Background
Recent European Association of Echocardiography and American Society of Echocardiography guidelines on three-dimensional echocardiography state that normal values of left ventricular (LV) parameters for age and body size remain to be established.
Methods
In 226 consecutive healthy subjects (125 women; age range, 18–76 years), comprehensive three-dimensional echocardiographic analyses of LV parameters were performed, and values were compared with those obtained by conventional echocardiography.
Results
Upper reference values (mean + 2 SDs) for three-dimensional LV end-diastolic and end-systolic volumes were 85 and 34 mL/m 2 in men and 72 and 28 mL/m 2 in women, respectively. Indexing LV volumes to body surface area did not eliminate gender differences. Lower reference values (mean − 2 SDs) for ejection fraction were 54% in men and 57% in women and for stroke volume were 25 and 24 mL/m 2 , respectively. Upper reference values for LV mass were 97 g/m 2 in men and 90 g/m 2 in women and for end-diastolic sphericity index were 0.49 and 0.48, respectively. Significant age dependency of LV parameters was identified and reported across age groups. Three-dimensional echocardiographic LV volumes were larger, ejection fraction was similar, and LV stroke volume and mass were significantly smaller in comparison with the corresponding values obtained by conventional echocardiography.
Conclusions
The investigators report a comprehensive analysis of LV geometry and function using three-dimensional echocardiography in a relatively large cohort of healthy Caucasian subjects with a wide age range. These may serve to establish age-specific and gender-specific reference ranges, which are crucial for the routine implementation of three-dimensional echocardiography to detect LV remodeling and dysfunction in clinical practice.
The quantification of left ventricular (LV) size, geometry, and function represents the most frequent indication for an echocardiographic study and is pivotal for patient evaluation and management. Indication for cardiac surgery, suitability for device implantation, indication for medical treatment in systolic heart failure, and suspension of cardiotoxic agents in patients with cancer are among the most critical decisions that rely on accurate assessments of LV geometry and function.
Three-dimensional echocardiography (3DE) enables comprehensive, accurate, and reproducible LV quantitation. The superiority of 3DE over conventional two-dimensional (2D) echocardiography (2DE) for LV volume measurement in comparison with cardiac magnetic resonance (CMR) has been well documented. Although there seems to be a small underestimation of LV volumes using 3DE, this modality provides LV volumes significantly closer to those measured with CMR than 2DE, and no less important, it has been proved to be more robust than 2DE when tested for intraobserver, interobserver, and test-retest reproducibility.
The identification of reference values for LV volumes, mass, and function is a prerequisite for the routine clinical application of quantitative 3DE. Indeed, both the European Association of Echocardiography and the American Society of Echocardiography recommend 3DE rather than 2DE for the routine clinical assessment of LV volumes. However, they also acknowledge that limited normative data are available, particularly because of the lack of gender-based and anthropometric analysis. These aspects have significantly contributed to the limited adoption of 3DE in routine clinical practice.
Accordingly, we designed this prospective, single-center, observational study to (1) perform a comprehensive analysis of LV geometry and function using state-of-the-art 3D echocardiographic equipment in a relatively large cohort of healthy subjects, (2) assess the effects of age and gender on LV 3D echocardiographic parameters, and (3) compare the values measured by 3DE with those obtained by conventional echocardiography in the same subjects.
Methods
Study Population
Between October 2011 and February 2013, 257 healthy Caucasian volunteers were prospectively recruited at a single tertiary center among hospital employees, fellows in training, their relatives, and individuals who underwent medical visits for driving or working licenses and met the inclusion criteria. The sample size of the study was determined according to Altman, who set 200 subjects as the minimum number to enroll in a study aiming to assess reference values for biologic variables. Prospective criteria for recruitment included age > 17 years, no history of cardiovascular or lung disease, no symptoms, absence of cardiovascular risk factors (i.e., systemic hypertension, smoking, diabetes, dyslipidemia), no cardioactive or vasoactive treatment, and normal results on electrocardiography and physical examination. Exclusion criteria were athletic training, pregnancy, body mass index > 30 kg/m 2 , and poor apical acoustic window, defined as more than two LV segments not adequately visualized without contrast infusion.
Blood pressure was measured in all subjects immediately before the echocardiographic examination. Height and weight were measured using a calibrated stadiometer and scale, and body surface area (BSA) was calculated according to the formulas of both Dubois and Dubois (BSA DB ) and Haycock et al. (BSA H ) (modified by Sluysmans and Colan ).
The study was approved by the University of Padua ethics committee (protocol 2380 P, approved on October 6, 2011), and written informed consent was obtained from all volunteers before screening for eligibility in the study.
Image Acquisition
Using a standardized protocol, all examinations were performed by three cardiologists with experience in research echocardiography (L.D.B., D.M., and L.P.B.) using a commercially available Vivid E9 ultrasound machine (GE Vingmed Ultrasound AS, Horten, Norway) equipped with M5S and 4V probes for 2DE and 3DE, respectively. All patients were examined in the left lateral position using grayscale second-harmonic 2D imaging, with the adjustment of image contrast, frequency, depth, and sector size for adequate frame rate and optimal LV border visualization. Care was taken to avoid LV foreshortening in both apical views, and image acquisition was done during breath holding to minimize respiratory movements.
Standard transthoracic echocardiography included (1) 2D-guided M-mode tracings of LV wall thickness and cavity diameter to calculate LV mass; (2) a zoom image of the LV outflow tract obtained from the parasternal 2D long-axis view; (3) spectral recordings of LV outflow tract flow sampled using a pulsed-wave Doppler sample volume positioned just proximal to the aortic valve, so that the location of the velocity recording could match the LV outflow tract measurement; and (4) apical four-chamber and two-chamber view recordings for LV volume measurements, taking care to maximize LV length and to reduce depth by excluding the left atrium to achieve the highest frame rate. All tracings and recordings contained at least three cardiac cycles to allow averaging of measurements.
Three-dimensional echocardiographic full-volume LV data sets obtained by stitching together four or six consecutive electrocardiographically gated subvolumes were acquired by the same examiner at the end of the standard 2D echocardiographic examination. Using multislice display of the 3D LV data set, special care was taken to adequately visualize all LV segments and to exclude any stitching artifacts between subvolumes. Data sets were stored digitally in raw-data format and exported to a separate workstation for analysis (EchoPAC BT 11; GE Vingmed Ultrasound AS).
Image Analysis
Image quality check and analysis were performed offline by two independent observers (D.M. and L.P.B.) with 3 years of experience with the software package used in this study. LV mass was calculated using measurements performed on 2DE-guided M-mode tracings according to the American Society of Echocardiography’s leading-edge convention. To calculate LV stroke volume, LV outflow tract diameter was measured in the parasternal long-axis view in midsystole, inner edge to inner edge, parallel and proximal (within 0.5 cm) to the aortic valve plane. End-diastole and end-systole were identified from 2D echocardiographic cine loops using frame-by-frame analysis of the apical four-chamber and two-chamber LV views as the largest and smallest cavity during the cardiac cycle, respectively. Then, manual tracing of endocardial border was performed in both frames, paying attention to include the papillary muscles within the LV cavity. LV volumes were calculated using the biplane disk-summation algorithm (modified Simpson’s rule), and ejection fraction was calculated as 1−end-systolic volume/end-diastolic volume. Two-dimensional echocardiographic end-diastolic sphericity index was calculated from LV end-diastolic volume and LV longitudinal axis (averaged from the LV longitudinal axes in four-chamber and two-chamber views) by applying the same formula as for 3D echocardiographic sphericity index (see below).
Quantification of 3D LV volumes and ejection fraction was performed using a commercially available software (4D AutoLVQ; GE Vingmed Ultrasound AS) previously validated against CMR and extensively described elsewhere ( Figure 1 ). Initialization of LV endocardial border tracing was manually performed by the operator, by identifying two points on the four-chamber view image at end-diastole and then at end-systole (one point in the middle of the mitral annulus and a second point at the LV apex). Manual editing of the semiautomatically generated endocardial contours was routinely applied to include the LV outflow, as well as papillary muscles and trabeculae within the LV cavity, because this approach was found to reduce bias in comparison with CMR. Editing involved the systematic addition of several attractor points, within a range of one to five points for the endocardium and two to eight points for the epicardium.

In addition, this software enabled the automatic calculation of LV sphericity index ( Figure 1 ) and LV mass ( Figure 2 ). LV sphericity index is a quantitative parameter reflecting LV shape, and it is calculated as the ratio between the LV end-diastolic volume divided by the volume of a sphere whose diameter is equal to the longitudinal LV axis measured at end-diastole. For LV mass calculation ( Figure 2 ), after LV endocavitary volume quantitation, the software automatically detected also the epicardial LV contours in end-diastole and in end-systole. Both endocardial and epicardial contours were verified by the operator and manually adjusted to fit the regional differences in LV wall thickness. Then, LV mass was automatically computed as (LV epicardial volume−LV endocardial volume) × 1.05 g/mL (the specific weight of myocardial tissue).

Statistical Analysis
Normal distribution of variables was verified using the Kolmogorov-Smirnov test. Continuous variables are summarized as mean ± SD, and categorical variables were reported as percentages. Variables were compared between men and women using unpaired t tests. Three-dimensional and 2D echocardiographic measurements obtained from the same subject were compared using paired t tests and Bland-Altman analysis. Pearson’s correlation was used to analyze the relationships between age and LV parameters.
Interobserver variability for 3D LV volumes and mass was analyzed in 15 random subjects by two independent observers (D.M. and L.P.B.). Intraobserver variability was analyzed in another group of 15 subjects by the same observer (D.M.) repeating the measurements 1 week later. For each parameter, reproducibility was reported as the coefficient of repeatability (1.96 SDs of the differences between the two measurements) by Bland-Altman analysis and as the intraclass correlation coefficient (ICC).
All analyses were carried out using SPSS version 19.0 (SPSS, Inc., Chicago, IL) and MedCalc version 10.0.1.0 (MedCalc Software, Mariakerke, Belgium). Differences among variables were considered significant at P < .05. Upper and lower limits of normality were computed as mean ± 2 SDs.
Results
Of the 258 healthy volunteers screened for eligibility, 32 (12%) were excluded for unknown cardiac pathology detected by echocardiography ( n = 5), obesity ( n = 5), professional athletic activity ( n = 3), elevated blood pressure levels at enrollment ( n = 5), premature ventricular contractions ( n = 2), pregnancy ( n = 1), and right bundle branch block ( n = 1). Another seven healthy subjects were excluded because of poor apical acoustic windows and three because of technical inadequate 3D LV data sets identified during image analysis. Thus, the overall feasibility in our study was 88%.
Temporal resolution of 3D data sets was 34 ± 5 volumes/sec, and for 2D data sets, resolution was 76 ± 7 frames/sec. The quality of 3D data sets for LV quantitation was judged subjectively, considering the signal-to-noise ratio, the degree of blood-tissue contrast, and the completeness of LV wall visualization; image quality was excellent in 31%, good in 45%, fair in 20%, and poor in 4% of subjects. The quality of 2D LV data sets for LV quantitation by the disk-summation method was verified by calculating the differences in the LV longitudinal axes in the four-chamber versus two-chamber view; <10% difference was found in 96% of subjects at end-diastole and in 90% at end-systole.
Table 1 summarizes the demographics and the 3D echocardiographic LV parameters of geometry and function in the study population taken as a whole and separately by gender. Subjects’ age range was 18 to 76 years, and 45% were men. There was no difference in age or heart rate between men and women. However, women showed significantly smaller body size and lower blood pressure levels than men ( Table 1 ).
Variable | Overall ( n = 226) | Men ( n = 101) | Women ( n = 125) | P |
---|---|---|---|---|
Age (y) | 44 ± 14 | 43 ± 14 | 44 ± 15 | .584 |
Height (cm) | 170 ± 9 | 177 ± 7 | 164 ± 7 | <.0001 |
Weight (kg) | 68 ± 11 | 76 ± 9 | 61 ± 8 | <.0001 |
Body mass index (kg/m 2 ) | 23 ± 3 | 24 ± 3 | 22 ± 3 | <.0001 |
BSA DB (m 2 ) | 1.78 ± 0.18 | 1.93 ± 0.13 | 1.66 ± 0.12 | <.0001 |
BSA H (m 2 ) | 1.30 ± 0.03 | 1.33 ± 0.22 | 1.28 ± 0.22 | <.0001 |
Heart rate (beats/min) | 67 ± 10 | 67 ± 11 | 68 ± 10 | .338 |
Systolic BP (mm Hg) | 123 ± 14 | 128 ± 12 | 118 ± 14 | <.0001 |
Diastolic BP (mm Hg) | 74 ± 8 | 77 ± 8 | 71 ± 9 | <.0001 |
LV EDV (mL) | 106 ± 25 | 123 ± 25 | 93 ± 16 | <.0001 |
LV EDV/BSA DB (mL/m 2 ) | 59 ± 10 | 63 ± 11 | 56 ± 8 | <.0001 |
LV EDV/BSA H (mL/m 2 ) | 73 ± 15 | 83 ± 16 | 66 ± 11 | <.0001 |
LV ESV (mL) | 39 ± 11 | 46 ± 10 | 33 ± 7 | <.0001 |
LV ESV/BSA DB (mL/m 2 ) | 22 ± 5 | 24 ± 5 | 20 ± 4 | <.0001 |
LV ESV/BSA H (mL/m 2 ) | 27 ± 7 | 31 ± 7 | 23 ± 5 | <.0001 |
LV SV (mL) | 68 ± 15 | 76 ± 16 | 60 ± 12 | <.0001 |
LV SV/BSA DB (mL/m 2 ) | 38 ± 6 | 39 ± 7 | 36 ± 6 | .001 |
LV SV/BSA H (mL/m 2 ) | 47 ± 9 | 51 ± 10 | 43 ± 7 | <.0001 |
LV ejection fraction (%) | 64 ± 4 | 62 ± 4 | 65 ± 4 | <.0001 |
LV mass (g) | 135 ± 23 | 150 ± 22 | 124 ± 16 | <.0001 |
LV mass/BSA DB (g/m 2 ) | 76 ± 9 | 77 ± 10 | 74 ± 8 | .023 |
LV mass/BSA H (g/m 2 ) | 103 ± 16 | 112 ± 16 | 96 ± 12 | <.0001 |
LV end-diastolic sphericity index | 0.35 ± 0.07 | 0.35 ± 0.07 | 0.34 ± 0.07 | .838 |
LV mass/EDV (g/mL) | 1.30 ± 0.2 | 1.24 ± 0.18 | 1.30 ± 0.18 | <.0001 |
LV volumes were larger in men than in women, even after normalization for BSA DB and BSA H ( Figure 3 ). BSA DB was significantly larger than BSA H , and using BSA H for indexing 3D echocardiographic LV volumes and mass amplified the differences between genders ( Table 1 ). Using BSA DB for indexing, upper reference values (mean + 2 SDs) for 3D LV end-diastolic and end-systolic volumes were 85 and 34 mL/m 2 in men and 72 and 28 mL/m 2 in women, respectively. Using BSA H for indexing, upper reference values (mean + 2 SDs) for 3D LV end-diastolic and end-systolic volumes were 115 and 45 mL/m 2 in men and 88 and 33 mL/m 2 in women, respectively. As expected, LV end-diastolic and end-systolic volumes calculated on 2D echocardiographic images were significantly smaller than those measured from 3D echocardiographic data sets ( Table 2 , Figure 4 ).
Variable | 3DE | 2DE | P | Upper limit by 3DE | Upper limit by 2DE |
---|---|---|---|---|---|
End-diastolic volume (mL) | 107 ± 25 | 97 ± 25 | <.0001 | 157 | 147 |
End-systolic volume (mL) | 39 ± 11 | 35 ± 11 | <.0001 | 61 | 57 |
Sphericity index | 0.35 ± 0.07 | 0.34 ± 0.06 | .35 | 0.49 | 0.46 |
Ejection fraction (%) | 64 ± 4 | 65 ± 4 | <.0001 | 56 ∗ | 57 ∗ |
LV ejection fractions were significantly higher in women ( Table 1 ). However, because LV volumes in women were smaller, LV stroke volumes in women were also found to be significantly smaller than in men, and this difference persisted after normalization for BSA ( Table 1 , Figure 3 ). Lower reference values (mean−2 SDs) for ejection fraction were 54% in men and 57% in women and for stroke volume were 25 and 24 mL/m 2 using BSA DB , and 31 and 29 mL/m 2 using BSA H , respectively. LV ejection fraction was statistically smaller with 3DE than with 2DE, but this difference was clinically irrelevant ( Table 2 , Figure 4 ). LV stroke volume measured with 3DE was significantly lower than tLV stroke volume calculated using the conventional 2D Doppler method (76 ± 16 vs 94 ± 20 mL in men and 60 ± 12 vs 75 ± 12 mL in women, P < .0001 for both; Figure 4 ).
LV shape assessed in terms of sphericity index measured at end-diastole was similar between genders ( Table 1 ). Overall, the end-diastolic LV longitudinal axis was longer by 3DE (8.4 ± 0.7 cm) than its average calculated from 2D echocardiographic four-chamber and two-chamber views (8.2 ± 0.7 cm) ( P < .0001), reflecting LV foreshortening and accounting, at least in part, for the smaller LV volumes by 2DE. When 3D echocardiographic LV end-diastolic sphericity index was compared with the corresponding values obtained by 2DE, no significant differences in LV shape were identified ( Table 2 ).
LV mass was significantly larger in men than in women, and this difference persisted even after indexing to BSA ( Table 1 ). Conversely, LV mass/volume ratios were significantly higher in women than in men. Upper limits of reference values (mean + 2 SDs) were 97 and 90 g/m 2 for 3D echocardiographic LV mass/BSA DB , 144 and 120 g/m 2 for 3D echocardiographic LV mass/BSA H , and 1.60 and 1.66 for 3D mass/volume ratio in men and women, respectively. LV mass measured with 3DE was significantly lower than the value calculated using the M-mode formula in both men (150 ± 22 vs 171 ± 35 g) and women (124 ± 16 vs 141 ± 30 g) ( P < .0001 for both; Figure 5 ).
Table 3 summarizes the relationships of LV parameters with age. LV volumes decreased with aging in both genders ( Table 4 ). This relationship was still evident after indexing to both BSA DB and BSA H and was more evident in women than in men ( Figure 3 ). Three-dimensional echocardiographic stroke volume and LV ejection fraction did not show any significant relationships with age in men, but stroke volume decreased and LV ejection fraction increased significantly with age in women. There was no correlation between age and LV mass or LV mass indexed to BSA DB in men, while LV mass decreased with age in women. The mass/volume ratio increased with age in both genders.
Variable | Men ( n = 101) | Women ( n = 125) | ||
---|---|---|---|---|
r | P | r | P | |
EDV (mL) | −0.25 | .014 | −0.36 | <.0001 |
EDV/BSA DB (mL/m 2 ) | −0.25 | .012 | −0.42 | <.0001 |
EDV/BSA H (mL/m 2 ) | −0.22 | .026 | −0.35 | <.0001 |
ESV (mL) | −0.28 | .005 | −0.44 | <.0001 |
ESV/BSA DB (mL/m 2 ) | −0.28 | .005 | −0.49 | <.0001 |
ESV/BSA H (mL/m 2 ) | −0.27 | .008 | −0.44 | <.0001 |
SV (mL) | −0.20 | .052 | −0.20 | .031 |
SV/BSA DB (mL/m 2 ) | −0.20 | .053 | −0.25 | .005 |
SV/BSA H (mL/m 2 ) | −0.17 | .098 | −0.20 | .025 |
Ejection fraction (%) | 0.18 | .067 | 0.28 | .002 |
Mass (g) | 0.01 | .940 | −0.18 | .050 |
Mass/BSA DB (g/m 2 ) | 0.03 | .749 | −0.20 | .024 |
Mass/BSA H (g/m 2 ) | 0.12 | .905 | −0.16 | .08 |
Sphericity index | 0.16 | .107 | 0.10 | .256 |
Mass/EDV (g/mL) | 0.32 | .001 | 0.29 | .001 |
Variable | 18–39 y | 40–59 y | ≥60 y | ||||||
---|---|---|---|---|---|---|---|---|---|
Men ( n = 40) | Women ( n = 44) | P | Men ( n = 38) | Women ( n = 54) | P | Men ( n = 24) | Women ( n = 26) | P | |
EDV (mL) | 128 ± 27 (182) | 98 ± 16 (130) | <.0001 | 119 ± 21 (161) | 94 ± 16 (126) | <.0001 | 116 ± 26 (168) | 84 ± 12 (108) | <.0001 |
EDV/BSA DB (mL/m 2 ) | 66 ± 11 (88) | 60 ± 8 (76) | .001 | 61 ± 9 (79) | 56 ± 8 (72) | .021 | 62 ± 12 (86) | 51 ± 7 (65) | .001 |
EDV/BSA H (mL/m 2 ) | 86 ± 17 (110) | 69 ± 10 (89) | <.0001 | 80 ± 13 (106) | 67 ± 11 (89) | <.0001 | 81 ± 17 (115) | 59 ± 8 (75) | <.0001 |
ESV (mL) | 50 ± 11 (72) | 36 ± 7 (50) | <.0001 | 44 ± 9 (62) | 33 ± 5 (43) | <.0001 | 44 ± 11 (66) | 28 ± 6 (40) | <.0001 |
ESV/BSA DB (mL/m 2 ) | 26 ± 5 (36) | 22 ± 4 (30) | <.0001 | 23 ± 4 (31) | 20 ± 3 (26) | <.0001 | 23 ± 5 (33) | 17 ± 4 (25) | <.0001 |
ESV/BSA H (mL/m 2 ) | 33 ± 7 (47) | 25 ± 5 (35) | <.0001 | 30 ± 6 (42) | 23 ± 3 (29) | <.0001 | 30 ± 8 (46) | 20 ± 4 (28) | <.0001 |
SV (mL) | 78 ± 17 (44 ∗ ) | 62 ± 10 (42 ∗ ) | <.0001 | 75 ± 13 (49 ∗ ) | 60 ± 14 (32 ∗ ) | <.0001 | 74 ± 15 (44 ∗ ) | 56 ± 9 (38 ∗ ) | <.0001 |
SV/BSA DB (mL/m 2 ) | 41 ± 7 (27 ∗ ) | 37 ± 5 (27 ∗ ) | .027 | 38 ± 6 (24 ∗ ) | 36 ± 6 (24 ∗ ) | .261 | 39 ± 7 (25 ∗ ) | 34 ± 5 (24 ∗ ) | .016 |
ESV/BSA H (mL/m 2 ) | 53 ± 11 (31 ∗ ) | 43 ± 7 (29 ∗ ) | <.0001 | 50 ± 8 (34 ∗ ) | 44 ± 8 (28 ∗ ) | <.0001 | 51 ± 10 (31 ∗ ) | 40 ± 6 (28 ∗ ) | <.0001 |
Ejection fraction (%) | 61 ± 4 (53 ∗ ) | 63 ± 3 (57 ∗ ) | .016 | 63 ± 3 (57 ∗ ) | 65 ± 4 (57 ∗ ) | .009 | 63 ± 3 (57 ∗ ) | 66 ± 5 (56 ∗ ) | .008 |
Mass (g) | 149 ± 22 (193) | 124 ± 15 (154) | <.0001 | 150 ± 22 (194) | 126 ± 16 (158) | <.0001 | 150 ± 23 (196) | 118 ± 19 (156) | <.0001 |
Mass/BSA DB (g/m 2 ) | 78 ± 9 (96) | 75 ± 8 (91) | .250 | 76 ± 11 (98) | 75 ± 8 (91) | .450 | 79 ± 10 (99) | 72 ± 10 (92) | .027 |
Mass/BSA H (g/m 2 ) | 112 ± 16 (144) | 96 ± 11 (118) | <.0001 | 113 ± 13 (139) | 98 ± 11 (120) | <.0001 | 113 ± 15 (143) | 92 ± 14 (120) | <.0001 |
Mass/EDV (g/mL) | 1.19 ± 0.16 (1.51) | 1.29 ± 0.16 (1.61) | .004 | 1.28 ± 0.20 (1.68) | 1.35 ± 0.20 (1.75) | .078 | 1.29 ± 0.18 (1.65) | 1.42 ± 0.15 (1.72) | .013 |
Sphericity index | 0.33 ± 0.06 (0.45) | 0.34 ± 0.07 (0.48) | .827 | 0.35 ± 0.08 (0.51) | 0.34 ± 0.06 (0.46) | .807 | 0.37 ± 0.08 (0.53) | 0.36 ± 0.07 (0.50) | .684 |
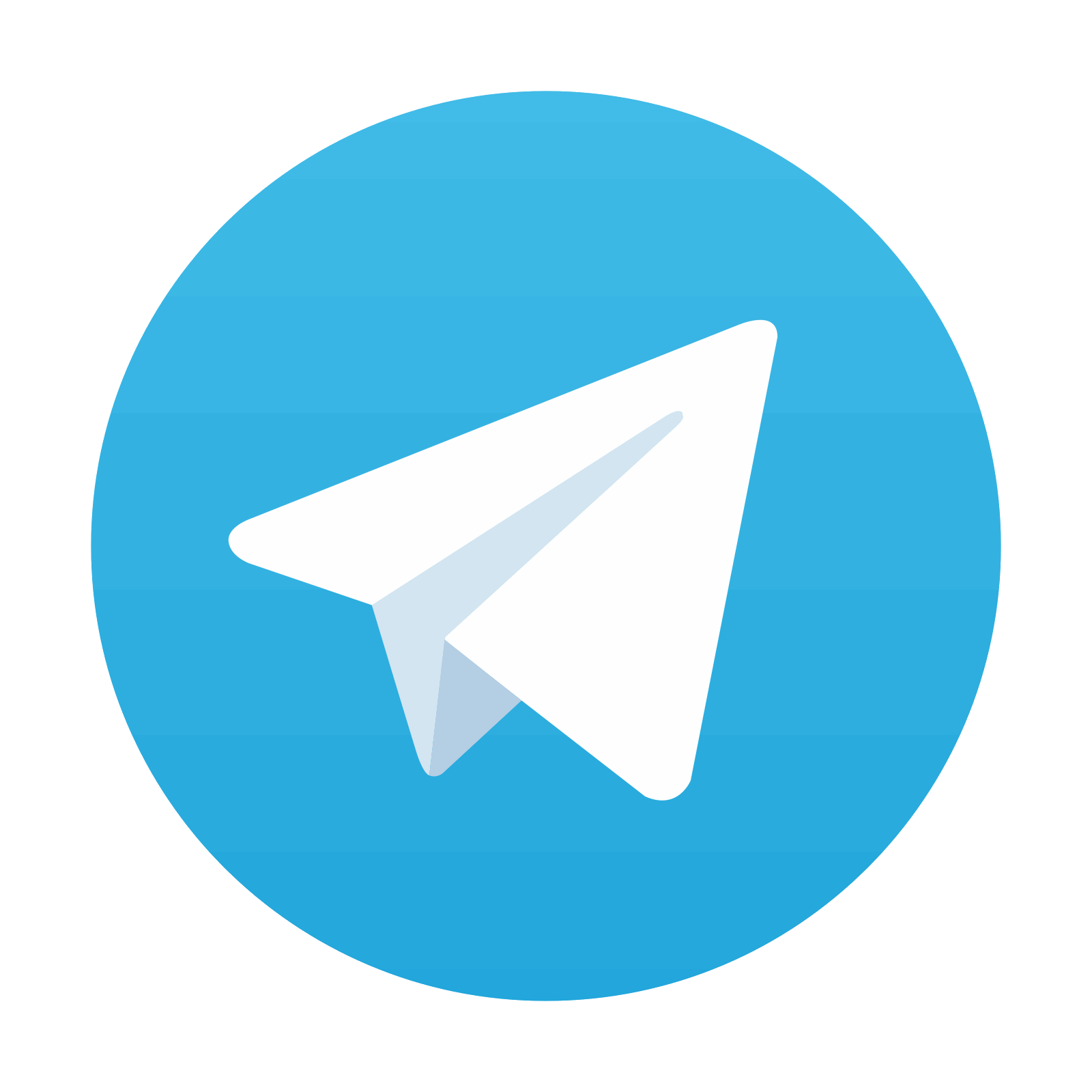
Stay updated, free articles. Join our Telegram channel

Full access? Get Clinical Tree
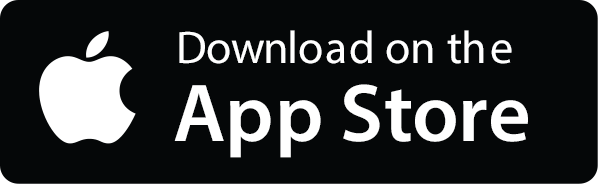
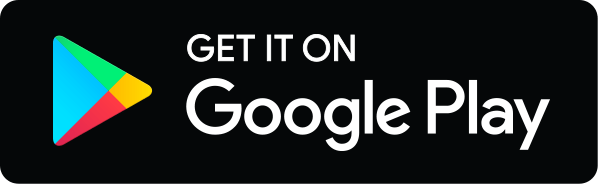
