Abstract
Reported rates of major complications following contemporary catheter ablation procedures vary by as much as five- to eightfold between various types of ablation procedures, ranging from 0.8% for supraventricular tachycardia, 3.4% for idiopathic ventricular tachycardia, 5.2% for atrial fibrillation, and 6.0% for ventricular tachycardia associated with structural heart disease. Death is a rare complication of catheter ablation, occurring in 0.11% to 0.30% of patients with regular supraventricular tachycardia, and in 0.31% of those with ventricular tachycardia. Transseptal catheterization appears to contribute to the cause of death in 0.2% of procedures.
Complications related to vascular access are among the most common problems observed following catheter ablation procedures. Other complications include iatrogenic arrhythmias, cardiac perforation, thromboembolism, air embolism, coronary artery injury, radiation exposure, as well as damage to surrounding extracardiac structures such as the esophagus and phrenic nerves. The optimal management of these complications is prevention. Several strategies have been employed to minimize the risk of complications. Early detection and prompt treatment are essential to improve the outcome after the occurrence of a complication.
Keywords
cardiac perforation, thromboembolism, coronary artery injury, phrenic nerve injury, esophageal injury, pulmonary vein stenosis, radiation exposure
Outline
Local Vascular Complications, 1042
Cardiac Perforation, 1043
Thromboembolism, 1046
Air Embolism, 1047
Coronary Artery Injuries, 1048
Iatrogenic Cardiac Arrhythmias, 1049
Atrioventricular Block, 1049
Macroreentrant Atrial Tachycardias, 1049
Ventricular Arrhythmias, 1049
Abnormal Sinus Node Function, 1049
Valvular Damage, 1050
Phrenic Nerve Injury, 1051
Pulmonary Vein Stenosis, 1054
Incidence, 1054
Mechanism, 1055
Clinical Presentation, 1056
Detection, 1056
Prevention, 1056
Management, 1056
Esophageal Injury, 1056
Incidence, 1056
Mechanism, 1057
Clinical Presentation, 1057
Detection, 1058
Management, 1058
Prevention, 1058
Radiation Exposure, 1062
Biological Effects of Ionizing Radiation, 1062
Nomenclature of Radiation Quantities and Units, 1062
Exposure to Ionizing Radiation During Electrophysiology Procedures, 1063
Clinical Presentation, 1063
Prevention, 1064
Reported rates of major complications following contemporary catheter ablation procedures vary by as much as five- to eightfold between various types of ablation procedures, ranging from 0.8% for supraventricular tachycardia (SVT), 3.4% for idiopathic ventricular tachycardia (VT), 5.2% for atrial fibrillation (AF), and 6.0% for VT associated with structural heart disease. Death is a rare complication of catheter ablation, occurring in 0.11% to 0.30% of patients with regular SVT, and in 0.31% of those with VT. Transseptal catheterization appears to contribute to the cause of death in 0.2% of procedures.
Local Vascular Complications
Incidence
Complications related to vascular access are among the most common problems observed following catheter ablation procedures, and these complications directly impact patient morbidity and increase health care costs. The reported incidence of local vascular complications varies between different studies because of differences in definition of minor and major complications, different types of procedures performed, and different patient population characteristics. The risk of local vascular complications is higher among females, older adults, obese patients, and those with preexisting peripheral vascular disease. In addition, the risk is related to the type of procedure performed (right or left heart catheterization), the size and number of sheaths used during the procedure, as well as the associated periprocedural use of anticoagulant or antiplatelet therapy. The rates of complications related to the site of vascular access are higher following catheter ablation of AF (1% to 13%) and VT (0.7% to 4.7%) compared with ablation of SVT (0.4%).
Detection and Management
Hematomas
Bleeding is the most common vascular complication. Acute bleeding generally can be controlled with prolonged manual compression. Vascular laceration can cause large hematomas in the groin and thigh. Groin hematomas usually resolve in 1 to 2 weeks if ongoing bleeding is stopped. Large hematomas can require blood transfusion, but surgical repair is rarely required. Arteriovenous fistulas and pseudoaneurysms need to be excluded in the setting of large or continually expanding hematomas. Retroperitoneal hematomas are often the result of arterial puncture above the inguinal ligament, allowing bleeding and hematoma to extend to the retroperitoneal space. Retroperitoneal hematomas should be suspected in the setting of a marked drop in hematocrit or unexplained hypotension or flank pain. Abdominal computed tomography (CT) scanning or ultrasound is required to confirm the diagnosis. Retroperitoneal bleeds are generally managed conservatively (bed rest, blood transfusion). Catheter or surgical interventions, however, can occasionally be required.
Pseudoaneurysms
Femoral artery pseudoaneurysms form when a hole through all the layers of the artery at the puncture site fails to seal, allowing persistent blood flow outside the vessel into a space contained by the surrounding tissue. This is in contrast to a hematoma, which has clotted blood outside the vessel with absence of flow. Pseudoaneurysms lack a fibrous wall and are contained by a surrounding shell of hematoma and the overlying soft tissues. Risk factors for pseudoaneurysm development include the use of large vascular sheaths, potent postprocedure anticoagulation, inadequacy of initial effort at hemostasis after removal of sheaths, and punctures of the femoral artery that are too distal—that is, at the level of bifurcation of the femoral artery or below. In addition, multiple attempts at vascular access (arterial or venous) with inadvertent arterial puncture appear to increase the risk for pseudoaneurysm formation; repeated damage to the arterial wall may result in weakening that leads to pseudoaneurysm development.
A pseudoaneurysm typically manifests as a painful pulsatile mass with a systolic bruit or thrill, and the diagnosis is confirmed by duplex ultrasonography. Pseudoaneurysms can be complicated by rupture or distal embolization. Pseudoaneurysms that are less than 2 cm in size and not enlarging often resolve without intervention, and serial imaging is recommended to confirm spontaneous resolution. On the other hand, pseudoaneurysms that are enlarging or are greater than 2 cm in size can be treated by ultrasound-guided compression of the “neck,” connecting the pseudoaneurysm with the vessel or by percutaneous thrombin injection. Occasionally, surgical repair is required, especially for large pseudoaneurysms with a wide connection to the parent artery.
Arteriovenous Fistulas
Femoral arteriovenous fistulas commonly result when bleeding from the arterial puncture tracks into the adjacent venous puncture. Arteriovenous fistulas are more likely to arise when arterial and venous punctures are performed on the same side or when femoral venous puncture is performed below the common femoral artery, where several superficial branches of the femoral artery overlie the femoral vein. The diagnosis is made on examination with the finding of a continuous “to and fro” bruit, and is confirmed by ultrasound. Many of the iatrogenic arteriovenous fistulas are small and close spontaneously within 1 year, but ultrasound-guided compression or surgical repair can be necessary. Because cardiac volume overload and limb damage are highly unlikely with persistent arteriovenous fistulas, conservative management for at least 1 year is reasonable.
Vascular Thromboembolism
Deep venous thrombosis and pulmonary embolism are rare but can result from venous injury, especially during prolonged procedures requiring multiple venous lines, or in the setting of venous compression by a large arterial hematoma. Femoral arterial thrombosis is rare and occurs more commonly in the setting of a small vessel lumen, the use of a large-diameter sheath, preexisting peripheral vascular disease, diabetes mellitus, female gender, and occasionally with vascular closure devices.
Prevention
Accurate vascular puncture and effective initial control of bleeding after sheath removal are the best measures to prevent local vascular complications. Early diagnosis and management of local access site complications are essential to reduce morbidity and improve outcome.
Traditionally, vessel puncture has relied upon an anatomical landmark technique using palpation of local arterial and bony landmarks. However, significant heterogeneity exists in the anatomical relationship of the femoral vein and artery. Overlap of the femoral artery and the common femoral vein occurs along some portion of their course in two-thirds of patients. In addition, high bifurcation of the common femoral artery at the level of the mid femoral head is not uncommon. Such anatomical variation cannot be appreciated using the anatomical landmark techniques, predisposing to accidental puncture of the artery during intended venous cannulation, and increasing the risk of pseudoaneurysm and AV fistula formation.
Real-time ultrasound guidance allows direct visualization of femoral vein size and location relative to the femoral artery, and identification of anatomical variations in arterial and venous spatial relationships, minimizing the risk of inadvertent arterial puncture. Ultrasound also allows easy identification of venous bifurcations, which are unsuitable targets for vascular access. Further, visualization of the vein and of the needle tip trajectory during the puncture allows directing the needle tip into the center of the vein and avoidance of a glancing approach toward the periphery of the vessel.
Although evidence on the value of ultrasound guidance is more robust for internal jugular vein cannulation, several recent nonrandomized studies have found the use of ultrasound to guide femoral venous cannulation in the electrophysiological (EP) laboratory to significantly (by more than 60%) reduce the risk of major and minor vascular access complications, decrease cannulation times, and reduce both the number of unsuccessful attempts and the number of unintended arterial punctures. Using micropuncture needle kits has also decreased vascular complications to a degree, especially in the event of inadvertent arterial access (that resolves more quickly after small-needle entry than with a larger needle).
Cardiac Perforation
Incidence
Previous studies reported the incidence of cardiac perforation with catheter-based interventions as follows: 0.8% for all procedures, 1.5% to 4.7% for valvuloplasty, 0.5% to 0.8% for angioplasty-atherectomy, 0.01% for diagnostic catheterization, 0.1% to 0.2% for EP studies, 0.2% for SVT ablation, 0.4% to 2.7% for VT ablation, and 0.5% to 4.0% for left atrium (LA) ablation.
The incidence of procedure-related cardiac tamponade has increased with the increasing number of catheter ablation procedures for the treatment of AF, which typically involves one or more atrial septal punctures, extensive intracardiac catheter manipulation and ablation within the thin-walled LA, and the need for high levels of systemic anticoagulation during the procedure.
Bleeding into the pericardial space is the most common complication of epicardial ablation procedures using the subxiphoid percutaneous approach. While approximately 10% to 20% of patients experience pericardial bleeding, particularly if inadvertent right ventricular (RV) puncture has occurred, pericardial bleeding of more than 80 mL is observed in up to 7%, though cardiac tamponade and severe bleeding are infrequent. Pericardial bleeding related to the epicardial access procedure is most commonly related to RV puncture. The proximal coronary arteries are covered by epicardial fat along the atrioventricular (AV) groove and the interventricular septum, located away from the typical path of the access needle and thus are less vulnerable to injury. Occasionally disruption of epicardial fat can result in limited bleeding. Pericardial bleeding also can develop later during the mapping and ablation procedure. Laceration of the myocardium or epicardial vessels can occur during manipulation of the pericardial sheath or catheter or as a result radiofrequency (RF) ablation.
Mechanism
Cardiac perforation can be caused by mechanical trauma secondary to catheter manipulation, myocardial tissue rupture due to RF ablation, or inadvertent injury during atrial septal puncture. Extensive catheter manipulation, especially in areas with thin walls such as the LA roof, RV free wall, right ventricular outflow tract (RVOT), RV apex, and atrial appendages, can cause mechanical tear of the chamber wall. Cardiac perforation can also occur away from the area of mapping and ablation, usually caused by penetration of an unattended RV catheter in the setting of rapid and vigorous heart action because of high-dose isoproterenol infusion. Inadvertent puncture of the right atrium (RA) free wall or lateral LA wall can occur during atrial transseptal puncture.
High RF power application can cause excessive tissue heating and boiling of water within the tissue under the electrode. As a result, evaporation and rapid steam expansion can occur intramurally, and a gas bubble can develop in the tissue under the ablation electrode. Continuous application of RF energy causes the bubble to expand and its pressure to increase, which can lead to eruption of the gas bubble (causing a popping sound) through the path with the least mechanical resistance that leaves behind a gaping hole (the so-called steam pop). This often occurs toward the heat-damaged endocardial surface (leading to crater formation) or, infrequently, across the myocardial wall (resulting in myocardial perforation). Steam pops are often associated with a sudden, although small (<10 Ω) impedance rise and a sudden drop in electrode temperature. When steam pops burst toward the endocardial surface, an intense shower of microbubbles is often detected on intracardiac echocardiography (ICE). The risk of steam pops is relatively low with temperature-controlled standard 4-mm-tip RF catheter ablation, since the electrode temperature, which approximates tissue temperature, is limited to a safe level. However, when there is significant discrepancy between tissue and electrode temperature, due to passive or active electrode cooling, tissue temperature can reach the boiling point without being detected by the monitoring electrode temperature.
Detection
Prompt recognition and management of cardiac perforation are critical to prevent the development of cardiac tamponade and potentially life-threatening hemodynamic collapse. Although the presentation often is dramatic with abrupt hypotension, it is often insidious, with a more gradual fall in blood pressure. Continuous blood pressure monitoring using an arterial line can help detect early hemodynamic compromise. Sinus tachycardia is common in the setting of cardiac tamponade that gradually develops. Nonetheless, the absence of tachycardia does not exclude cardiac tamponade; in fact, sinus bradycardia if often observed secondary to a vagal reflex in the setting of a rapidly developing cardiac tamponade, and patients who are dependent on ventricular pacing will not show this. Any chest pain that persists beyond the completion of an ablation lesion, especially if associated with hypotension and diaphoresis, should alert the operator to the possible development of pericardial effusion.
Assessment of the cardiac silhouette fluoroscopically can provide the first clue, especially if a similar assessment was made at baseline. Decreased excursion of the lateral heart border on fluoroscopy in the left anterior oblique (LAO) projection, indicating accumulating pericardial effusion, usually can be seen well before a drop in blood pressure and prior to progression to cardiac tamponade. Cineangiography often reveals a double layer in which the epicardial fat can be visualized separate from the outer pericardial shadow. Some operators obtain a baseline LAO cine image at the onset of a procedure to serve as a reference for comparison during the procedure, followed by intermittent evaluation of the same fluoroscopic projection during the procedure.
Transthoracic echocardiography is the most definitive method for confirming the development of pericardial effusion. ICE, commonly used to guide transseptal puncture for LA procedures, can enable early detection of pericardial effusions before the emergence of tamponade physiology. Most of the pericardial space is not visualized from the RA imaging perspective used to visualize the interatrial septum; advancing the ICE catheter into the RV and rotating the transducer against the interventricular septum can readily identify pericardial effusions ( Fig. 32.1 ).

Perforation without tamponade can occur during placement of diagnostic catheters, or while accessing the LA by transseptal catheterization. Detection of pericardial effusion at this time can prevent progression to tamponade but requires a high index of suspicion. Several clues should alert the operator to the possibility of cardiac perforation, including a ventricular catheter that reaches the edge of the cardiac silhouette, high pacing thresholds at sites despite normal electrograms and apparent good tissue contact on fluoroscopy, right bundle branch block pattern during “RV” pacing, and a catheter tip position discordant from where it should be (i.e., an RV catheter too far leftward with intended RV apical location; eFig. 32.1 ).

With atrial septal puncture for accessing the left heart chambers, the aorta can be inadvertently entered; if only the needle enters the aorta and this is recognized before advancing the dilator and sheath, the needle can be withdrawn and the patient monitored for stability of vital signs and with echocardiography. The procedure can be continued if there is no accumulation of pericardial fluid after 15 to 30 minutes of monitoring. If the dilator and sheath have been advanced into the aortic root before the error is recognized, it is imperative that the sheath not be removed at this time, as this can result in immediate and perhaps irreversible hemodynamic collapse due to intractable bleeding into the pericardial space. A cardiothoracic surgeon should be consulted immediately, who can then remove the sheath and repair the defect in the aortic wall under direct vision.
In addition, during attempted atrial septal puncture, the needle and dilator can climb higher on the right atrial septal wall, where puncture of the free wall occurs, and with continued advancement of the transseptal apparatus, it immediately enters the LA, at which time a left atrial pressure tracing will be observed and fluoroscopic and ICE appearance shows stable LA placement. Only when the sheath is removed later in the procedure does pericardial bleeding occur. Similarly, other catheters (RV, coronary sinus [CS]) may perforate at the time of initial placement and “plug their own hole” until the end of the procedure when they are removed, allowing bleeding into the pericardium.
Management
Cardiac tamponade is the most dramatic complication observed during catheter ablation procedures and can be associated with increased mortality if not managed promptly.
The management of pericardial effusion is largely determined by its relative size and hemodynamic effect. Trivial pericardial effusions, if recognized early during the procedure, should be monitored continuously but do not warrant termination of the procedure. For larger effusions, the procedure should be terminated and anticoagulation, if administered, should be reversed. Protamine is used to reverse the effects of heparin, idarucizumab for dabigatran, four-factor prothrombin complex concentrate for direct factor Xa inhibitors, and activated factor VII, fresh frozen plasma, and vitamin K can be used in patients with therapeutic anticoagulation with warfarin. IV fluids, vasopressors, and transfusion of blood products can be required, depending on the extent of the effusion and the severity of hemodynamic decompensation. IV atropine can be of value in patients with increased vagal tone.
If perforation without tamponade is recognized, an echocardiogram should be obtained as a baseline. If the offending catheter is a standard 5 or 6 Fr shaft, it can often be withdrawn back into the heart while monitoring the echocardiogram for accumulation of pericardial fluid. Most often, there will be no further bleeding into the pericardial space and the procedure can be continued. If systemic anticoagulation is planned for left-sided catheterization, the operator must decide how important it is to continue the procedure with the risk of bleeding into the pericardium. If a larger catheter or large vascular sheath has been inadvertently placed in the pericardial space, echocardiography should be obtained along with immediate cardiothoracic surgical consultation. In many if not most cases, the sheath can be safely withdrawn into the heart without adverse consequences, but the team must be ready to transport the patient to an operating room for repair of a hole or tear in the wall of the affected heart chamber. In some cases, especially when the patient is fully anticoagulated, it may be prudent to transport the patient to the operating room and prepare for urgent sternotomy before removing the catheter/sheath, so as to be able to rapidly enter the chest if hemodynamic collapse occurs.
Although pericardiocentesis typically is required for large pericardial effusions and for smaller effusions manifesting signs of cardiac tamponade, and can effectively restore hemodynamic function, it carries the potential risk of cardiac chamber laceration, inadvertent puncture of the RV, pneumothorax, and infection. In a subset of patients (especially older female patients with a small to moderate amount of pericardial effusion who are not anticoagulated), a conservative approach incorporating administration of IV fluids and vasopressors to address the hemodynamic consequences of cardiac tamponade was found in a recent report to be a reasonable initial strategy and could obviate the need for emergency pericardiocentesis.
Pericardiocentesis
Pericardiocentesis should be performed promptly when indicated because there is usually a narrow therapeutic time window for intervention before critical hemodynamic compromise ensues. The procedure can be performed under fluoroscopic guidance. Echocardiography can also be used to guide pericardiocentesis. When echocardiography is not readily available, fluoroscopy is usually adequate to establish the diagnosis and guide a safe procedure, and reliance on transthoracic echocardiography should not lead to unnecessary delay in diagnosis or therapy. The technique of percutaneous pericardial access via the subxiphoid approach is discussed in Chapter 4 .
Some investigators have proposed the insertion of two drains in the pericardial sac to allow for faster evacuation of blood, followed by continuous aspiration with negative pressure manual suction. This technique can potentially allow the pericardium to abut and oppose the perforation site and “seal” of the bleeding source. Furthermore, rapid evacuation of the pericardial effusion can help prevent the formation of an intrapericardial thrombus ( Fig. 32.2 ).

Autotransfusion of blood removed from the pericardial space can be of value in patients with persistent bleeding, and is best done using an autologous blood recovery system (Cell Saver) because direct autotransfusion can result in a systemic inflammatory response.
Complete drainage of pericardial effusion can be confirmed by ICE, transthoracic echocardiography, or contrast injection into the pericardium. In most patients, an indwelling catheter is required for a short interval after initial drainage to confirm that the bleeding has stopped and that no effusion is reaccumulating. Traditionally the drain is left in the pericardial space for approximately 12 to 24 hours, although recent reports suggest that early removal of drains (within 1 to 4 hours of ensuring cessation of bleeding) is a safe practice and is associated with less patient discomfort and shorter hospitalization. Of note, coagulation of the pericardial blood and intrapericardial thrombus formation can impede effective pericardial drainage and cause restrictive effects on cardiac chamber filling; if this is even a moderate volume, urgent surgical exploration can be warranted, since any additional bleeding that can further compromise the circulation may be difficult to detect and impossible to evacuate with percutaneous drainage. High-dose protamine, especially when combined with prothrombin complex concentrate, can potentially be thrombogenic.
Early pericarditis after cardiac perforation is common. In one report, 53% of patients with cardiac perforation had persistent chest pain (suggestive of pericardial inflammation) after effusion evacuation and removal of the pericardial catheter. Subacute reaccumulation of pericardial fluid (suggestive of post cardiac injury syndrome or inflammatory pericarditis) can also occur, requiring repeat pericardiocentesis. Several measures have been suggested to help reduce the severity of pericardial inflammation: (1) injection of steroids (e.g., 0.5 to 1.0 mg/kg of methylprednisolone or 2.0 mg/kg of triamcinolone) into the pericardial space at the conclusion of the procedure has been shown in animal models to be effective in reducing postprocedural pericarditis and adhesion formation; (2) postprocedural use of colchicine has been shown in small series to reduce postsurgical pericardial inflammation; (3) an administration of antibiotic therapy as long as the pericardial drain is in place; and (4) early removal of pericardial drains. Some patients have persistent mild hypotension and bradycardia for 1 to 2 days after drainage of blood from the pericardial space, causing concern for fluid reaccumulation. This may be due to a prolonged vagal reaction and typically resolves spontaneously.
Surgical Repair
There is currently no consensus on the threshold for surgical exploration and repair of cardiac perforation. Even large effusion (up to 2 L) can be managed with percutaneous pericardiocentesis without surgical intervention. However, continuing intrapericardial bleeding and rapid reaccumulation of pericardial effusion after initial evacuation can suggest cardiac chamber wall laceration rather than microperforation, and should prompt consideration of immediate surgical exploration. Up to 16% of LA perforations during AF ablation require surgical closure.
It is often helpful to identify the potential site of cardiac perforation to guide appropriate surgical exploratory approach. This can often be indicated by the techniques used during the procedure and cardiac chambers being catheterized. However, cardiac perforation caused by a catheter remote from the site of ablation should also be considered.
Measurement of oxygen saturation of aspirated pericardial blood can be helpful diagnostically to assess for venous (right-sided) versus arterial (left-sided) bleed. In addition, the location of the effusion or superimposed thrombus within the intrapericardial space sometimes can suggest the site of cardiac perforation (e.g., posterior effusion in the setting of LA perforation, and anterior effusion in the setting of RV perforation).
Thromboembolism
Systemic thromboembolism can complicate EP procedures in the left side of the heart. The rate of thromboembolic events is 0.4% to 2.1% for AF ablation and up to 2.8% for ablation of VT originating in the LV. In patients undergoing AF ablation, a history of a prior cerebrovascular event is the most potent individual risk factor for postablation cerebrovascular events and is associated with a ninefold increased risk of periprocedural stroke. In addition, the incidence of periprocedural stroke increases in a stepwise fashion with an increasing CHADS2 score.
Mechanism
Potential sources of emboli include thrombus formation on high-profile wires, catheters, and sheaths inserted in the LA or LV, char formation at the tip of the ablation catheter or at the site of ablation, thrombi or air passing through a patent foramen ovale or transseptal puncture, and dislodgment of a preexistent LA or LV thrombus by catheter manipulation. In addition, new LA appendage thrombi can develop in patients with persistent AF after planned or inadvertent conversion to normal sinus rhythm (NSR), especially in the setting of insufficient levels of anticoagulation before, during, or after ablation. Furthermore, endocardial disruption from the ablation lesions can potentially become a nidus for thrombus formation. Also, aortic atheroembolism can occur during retrograde transaortic LV access for ablation of VTs or AV bypass tracts (BTs), and it tends to be associated with difficulty in the manipulation of catheters within a severely diseased aorta.
Thromboembolic events typically occur within 24 hours of the ablation procedure, with the high-risk period extending for the first 2 weeks following ablation. Cerebral thromboembolism is most common, but emboli can also involve the coronary, abdominal, or peripheral vascular circulations. In addition, recent studies demonstrated a high incidence of asymptomatic cerebral emboli (detected on brain magnetic resonance imaging [MRI] in up to 50% of patients) in the first 24 to 48 hours following AF ablation. The pathogenesis and clinical significance of these lesions remain uncertain.
Prevention
Prevention remains the best strategy in minimizing cerebrovascular events during left heart mapping and ablation, and this may be achieved by the following: (1) exclusion of intracardiac thrombi with preprocedural transesophageal echocardiography (TEE) in patients with AF (and transthoracic echocardiography in patients with ischemic VT and cardiomyopathy); (2) aggressive intraprocedural anticoagulation, including early heparin administration (preferably before catheterization of the left heart), followed by continuous infusion to maintain the activated clotting time at greater than 300 seconds; (3) meticulous attention to sheath management, including constant infusion of heparinized saline and air filters; (4) minimizing char formation during lesion creation by regulating power delivery to prevent abrupt impedance rise; and (5) using ICE during AF ablation for early detection of intracardiac thrombi and accelerated bubble formation consistent with endocardial tissue disruption with RF application.
Open-irrigation RF ablation or cryoablation, compared with standard 4- or 8-mm solid tip or closed irrigation electrodes, can potentially decrease the formation of char and thrombus at the tip of the ablation catheter. Administration of large doses of protamine on completion of the ablation procedure to reverse heparin abruptly can potentially promote thrombogenesis and warrants further evaluation to confirm its safety. Also, an uninterrupted oral anticoagulation strategy at the time of AF ablation eliminates a period of inadequate anticoagulation immediately following the ablation procedure, a critical period for thromboembolic risk because of the inflammation and irritation inherently associated with ablation. In patients with severe disease of the aorta, using a long vascular sheath to advance the ablation catheter directly into the LV can potentially reduce the risk of aortic atheroembolism.
Air Embolism
Vascular air embolism is a potentially life-threatening event that can occur during left and right heart procedures. Because many cases of venous air embolism go unnoticed, the true incidence of this complication is unknown. Air embolism has been reported in the interventional radiology literature at an incidence of 0.13%.
Mechanism
Small amounts of air embolized in the venous circulation are generally broken up in the pulmonary capillary bed and absorbed from the circulation without significant sequelae. Embolization of large volumes of air (>5 mL/kg) can cause severe complications (shock or cardiac arrest). However, complications have been reported with as little as 20 mL of air. On the other hand, embolization of as little as 2 or 3 mL of air into the arterial circulation can be fatal.
Paradoxical air embolization into the arterial circulation can occur through direct passage of air into the arterial system via anomalous structures such as an atrial or ventricular septal defect, a patent foramen ovale, or pulmonary arterial-venous malformations. Direct arterial air embolism is caused by the introduction of air into the LA via the transseptal sheath, as well as via long vascular sheaths occasionally used for catheter stabilization during transaortic mapping and ablation in the LV.
With the patient in the supine position, large volumes of air rapidly entering the venous circulation typically lodge in the RVOT or pulmonary artery, and can cause RVOT obstruction, marked reduction of cardiac output, and potentially hemodynamic collapse. Also, air embolization can lead to serious inflammatory changes in the pulmonary vessels, including direct endothelial damage and accumulation of platelets and fibrin. Air in the systemic circulation can induce ischemia by various mechanisms, such as obstruction of the blood flow, vasospasm, and thrombus formation because of platelet activation.
Clinical Presentation
Most cases of venous air embolism are subclinical and do not result in untoward outcomes, and even when symptomatic, they go unrecognized because of the nonspecific nature of clinical presentation that can mimic other cardiac, pulmonary, and neurological dysfunctions. Therefore, a high index of suspicion is necessary to establish the diagnosis.
The outcome of venous air embolism is directly related to the amount of air and the rate at which it enters the vein. Spontaneously breathing patients can experience more serious consequences than those under controlled positive-pressure ventilation because they generate negative intrathoracic pressure during the respiratory cycle, facilitating air entrainment. Awake patients typically manifest shortness of breath, continuous coughing, chest pain, and a sense of “impending doom.” Jugular venous distention, hypotension, tachycardia, and electrocardiogram (ECG) signs of right heart strain (ST-T wave abnormalities) can be observed. Severe cases are characterized by cardiovascular collapse.
Arterial air emboli can distribute to almost any organ and can have devastating clinical sequelae. Direct cerebral air embolism can be associated with altered mental status, seizures, and focal neurological signs. A common presentation of air embolism during LA mapping and ablation is acute inferior ischemia, heart block, or both ( eFig. 32.2 ). This reflects preferential downstream migration of air emboli into the right coronary artery (ostium of the right coronary artery is located more superiorly than that of the left coronary artery in the supine patient). Importantly, atrial-esophageal fistula should be ruled out if air embolism is documented days or weeks after the ablation procedure.

Detection
Routine diagnostic modalities to identify air embolism in the terminal arterial circulations lack sensitivity, and diagnosis is typically based on the appropriate clinical scenario, with possible air identified in cardiac chambers. Air in the RV or pulmonary artery can be visualized on fluoroscopy as well as transthoracic echocardiography. TEE is currently the most sensitive monitoring device for venous air embolism. Prompt CT or MRI obtained before the intravascular air is absorbed can show multiple serpiginous hypodensities representing air in the cerebral vasculature, with or without acute infarction, but imaging later in the course of the disease shows diffuse acute infarcts ( eFig. 32.3 ).

Prevention
The optimal management of air embolism is prevention. Careful sheath management, including constant infusion of heparinized saline and air filters, should be observed. Although air can be introduced through the infusion line, it can also occur with suction when catheters are removed. Therefore whenever catheters are removed, they need to be withdrawn slowly to minimize suction effects, and the fluid column within the sheath should be aspirated simultaneously. The sheath should then be aspirated and irrigated to ascertain that neither air nor blood has collected in the sheath. Importantly, the entire volume of the sheath should be aspirated after initial deployment as well as after each time a catheter is removed and reinserted, to ensure that a continuous column of fluid is present in the sheath and disallowing the possibility of trapped air that could otherwise be introduced when a catheter is advanced through the sheath. Testing to ascertain the sheath’s capacity prior to insertion is a good practice. This is especially important when inserting and removing balloon catheters through large sheaths.
Management
Initially it is essential to take all measures necessary to prevent further air embolization. For venous air embolism, placing the patient in the left lateral decubitus and Trendelenburg position helps air remain in the RA, where it will not contribute to an “air lock” in the RVOT. In addition, direct extraction of air from the venous circulation if localized in the RA or RV can be attempted by aspiration from a central venous or pulmonary artery catheter. Air aspiration should be performed with the patient supine or in a Trendelenburg position while holding his or her breath at the end of inspiration or during a Valsalva maneuver.
Administration of intravenous (IV) fluids and vasopressors may be necessary for hemodynamic support. Supplemental 100% oxygen therapy can reduce the size of the air embolus by increasing the rate of nitrogen absorption from air bubbles. Because gas bubbles are not buoyant enough to counteract arterial blood flow, these measures are more suitable for treating venous and RV air emboli. Supportive care usually results in complete resolution of symptoms and signs within minutes. Suspected coronary arterial air embolism should be investigated with coronary arteriography, especially if ST segments changes persist beyond 2 to 3 min, to exclude spasm or true thrombus.
When cerebral air embolism is suspected, it is important to maximize cerebral perfusion by administration of fluids and supplemental oxygen. Hyperbaric oxygen therapy is regarded as the treatment of choice in patients with cerebral air embolism, and prompt transfer to a hyperbaric oxygen therapy center should be considered. When started within a few hours, hyperbaric oxygen therapy can potentially compress the existing bubbles, speed resolution of bubbles by establishing a high diffusion gradient, improve oxygenation of ischemic tissues, and reduce endothelial thrombo-inflammatory injury.
Coronary Artery Injuries
The incidence of coronary artery injuries during ablation procedures is extremely low (0.06% to 0.1%), despite the close proximity of coronary arteries to common sites of ablation. The low incidence is likely due, at least in part, to the protective effect of convective cooling conferred by the high-velocity blood flow within the epicardial coronary arteries.
The potential for acute coronary artery occlusion is a significant risk consideration with catheter ablation within the aortic sinuses of Valsalva. Damage to the coronary arteries can result from catheter manipulation and ablation in the aortic root, secondary to RF energy delivery in close proximity to the ostium of the right or left coronary artery, as well as inadvertent catheter engagement of the left main coronary artery when ablating in the left sinuses of Valsalva.
It is also important to recognize the potential risk of coronary artery damage when ablating in the RVOT and pulmonary artery. The right coronary artery is typically 4 to 5 mm away from the proximal part of the RVOT near its rightward free wall, and is separated by a variable amount of fat. In addition, the cephalocaudal separation of the pulmonic and aortic valves results in close proximity of the pulmonic valve to the origin of the right coronary artery, and the left main coronary artery lies in immediate posterior proximity to the subvalvular RVOT near the pulmonic valve as well as the supravalvular pulmonary artery.
Because of the proximity of the right coronary artery to the cavotricuspid isthmus (CTI) and the left circumflex artery to the lateral mitral annulus, coronary artery injury can potentially occur during ablation at mitral or tricuspid annuli. Transient inferior ST segment elevation and acute occlusion of the right coronary artery have been reported following ablation of the CTI, and left circumflex artery injury has been reported following ablation of left-sided AV BTs. Also, late stenosis of the right coronary artery has been observed in children with Ebstein anomaly of the tricuspid valve who undergo ablation of a right-sided AV BT, and coronary injury or infarction has occurred during ablation of posterior septal BTs ablated within the CS or middle cardiac vein.
Percutaneous epicardial ablation probably poses a greater risk of coronary artery injury (laceration, intimal hyperplasia, intravascular thrombus formation, or vasospasm), especially in the anterior and posterior septal and the basal ventricular areas, where coronary arteries and veins are known to traverse. The CS and its branches are also in close relation to the distal circumflex and the posterolateral branches of the right coronary artery, and ablation within the coronary venous system (for AV BTs, lateral mitral isthmus, and VT) can cause coronary injuries.
Mechanism
RF ablation-induced acute coronary artery injury can be mediated by vasospasm, intravascular thrombus formation, or direct vessel trauma. Transient thermal irritability resulting in coronary spasm appears to be the most common mechanism. Acute or subacute thrombosis can result from RF-induced functional and morphological damage to the coronary artery media and endothelium. Thermally induced denaturation of collagen fibers can develop in the vessel wall, leading to intimal hyperplasia and subsequent vessel narrowing. In addition, an inflammatory mechanism can result in delayed medial necrosis and intimal hyperplasia causing late stenosis. During epicardial ablation, coronary injury can result from direct disruption of the arterial wall or coronary laceration.
In general, the susceptibility of coronary arteries to thermal damage is inversely proportional to the electrode-to-artery distance. Arterial injury is inversely proportional to vessel diameter; there is little evidence of injury when vessel diameter exceeds 0.5 to 1.0 mm. High RF power delivery in small hearts, such as in pediatric patients, or in direct contact with the vessel can increase the risk of coronary arterial injury.
The high-velocity blood flow within the epicardial coronary arteries confer a protective effect against RF-induced thermal injury by allowing these vessels to act as a heat sink. Substantive heating of vascular endothelium is prevented by heat dissipation in the coronary blood flow (convective cooling), even when the catheter is positioned close to the vessel. Convective cooling likely accounts for the low incidence of coronary artery complications following RF ablation. The protection conferred by convective cooling during RF ablation, however, can be limited by reduction in coronary blood flow, as can be seen in small caliber vessels and in arteries with preexisting stenosis.
Clinical Presentation
Acute occlusion of a coronary artery (by spasm or thrombosis) can manifest as chest discomfort (in nonanesthetized patients) or acute ST-T segment changes on the surface ECG. A high index of suspicion is warranted, since the clinical presentation of acute coronary injury (e.g., chest discomfort and elevation in cardiac troponin levels) can easily be misdiagnosed as pericardial irritation or myocardial injury secondary to RF ablation. Monitoring surface ECG leads for ST changes during an ablation at high-risk sites is imperative to detect acute coronary injury. Also, obtaining a 12-lead ECG immediately after ablation is a critical step that can diagnose ST changes while still treatable and possibly prevent dramatic complications.
Importantly, RF ablation frequently (in 25% to 100% of cases) causes significant elevations in cardiac troponin levels that are not related to coronary arterial injury, with mean peak levels of 0.13 to 6 ng/mL for troponin I, and 0.20 to 2.41 ng/mL for troponin T. In contrast to acute coronary syndromes, troponin elevations observed following RF ablation typically peak early (2 to 8 hours vs. 18 to 24 hours) and normalize early. The levels of troponin elevation correlate with the number of RF lesions applied, the site of lesions (ventricular more than atrial more than annular), and the approach to the left side (transaortic more than transseptal). Ablation of focal lesions (atrioventricular nodal reentrant tachycardia [AVNRT], atrioventricular reentrant tachycardia) causes less frequent (in 25% to 88% of cases) elevation of troponin levels as compared with linear lesion ablation (atrial flutter, AF, and VT), where all patients are expected to have an increase in troponin levels. The prognostic significance of asymptomatic elevations of troponin I remains unclear.
Prevention
Several precautions are important to avoid injury to coronary arteries during percutaneous epicardial ablation, ablation via the coronary venous system, and ablation in the aortic sinuses of Valsalva. Prior to ablation, direct visualization of the relation between the ablation site and adjacent coronary arteries must be obtained, usually by coronary angiography (or ICE imaging) performed with the ablation catheter positioned at the target site. Although an absolute safe distance between the ablation site and epicardial artery has not been defined, a distance of at least 5 mm between the ablation catheter and an epicardial artery is commonly accepted. It is also important to ensure that the catheter is not touching the vessel at any point of the cardiac cycle during angiography.
When ablation in the aortic sinus of Valsalva is planned, aortic root angiography may be considered to visualize the aortic root and the ostia of the right and left coronary arteries. Also, when selective angiography of the coronary arteries is performed, a 5 Fr coronary angiography catheter may be left at the ostium of the coronary artery to serve as a marker and for protection of the artery in case of ablation catheter dislodgment during RF application. Alternatively, a combination of electroanatomic mapping and ICE imaging can be used to confirm anatomic location, catheter tip position and contact, and distance to coronary vasculature, and to monitor RF delivery.
In addition, RF energy output should be minimized during ablation in close proximity to coronary arteries, especially in the presence of small-caliber or diseased vessels. RF application should also be stopped as soon as it is evident that the ablation lesion is not successful in achieving the desired EP effect. Furthermore, ablation is often performed during continuous fluoroscopy to observe for catheter dislodgment, and energy delivery should be discontinued when even minimal dislodgment from the site showing the best mapping findings. Coronary angiography is often performed immediately after the ablation procedure to exclude coronary artery spasm, dissection, or thrombus.
Of note, experimental studies suggest a potential benefit of intracoronary irrigation with chilled saline for coronary protection from heat-induced damage during epicardial RF ablation. The safety and efficacy of this technique in clinical practice, however, have not been evaluated. Also, cryoablation appears to have less risk of coronary injury in animal models, but can still create occlusion and intimal damage when in close proximity, particularly to small vessels.
Iatrogenic Cardiac Arrhythmias
Atrioventricular Block
AV block is the most important complication of AVNRT ablation, occurring in about 0.2% to 0.8% of slow pathway ablation. AV block generally occurs during RF delivery or within the first 24 hours postablation, and is almost always preceded by junctional ectopy with VA block. The level of block is usually in the atrioventricular node (AVN). Predictors of AV block include proximity of the anatomical ablation site to the compact AVN, occurrence of fast junctional tachycardia (cycle length [CL] <350 milliseconds) during RF application, occurrence of junctional rhythm with VA block, the number of RF applications (related to the amount of tissue damage), and significant worsening of anterograde AV conduction during the ablation procedure. The anterior approach for AVNRT ablation is associated with a higher risk of inadvertent complete AV block (approximately 10%, but ranging from 2% to 20%). During ablation of AVNRT, careful monitoring of AV conduction during RF application and prompt discontinuation of energy delivery on evidence of AV block are the keys to minimize the incidence of AV block. RF delivery should be immediately discontinued when the following occur: (1) the impedance rises suddenly (>10 Ω); (2) the PR interval (during NSR or atrial pacing) prolongs; (3) AV block develops; (4) retrograde conduction block is observed during junctional ectopy; or (5) fast junctional tachycardia (tachycardia cycle length <350 milliseconds) occurs, which can herald imminent heart block.
AV block can also occur when ablating along the interventricular septum in the close vicinity of the compact AVN or His bundle (HB), including ablation of superoparaseptal and midseptal AV BTs or ATs, as well as para-Hisian VTs. In addition, because the penetrating HB lies at the membranous septum formed in part by the commissure between the right coronary and noncoronary sinuses of Valsalva, ablation of VTs originating in this region can potentially damage the HB ( Fig. 32.3 ).

When a His potential is detectable at the ablation catheter location, titrated RF energy output and RF application for a short duration should be used, coupled with overdrive atrial pacing to monitor AV conduction in case accelerated junctional rhythm occurs. Alternatively, cryoablation can be used in this region, with its slightly better safety margin for AV conduction.
In some patients, mechanical trauma from the catheter induces temporary AV block. Also, catheter manipulation in the RV or LV can cause mechanical bundle branch block (often transient), which can potentially cause complete AV block in patients with preexisting block in the contralateral bundle branch. Furthermore, ablation of the right bundle branch in patients with bundle branch reentrant VT is commonly followed by complete AV block (in up to 30%) because of preexisting severe disease in the left bundle branch.
Macroreentrant Atrial Tachycardias
Whereas the occurrence of new arrhythmias following focal RF ablation has not been a clinical problem, linear ablation lesions, if incomplete, can promote reentry propagating through the gaps in the ablation lines. Even when conduction block across the ablation lines is verified, the ablation lines commonly performed in the LA for ablation of AF can still facilitate reentry by providing conduction obstacles and protected isthmuses with adjacent anatomical structures in the atrium. In fact, LA macroreentry is a relatively common complication of catheter ablation of AF, occurring in up to 50% of cases. The incidence of ATs seems to be lower following segmental ostial pulmonary vein (PV) isolation (<5%) compared with circumferential PV isolation, and much higher following circumferential or linear LA ablation. Targeting complex fractionated atrial electrograms without linear lesions or PV isolation is associated with a moderate risk for postablation ATs. Following stepwise approaches of catheter ablation incorporating extensive lesions in the LA and RA to terminate persistent AF, ATs can be observed in more than 50% of patients. Although ATs can occur at variable time intervals after AF ablation procedures, the most common timing appears to be 1 to 2 months postablation.
Ventricular Arrhythmias
Malignant ventricular arrhythmias and sudden cardiac death (SCD) have been observed in the early phase following AV junction ablation. Polymorphic VTs are related to electrical instability caused by an initial prolongation and then slow adaptation of repolarization caused by changes in the heart rate and activation sequence. Most polymorphic VTs, ventricular fibrillation, and torsades de pointes that have been reported seem to be consistent with a pause or bradycardia-dependent mechanism. To reduce the risk of these arrhythmias, routine pacing at 80 beats/min has been recommended following AV junction ablation for some period of time. Patients with high-risk factors for arrhythmias, such as congestive heart failure or impaired LV function, may require pacing at higher rates (e.g., at 90 beats/min for 1 to 3 months), as well as in-hospital monitoring for at least 48 hours. Adjustment of the pacing rate, although rarely to less than 70 beats/min, is usually undertaken after 1 week in most patients, preferably after an ECG evaluation for repolarization abnormalities at the lower rate.
Abnormal Sinus Node Function
Inappropriate sinus tachycardia can occur in some patients after posteroseptal BT or AVN ablation, suggesting disruption of the parasympathetic input and/or sympathetic input into the sinus node and AVN. This typically resolves within 3 months following ablation. Some patients have severe sinus bradycardia or arrest after AF ablation; this may be due to sinus node remodeling after long periods of AF (months), but has also been observed in patients with normal sinus node function prior to ablation. In these cases, damage to the sinoatrial node artery can occur when it originates from the circumflex artery (and courses over the LA roof), and an LA roof ablation line interrupts the sinus node artery. This often resolves but may take over a week, and some patients have had permanent pacemaker implantation.
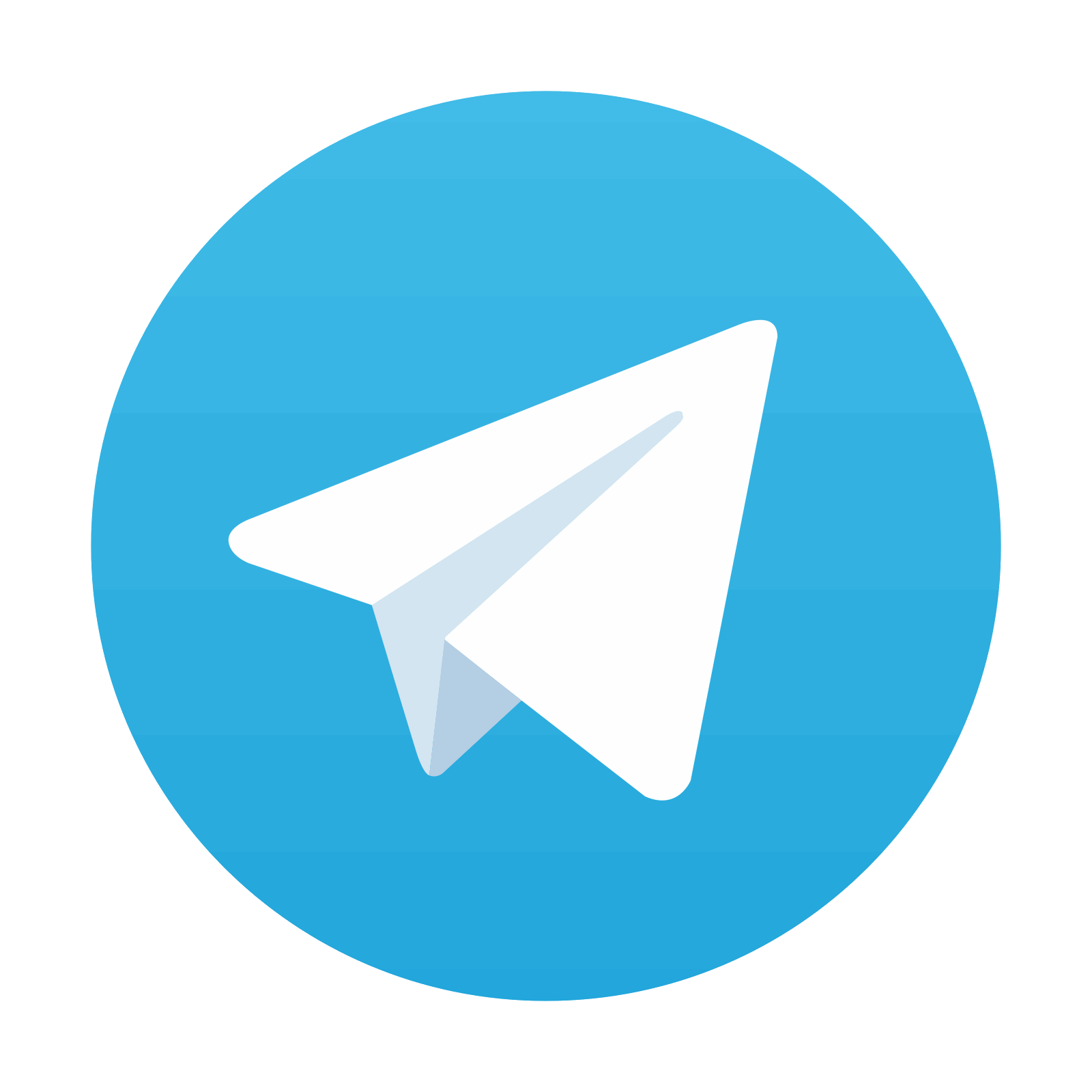
Stay updated, free articles. Join our Telegram channel

Full access? Get Clinical Tree
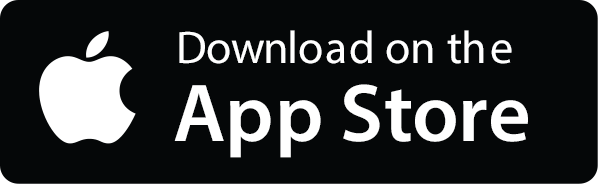
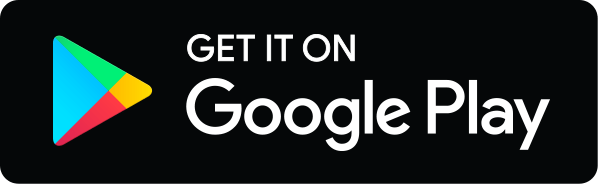