled for noncoronary cardiac surgery. If the clinical suspicion is low, but not insignificant, CTA affords the surgeon a method of assessing coronary disease without subjecting the patient to femoral arterial puncture with its known complications. For example, patients undergoing isolated mitral valve surgery for degenerative, myxomatous disease have a low prevalence of CAD, making CTA an ideal alternative to conventional angiography to exclude significant CAD. When the CT protocol described in Part 1 is followed, high-quality imaging is routine, and when CTA excludes CAD, surgeons have increased confidence in using CTA alone. Follow-up with DSA can be reserved for those patients who might benefit from catheter-based intervention.
Current guidelines from all major societies support the use of cardiac CT for evaluation of low- to intermediate-risk patients while also being appropriate even for the high-risk CAD patients.28–32
Cardiac CT provides the cardiac surgeon with a noninvasive method to assess graft patency after coronary artery bypass graft (CABG). Studies using early 16 detector row CT scanners suggest 100% sensitivity and specificity for identifying occluded versus patent grafts,33–35 with benefits from advanced image postprocessing tools.36 In similar fashion, 64- and 320-slice CTA have demonstrated good diagnostic accuracy in the evaluation of significant venous or arterial grafts stenosis, nongrafted, and recipient vessels.37,38 The additional prognostic value of CTA helps enable long-term risk stratification of CABG patients.39 In clinical practice, this application is of value in the evaluation of the symptomatic patient in the early postoperative period in whom graft failure is being considered (Fig. 6-8). It is particularly useful for the demonstration of graft patency in patients with a remote surgical history and unknown graft anatomy prior to DSA, and in patients in whom conventional angiography fails to demonstrate a known graft (Fig. 6-9). In the reoperative setting, such data has virtually revolutionized surgical decision-making and planning prior to surgery in patients who have already undergone CABG. Knowledge of anatomic placement of prior grafts, cannulation sites, and previous incisions have impacted properative planning of the reoperative cardiac surgery patient.
FIGURE 6-8
(A) Postoperative cardiac CT performed for evaluation of graft patency. Three-dimensional volume-rendered image demonstrates patent LIMA to LAD (black arrows), T-graft RIMA to obtuse marginal (white arrows), and SVG to RCA (grey arrows). This study was obtained in a patient 1-day follow off-pump coronary artery bypass grafting. The patient had developed recurrent chest pain and an elevated troponin. Cardiac CT ruled out early graft failure as a cause for the patient’s presentation. (B) Companion case in a different patient. Oblique multiplanar reformatted images demonstrate an acutely occluded saphenous vein graft to obtuse marginal. Note the patent graft stump (black arrow) and thrombosed graft body (white arrows).


FIGURE 6-9
Cardiac CT performed to evaluate possible radial graft occlusion. The patient had surgery 1 month prior and presented with recurrent angina. The radial to RCA graft could not be selective catheterized at conventional angiography and was also not seen at aortic root injection. Three-dimensional volume-rendered image demonstrates patent radial graft (white arrows) to RCA (black arrow). The anastomosis is not seen on this orientation.

Patients with recurrent angina after bypass surgery may have developed stenosis or occlusion in bypass grafts, or may have progression of native coronary disease. In these patients, CTA can be more limited. For example, exclusion of significant stenosis in a graft may be problematic due to metallic surgical clips that cause artifact (Fig. 6-10). Moreover, native CAD in these patients is often advanced and heavily calcified. A large volume of calcium may result in an uninterpretable study for many segments of the native coronaries (Fig. 6-11). CTA for plaque assessment in such patients may not be definitive; consequently conventional angiography may be preferred in this population.40 CTA is increasingly useful for patients with less calcification and less metal artifact.
FIGURE 6-10
Surgical clip artifacts can limit cardiac CT evaluation of coronary bypass grafts. Double oblique maximum intensity projection image (10 mm thick) demonstrates multiple surgical clips placed along the length of a radial to PIV graft (black arrows). Artifact from these metallic clips can partially or completely obscure the adjacent vessel lumen precluding evaluation of these segments for the presence or absence of stenosis. Although CT can unequivocally demonstrate graft patency based on the delivery of contrast throughout the entire course of the graft, surgical clip artifact usually does not allow complete graft evaluation to rule out graft stenosis.

FIGURE 6-11
Cardiac CT is often limited in its evaluation of native coronary arteries in the postcoronary artery bypass graft patient due to the presence of advanced and heavily calcified coronary atherosclerosis. Double oblique maximum intensity projection image (4 mm) demonstrates (A) the proximal LAD (black arrows) and a large first diagonal branch (white arrow) and (B) the proximal right coronary artery (white arrows). The white areas are very high attenuation and represent calcification. All vessel segments demonstrated are heavily calcified. The extent of calcification completely obscures the vessel lumen and presence or absence of stenosis cannot be reliably assessed.
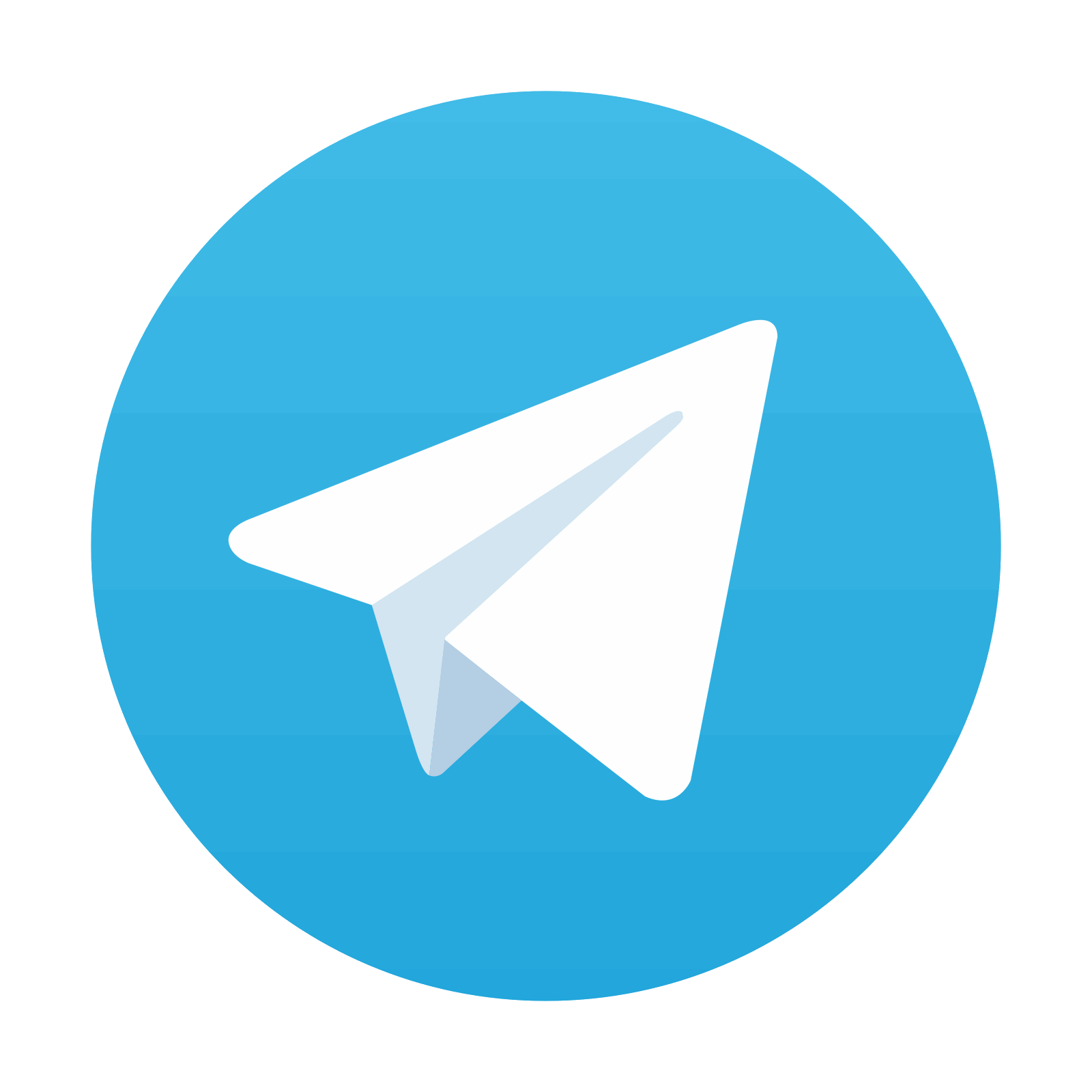
Stay updated, free articles. Join our Telegram channel

Full access? Get Clinical Tree
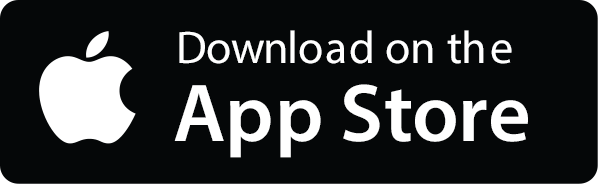
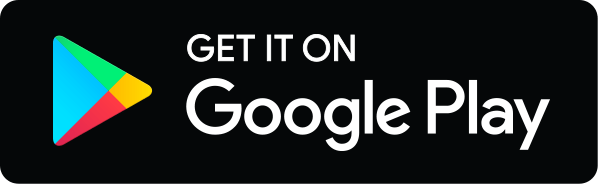
